We manufacture the deep drawing press machine. Discount Price from the Manufacturer. Get more information about deep drawing defects.
Common Deep Drawing Defects
The three major common defects which occur during DD are fracture, wrinkling, and tearing. A fracture occurs when the sheet metal is subjected to strains exceeding the safe strain limits of the material. For ductile sheets, this fracture usually occurs near the punch corner. It is because the maximum forming load appears in the material in this region and also stress concentration lines are converging in this section. Once this necking exceeds a certain value, a fracture appears in the drawn cup.
Wrinkling occurs in the flange when compressive stresses in the circumferential direction reach a critical point of instability.
It can occur in regions where the workpiece is unsupported or when the blank holding force is insufficient.
Deep drawing of anisotropic sheets results in a drawn cup with an uneven top edge i.e. some kind of ears are formed at the top as shown in Fig.
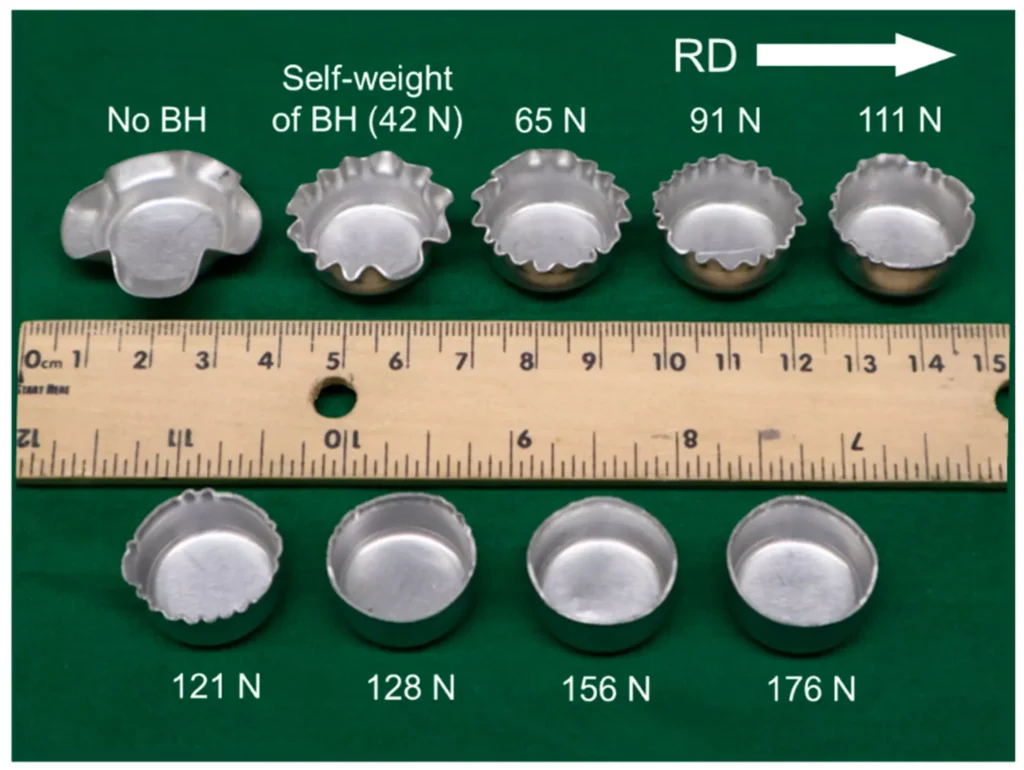
Conventional Deep Drawing
In the conventional deep drawing (CDD) process, the sheet metal is formed into a cup-shaped component. In this process, a flat circular
sheet of metal called blank is placed over the opening in the die and then pushed through and deformed by a moving punch. As the punch
moves downward, the outer annulus of the blank(flange) moves radially inward. The tendency of the flange to fold upward(wrinkling) is restricted by the blank holder force. In CDD, the majority of the deformation occurs in the flange of the cup. The metal is subjected to three different types of stress systems.
Problems encountered in conventional deep drawing
Some of the problems encountered during the conventional deep drawing operation are:
- Difficulty in finding the metal flow precisely.
- Difficulty in setting the criteria for the stability of the metal forming process.
- The complexity involved in the selection of precise process parameters and proper press tool design parameters.
- Large deformation during a deep drawing at room temperature causes problems in forming high-strength, low-formability materials like magnesium, aluminum alloys etc
- Deformation-induced transformation of microstructure as in the case of austenitic stainless steels.
