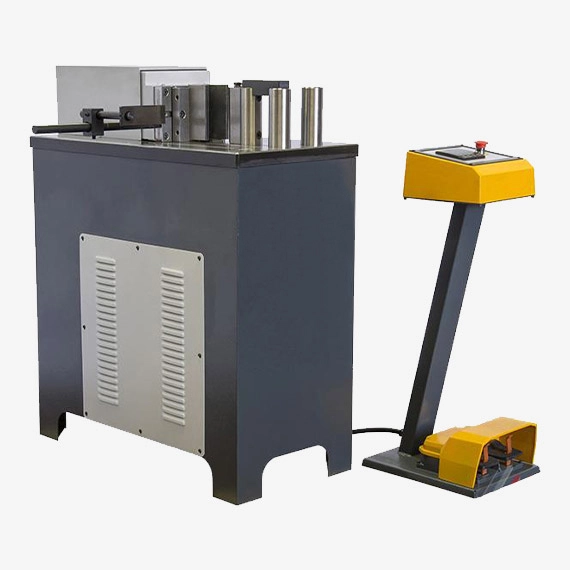
We manufacture a 20 Ton Hydraulic Press for Sale to bend sheet metal edges. 20 Ton Hydraulic Press for Sale are used in metalworking industries
A 20-ton hydraulic press is a powerful and versatile tool commonly used in metal fabrication and other industrial applications. It utilizes hydraulic pressure to apply immense force to a ram, which in turn forces a punch down onto a workpiece, pressing it into a desired shape or form. 20-ton hydraulic presses are suitable for a wide range of applications, including:
- Bending Sheet Metal: These machines are widely used to bend sheet metal components for various products, such as enclosures, brackets, ductwork, structural components, and various other sheet metal parts.
- Forming Extrusions: 20-ton hydraulic presses can form extrusions, which are shapes created by forcing metal through a die. This process is used to create various components, such as tubes, channels, and beams.
- Stamping and Punching: These presses can be used for stamping and punching operations, where a punch is used to cut or form shapes in sheet metal. This process is used to create components like washers, gaskets, and electrical connectors.
- Prototyping Sheet Metal Designs: These machines are employed to create prototypes of sheet metal components to evaluate form, fit, and functionality before full-scale production.
- High-Volume Production: 20-ton hydraulic presses are designed for high-volume production, enabling efficient manufacturing of large quantities of sheet metal components.
Key Components of a 20-Ton Hydraulic Press
A 20-ton hydraulic press consists of several essential components that work together to achieve precise and efficient pressing operations:
- Frame: The sturdy frame provides a solid base for the machine and supports the pressing mechanism. It is typically constructed from heavy-duty steel or cast iron to withstand the immense forces involved in pressing.
- Hydraulic System: The heart of the machine, the hydraulic system generates and supplies pressurized hydraulic fluid to the pressing mechanism. It consists of a hydraulic pump, hoses, valves, and a hydraulic cylinder. The hydraulic cylinder applies high-pressure hydraulic fluid to the ram, providing the force required to press the workpiece.
- Ram: The movable ram applies downward force onto the workpiece. Connected to the hydraulic cylinder and guided by rails, it ensures precise movement and alignment during the pressing process.
- Punch: The punch is a tooling component that forms the desired shape or feature in the workpiece. Typically made from hardened steel or tool steel to withstand abrasion and pressing forces, its profile corresponds to the desired shape.
- Die: The die, another tooling component, supports the workpiece during pressing. Designed to match the punch’s shape, it creates the desired shape or feature and prevents distortion. The die provides a stable surface against which the punch forces the workpiece to deform.
- Work Table: The adjustable work table provides a stable surface for positioning and securing the workpiece during pressing. It accommodates different workpiece sizes and orientations, ensuring precise positioning.
- Back Gauge: The back gauge, also known as the depth stop, controls the distance between the rear edge of the workpiece and the pressing line. It ensures consistent pressing positions and prevents the workpiece from slipping during the pressing process, maintaining accuracy and consistency.