- Number of tools required to produce the desired defect-free component
Limitations of Conventional Deep
Drawing
The main limitations of conventional deep drawing are:
A very high limiting draw ratio cannot be obtained due to excessive thinning and fracture in the cup wall, associated with large draw ratios. For most of the common sheet materials, an LDR of more than 2.2-2.3 is extremely difficult to achieve.
These stresses have an influence on the thickness variation in the drawn cup. The primary deformation occurs in the flange of the deforming which undergoes radial tension and circumferential compression. Because of this, the thickness increases in the flange portion. The second deformation zone is the bending around the die radius while the third deformation zone is the uni-axial stretching (plane strain) in the cup wall, which causes thinning of the metal. Very sharp corners of the punch and the dying lead to fracture. High friction between the die and the sheet puts a limit on the LDR and increases the punch force required for drawing
The surface finish of the component obtained usually is of low quality due to the friction between the sheet and the punch and between the die and the sheet Beyond a certain extent enhancement in formability is not possible because of the inherent limitations of conventional sheet-forming processes. But it was shown that higher forming limits than in conventional forming processes would be possible with the modification of the process itself.
Non-Conventional Deep Drawing
Non-conventional deep drawing is one in which other than the usual mechanical punch like hydraulic, pneumatic, gas, etc., is used to provide the required force or some additional means are used along with the conventional method to improve the forming characteristics of a
material. Some of the nonconventional deep drawing operations are hydro-forming (HF), hydro-mechanical forming (HMDD), warm deep drawing (WDD), superplastic forming (SPF) electromagnetic forming (EMF), incremental forming (IF), etc.
Advantages of non-conventional deep drawing
Metals can be deep drawn which, otherwise, cannot be made at room temperature due to their poor forming characteristics.
- Possibility of deep drawing high strength low formability metals and alloys.
- Components of the very thin sheets (less than 0.5 mm) can be drawn successfully.
- Lesser forming force to form the component.Reduction in the number of tools required for producing the desired part and hence the reduction in production cost.
- Decrease in the probability of defect formation on the product surface.
Limitations of non-conventional deep drawing
The higher capital cost of the additional equipment in some of the processes like hydroforming, and electromagnetic forming. These processes are justifiable only when the production quantities are larger.
- In some processes like electromagnetic forming, gas forming, and operational safety is the primary concern.
- Considerable operational time, as in superplastic forming, etc., and hence the productivity is lower and the cost of the part is higher.
- Numerous process parameters need to be optimized in order to achieve a defect-free component
Deep Drawing Defects
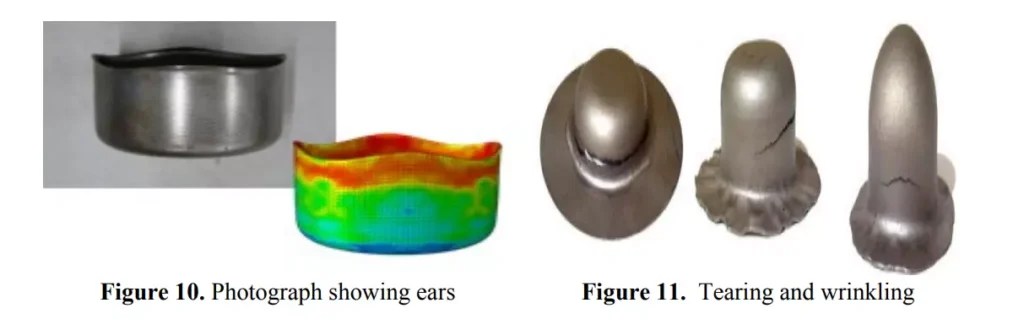
Ironing
Iron consists principally in reducing the wall thickness of the cup by restricting the clearance between the punch and the die to a value less than the blank thickness. The punch load is of primary importance in ironing because it determines the tension in the cup walls and hence the maximum reduction possible for a given punch load. A theoretical study of ironing has been reported in reference and an experimental investigation was carried out using hemispherical head punches for the different conditions of wall thinning, die profile, and lubrication
Galling
It is the transfer of some particles from the cup wall into the part of the die in contact with it. It causes damage to the die surface. It depends on the hardness o the blank and the lubricant’s effectiveness. It can be avoided by keeping the die surface in very good surface quality and using efficient lubricants to reduce friction between the cup and the die surface in contact with it.
Orange Peeling
It occurs at the outer surface of the cup when the grain size of its material is large it can be avoided by reducing the grain size prior to drawing either by heat treatment or by adding grain refining the grains with the addition of the appropriate refiners.
Earing
Earing is one of the defects which is commonly observed in the deep drawing process. By definition, earing is the uneven height at the edge of a drawn product, forming a series of peaks and valleys along its circumference. Kishor and Kumar (2002) defined earing as the formation of waviness on the top of the drawn cup.
The number of ears formed is commonly four (Hosford and Caddell, 2007), but might also be two, six, or eight, depending on the thermo-mechanical processing and microstructure of the sheet. During deep drawing, the sheet metal is subjected to a different amount of plastic strain for each angle relative to the rolling direction, which causes a different amount of elongation resulting in the formation or ears. The difference in the number of elongations results in the formation of ears.
The difference in the amount of plastic deformation at different angles is due to the anisotropic properties of the material. Earing in the deep drawing is usually not desirable as the ears serve no purpose and will have to be cut off, resulting in loss of material, production rate, and increase in production costs
It is caused by planar anisotropy which is due to variations in the mechanical behavior of the sheet from its plane to any other direction inclined or perpendicular to it they are normally even in number 2
or 4 or 6. The worst number is 8 in the case of the brass blank. They appear on the upper part of the drawn cup and are treated by trimming. The photograph in Figure 10 clearly shows the ears on the steel specimen.
Tearing
It might take place at the inner region of the annular part of the rim near the die profile if the holding down pressure is high which stops the blank from sliding and bending over the die profile radius, or it might take place in the maximum thinning region in the clearance region near the punch profile which is subjected to bending and stretching as clearly indicated in Figure 11.
Wrinkling
Wrinkling is one of the major defects that occur in sheet metal forming by the conventional deep drawing process. Wrinkling may be a serious obstacle to a successful forming process and to the assembly of parts, and may also play a significant role in the wear of tools. In order to improve productivity and the quality of products, wrinkling must be avoided. Wrinkling is a kind of buckling phenomenon that prevents from forming of the sheet.
If the buckling takes place in a flange area it is well known as well as it is called puckering if takes place on the wall of the cup. The schematic diagram in Figure 2.12 shows the mechanism of wrinkling initiation and growth in the cylindrical cup deep drawing process and Figure 2.13 shows an example of wrinkling after the deep drawing test.
During the deep drawing process, the sheet under the blank holder is drawn into the deformation zone by the punch. As a result, compressive hoop stress and thus wrinkling can be developed in the sheet metal under the holder (flange wrinkling) as well as those in the side wall, as wrinkling is a phenomenon of compressive instability.
The magnitude of the compressive stress necessary to initiate the side-wall wrinkling is usually smaller than that for the flange wrinkling since the wall is relatively unsupported. Hence, the formation of side-wall wrinkles is relatively easier especially when the ratio of the unsupported dimension to sheet thickness is large.
There are several factors that lead to the wrinkling formation like the retaining force of the blank, the geometrical parameters of the die, the frictions that appear during deep-drawing between the blank and the work elements of the die, the material characteristics and anisotropy, the contact conditions, the part geometry, the mechanical properties of the material, the imperfections in the structure and the initial state of internal tensions of the material, etc.
The wrinkling which occurs in the deep drawing process can be divided into two types that is corrugation which is flange instability and bending over that is the instability in the body of the piece. The phenomenon of wrinkling is specific to the process of deep drawing and also depends on the position in the piece in which it occurs.
Usually, the retaining force has to increase along with the increase of the deep drawing depth but it has to take note that if its value is too big it can lead to cracks and even a break of the material. The main geometric parameter of the die which influences the wrinkling is the diameter of the punch. In the case of friction between the piece and the tool, the increase of the coefficient of friction determines the wrinkling to reduce but the high value of the coefficient can cause cracks and material breakage.
This defect starts in the rim region of the blank and travels towards the middle region, the clearance zone between the punch and die profiles, by the continuation of the deep drawing process, as indicated in Figure 11. Examination of the available literature reveals that experimental research work on this important defect is relatively little compared to the analytical and theoretical work.
The critical dimensions of the rim at the onset of wrinkling were given in reference where it was stated that wrinkling depends on the properties of the blank thickness and its mechanical properties in addition to the shape and dimensions of the die set and the method by which the holding down pressure is applied. The experimental work of reference revealed that the resistance to wrinkling increases with the increase of strain percentage; which explains the use of the draw-beads in the deep drawing process to reduce the liability to wrinkling.
The authors of reference found the critical values of stress and punch displacement at which wrinkling starts to occur. They also agree with the authors of reference that both the critical stress and displacement increase with the increase of strain in the process.