- Controls: The control panel allows the operator to set and monitor the pressing process. Modern hydraulic presses often feature advanced computer controls for precise and automated operation. These controls enable operators to set pressing forces, pressing speeds, and other parameters, ensuring consistent and repeatable results.
Safety Precautions for Operating 20-Ton Hydraulic Presses
When operating 20-ton hydraulic presses, it is crucial to adhere to strict safety protocols to prevent injuries and accidents:
- Thorough Training: Operators must receive comprehensive training on the specific machine model, its operation, and safety procedures.
- Personal Protective Equipment (PPE): Always wear appropriate PPE, including safety glasses, gloves, and hearing protection, to protect from flying debris, sharp edges, and noise.
- Secure the Workpiece: Ensure the workpiece is securely positioned and clamped to the work table to prevent movement during pressing operations.
- Clear Work Area: Keep the work area free of obstructions and potential hazards. Secure any loose objects or hanging wires.
- Maintain Proper Form: Maintain proper stance and posture while operating the press to avoid strain or injury.
- Emergency Stop Procedures: Familiarize yourself with the emergency stop procedures and be prepared to activate them in case of any unexpected malfunctions or hazards.
- Regular Maintenance: Follow the manufacturer’s recommended maintenance schedule to ensure the machine and tooling are functioning correctly and safely.
By following these safety precautions and adhering to proper operating procedures, individuals can safely operate 20-ton hydraulic presses and produce high-quality components for various applications.
20 Ton Hydraulic Press for Sale
A horizontal hydraulic press is a powerful and versatile machine used for a wide range of metal fabrication tasks, including bending, straightening, and forming sheet metal components. It utilizes hydraulic pressure to apply force to a movable ram, which in turn forces a punch down onto a workpiece, pressing it into the desired shape or form. Unlike vertical hydraulic presses, horizontal presses operate with the ram and punch moving horizontally, offering unique advantages for specific applications.
Key Components of a Horizontal Hydraulic Press
- Frame: The sturdy frame provides a solid base for the machine and supports the pressing mechanism. It is typically constructed from heavy-duty steel or cast iron to withstand the immense forces involved in pressing.
- Hydraulic System: The heart of the machine, the hydraulic system generates and supplies pressurized hydraulic fluid to the pressing mechanism. It consists of a hydraulic pump, hoses, valves, and a hydraulic cylinder. The hydraulic cylinder applies high-pressure hydraulic fluid to the ram, providing the force required to press the workpiece.
- Ram: The movable ram applies force onto the workpiece, moving horizontally along the press bed. Connected to the hydraulic cylinder and guided by rails, it ensures precise movement and alignment during the pressing process.
- Punch: The punch is a tooling component that forms the desired shape or feature in the workpiece. Typically made from hardened steel or tool steel to withstand abrasion and pressing forces, its profile corresponds to the desired shape.
- Die: The die, another tooling component, supports the workpiece during pressing. Designed to match the punch’s shape, it creates the desired shape or feature and prevents distortion. The die provides a stable surface against
Fluid Power
Fluid power is a broad engineering discipline encompassing the generation, control, and transmission of power using pressurized fluids, typically either liquids (hydraulic systems) or gases (pneumatic systems). The fundamental principle underlying fluid power systems is Pascal’s Law, which states that pressure exerted on a confined fluid is transmitted undiminished in all directions. This principle forms the basis for the operation of hydraulic and pneumatic systems.
In hydraulic systems, hydraulic fluid (usually oil) is pressurized by a hydraulic pump and then directed through control valves to act upon hydraulic cylinders or motors, generating linear or rotary motion, respectively. Hydraulic systems are known for their high power density, precise control, and ability to generate large forces, making them suitable for a wide range of applications including construction equipment, manufacturing machinery, and aerospace systems.