Hydraulic presses are powerful machines used to apply a significant amount of force to an object through hydraulic fluid pressure. They are essential in various industrial applications, providing the necessary force for processes such as metal forming, stamping, bending, and molding. The versatility and efficiency of hydraulic presses make them indispensable tools in manufacturing and production lines. This document will explore the different types of hydraulic presses, their application areas, components, operational principles, manufacturing process, and the challenges and advancements in the industry.
Types of Hydraulic Presses
Hydraulic presses come in various designs, each suited to specific applications and requirements. The primary types of hydraulic presses include C-frame presses, H-frame presses, four-column presses, straightening presses, arbor presses, laminating presses, and transfer presses.
C-frame presses, also known as gap-frame presses, have a C-shaped frame that provides three-sided access to the work area. This design is ideal for applications requiring easy loading and unloading of materials.
H-frame presses, or two-post presses, have a robust H-shaped frame that offers excellent stability and strength. They are commonly used for heavy-duty tasks such as metal forming and straightening.
Four-column presses, or four-post presses, have four vertical columns that provide superior support and uniform force distribution. These presses are suitable for large-scale applications requiring high precision and repeatability.
Straightening presses are specialized hydraulic presses used to straighten bent or distorted metal components. They are widely used in the automotive and construction industries.
Arbor presses are smaller, manually operated hydraulic presses used for light-duty tasks such as assembly, riveting, and broaching. They are commonly found in workshops and small manufacturing facilities.
Laminating presses are used to bond multiple layers of material together under heat and pressure. These presses are essential in industries such as electronics, where laminated components are common.
Transfer presses are automated hydraulic presses that move the workpiece through multiple stations for different operations. They are highly efficient and used in high-volume production environments.
Application Areas
Hydraulic presses are employed in various industries, thanks to their ability to deliver consistent and precise force. Key application areas include:
Metal forming and forging: Hydraulic presses are crucial in shaping and forming metal parts through processes such as stamping, bending, and deep drawing. They are essential in the production of automotive parts, machinery components, and structural elements.
Automotive industry: In the automotive sector, hydraulic presses are used for manufacturing various parts, including body panels, chassis components, and engine parts. They play a critical role in ensuring the structural integrity and performance of vehicles.
Aerospace industry: The aerospace industry relies on hydraulic presses for forming and shaping high-strength materials used in aircraft components. Precision and reliability are paramount in this industry, making hydraulic presses indispensable.
Plastic and rubber molding: Hydraulic presses are used in the molding of plastic and rubber components, including automotive parts, household goods, and medical devices. They ensure consistent product quality and precision.
Electrical and electronics industry: In the electronics sector, hydraulic presses are used for laminating circuit boards, forming connectors, and assembling electronic components. They provide the necessary force and precision for delicate operations.
Medical device manufacturing: Hydraulic presses are used in the production of medical devices, including surgical instruments, implants, and diagnostic equipment. They ensure the high precision and quality required in the medical field.
Packaging industry: Hydraulic presses are employed in the packaging industry for forming and shaping packaging materials, such as cardboard, plastic, and metal. They help produce packaging solutions that are strong, durable, and aesthetically pleasing.
Components of a Hydraulic Press
A hydraulic press comprises several key components that work together to generate and control the applied force. The main components include the frame, hydraulic cylinder, hydraulic pump, control valves, hydraulic fluid, pressure gauges and sensors, and die and tooling.
The frame is the main structure of the hydraulic press, providing stability and support for all other components. It is typically made of high-strength steel to withstand the significant forces generated during operation.
The hydraulic cylinder is the core component that generates the pressing force. It consists of a cylindrical chamber, a piston, and a piston rod. When hydraulic fluid is pumped into the cylinder, it moves the piston, which in turn applies force to the workpiece.
The hydraulic pump is responsible for generating the hydraulic fluid pressure needed to move the piston. It draws hydraulic fluid from a reservoir and delivers it to the cylinder under high pressure.
Control valves regulate the flow of hydraulic fluid to and from the cylinder, controlling the movement and force of the press. These valves can be manually operated or automated, depending on the press design.
Hydraulic fluid, typically oil, is the medium through which force is transmitted in the hydraulic system. It must have suitable properties, such as viscosity and lubricity, to ensure efficient operation and protect system components.
Pressure gauges and sensors monitor the hydraulic fluid pressure within the system. They provide real-time feedback to the operator or control system, ensuring safe and accurate press operation.
Die and tooling are the interchangeable components that come into direct contact with the workpiece. They are designed to shape, form, or cut the material as required by the specific application.
How Hydraulic Presses Work
Hydraulic presses operate based on Pascal’s principle, which states that pressure applied to a confined fluid is transmitted equally in all directions. This principle allows hydraulic presses to generate significant force with relatively small input pressure.
The operation of a hydraulic press begins with the hydraulic pump drawing fluid from the reservoir and delivering it to the cylinder. The control valves regulate the flow of fluid, directing it into the cylinder to move the piston. As the piston moves, it applies force to the workpiece placed between the die and tooling.
The hydraulic fluid plays a crucial role in this process, as it transmits the applied pressure and lubricates the system components. The pressure gauges and sensors continuously monitor the fluid pressure, providing feedback to ensure the press operates within safe limits.
The force generated by the hydraulic press can be precisely controlled by adjusting the hydraulic fluid pressure and the position of the control valves. This allows for accurate and repeatable operations, essential for high-quality manufacturing.
Manufacturing of Hydraulic Presses
The manufacturing of hydraulic presses involves several stages, from design and engineering to assembly and quality control. Each stage is critical to ensuring the press’s performance, reliability, and safety.
Design and engineering: The process begins with the design and engineering phase, where specifications for the press are developed based on the intended application. This includes selecting suitable materials, determining the required force and stroke, and designing the frame and hydraulic system.
Material selection: High-quality materials, such as high-strength steel for the frame and durable alloys for the hydraulic components, are selected to ensure the press’s longevity and performance.
Fabrication of components: The individual components of the hydraulic press, including the frame, cylinder, and pump, are fabricated using precision machining and manufacturing techniques. This ensures that each component meets the required tolerances and specifications.
Assembly process: The fabricated components are then assembled into the complete hydraulic press. This involves mounting the cylinder, pump, and control valves onto the frame, connecting the hydraulic lines, and installing the die and tooling.
Quality control and testing: Rigorous quality control measures are implemented throughout the manufacturing process to ensure the press meets all specifications and standards. This includes pressure testing the hydraulic system, verifying the accuracy of the control valves, and performing operational tests to ensure the press functions correctly.
Advancements and Innovations
The hydraulic press industry is continually evolving, driven by advancements in technology and increasing demands for efficiency and precision. Key innovations include automation and control systems, energy efficiency improvements, and smart hydraulic presses.
Automation and control systems: Modern hydraulic presses are often equipped with advanced control systems that automate the pressing process. This includes programmable logic controllers (PLCs), human-machine interfaces (HMIs), and sensors that monitor and adjust the press’s operation in real time. Automation improves efficiency, reduces the risk of human error, and enhances the consistency of the finished products.
Energy efficiency improvements: Manufacturers are focusing on developing hydraulic presses that consume less energy and have a smaller environmental footprint. This includes using variable displacement pumps, energy recovery systems, and optimizing the hydraulic system’s design to minimize energy losses.
Smart hydraulic presses: The integration of IoT (Internet of Things) technology into hydraulic presses has led to the development of smart presses. These presses can communicate with other machines and systems, providing real-time data on their status, performance, and maintenance needs. This connectivity allows for predictive maintenance, reducing downtime and extending the press’s lifespan.
Challenges in Hydraulic Press Manufacturing
The manufacturing of hydraulic presses presents several challenges, including precision and quality requirements, cost management, technological advancements, and environmental considerations.
Precision and quality requirements: Hydraulic presses must deliver consistent and precise force, which requires high levels of accuracy in the manufacturing process. Ensuring each component meets the required tolerances and specifications is critical to the press’s performance and reliability.
Cost management: The cost of materials, labor, and energy can significantly impact the overall cost of manufacturing hydraulic presses. Manufacturers must balance quality and cost to remain competitive in the market.
Technological advancements: Keeping up with technological advancements is essential for manufacturers to meet the evolving demands of the industry. This requires continuous investment in research and development to incorporate new technologies and improve existing designs.
Environmental considerations: Environmental regulations and sustainability concerns are increasingly important in hydraulic press manufacturing. Manufacturers must develop eco-friendly presses that consume less energy, use recyclable materials, and minimize their environmental impact.
Conclusion
Hydraulic presses are essential machines in various industries, providing the necessary force for processes such as metal forming, stamping, and molding. Understanding the different types of hydraulic presses, their components, and how they work is crucial for effective application and operation.
The manufacturing process of hydraulic presses involves careful design and engineering, material selection, precision fabrication, and rigorous quality control. Despite the challenges, advancements in technology and innovations are driving the industry forward, leading to more efficient, precise, and environmentally friendly hydraulic presses.
As industries continue to evolve, the hydraulic press industry must adapt and innovate to meet the demands of efficiency, precision, and sustainability. Through continuous research and development, manufacturers can enhance the performance and reliability of hydraulic presses, contributing to the success of various industrial applications.