Pneumatic systems, on the other hand, use compressed air as the working fluid. Compressed air is generated by pneumatic compressors and stored in reservoirs before being directed through control valves to pneumatic actuators, such as cylinders or motors. Pneumatic systems are valued for their simplicity, cleanliness, and relatively low cost, though they typically offer lower power density compared to hydraulic systems.
Both hydraulic and pneumatic systems utilize a variety of components including pumps, valves, actuators, and control systems to perform specific tasks. These systems are commonly employed in industrial automation, manufacturing processes, automotive systems, and mobile machinery due to their versatility, efficiency, and reliability.
Fluid power engineers are responsible for designing, implementing, and maintaining fluid power systems to meet specific performance requirements. This involves considerations such as fluid selection, system layout, component sizing, and control strategies. Additionally, fluid power engineers must address challenges related to system efficiency, safety, and reliability, often employing advanced technologies such as computational fluid dynamics (CFD) and simulation tools to optimize system performance and minimize energy consumption.
In summary, fluid power plays a critical role in modern engineering by providing efficient and flexible means of transmitting power for a diverse range of applications. Understanding the principles and applications of fluid power is essential for engineers working in fields such as mechanical, aerospace, and industrial engineering.
Hydraulic Systems
Hydraulic systems are a cornerstone of modern engineering, utilizing the principles of fluid mechanics to transmit power through the controlled movement of hydraulic fluids. These systems are widely employed across various industries due to their high power density, precise control, and ability to generate substantial forces.
At the heart of a hydraulic system is the hydraulic pump, which pressurizes the hydraulic fluid, typically oil, to a level necessary for the intended application. The pressurized fluid is then directed through a network of hydraulic lines and control valves to hydraulic actuators, such as cylinders or motors, where it exerts force to perform mechanical work.
Hydraulic cylinders are commonly used for linear motion applications, such as lifting heavy loads or actuating machinery components. These cylinders consist of a cylindrical barrel, a piston, and hydraulic fluid. When pressure is applied to one side of the piston, it moves linearly, exerting force in the process. Hydraulic motors, on the other hand, convert hydraulic energy into rotary motion, making them suitable for applications such as driving conveyor belts or rotating equipment.
Control valves play a crucial role in regulating the flow and direction of hydraulic fluid within the system. Directional control valves determine the path of fluid flow, while pressure control valves regulate the pressure levels to ensure safe and efficient operation. Proportional valves offer finer control by modulating the flow or pressure based on input signals, enabling precise positioning and velocity control.
Hydraulic systems find extensive use in various industries, including construction, manufacturing, agriculture, and aerospace. Excavators, bulldozers, and cranes in the construction industry rely on hydraulic systems for their lifting and digging operations. Manufacturing machinery, such as presses and injection molding machines, utilize hydraulic power for precise control over force and speed. Aircraft and spacecraft employ hydraulic systems for functions like landing gear deployment and flight control surfaces’ actuation.
Maintaining hydraulic systems is essential to ensure their continued reliability and performance. Regular inspection of components, monitoring fluid quality, and addressing leaks are vital aspects of hydraulic system maintenance. Additionally, proper fluid filtration and temperature control help prolong the lifespan of system components and prevent damage due to contamination or overheating.
In conclusion, hydraulic systems represent a versatile and efficient means of power transmission in modern engineering. Their ability to generate high forces, precise control, and adaptability to various applications make them indispensable across a wide range of industries. Understanding the principles and components of hydraulic systems is essential for engineers working in fields such as mechanical, civil, and aerospace engineering.
Pneumatic Systems
Pneumatic systems are essential components of modern engineering, utilizing compressed air or inert gases to transmit power and control mechanical motion. These systems offer advantages such as simplicity, cleanliness, and relatively low cost, making them ubiquitous in a wide range of applications across industries.
At the core of a pneumatic system is the pneumatic compressor, which compresses ambient air or gases to a desired pressure level. The compressed air is stored in reservoirs or tanks, ready to be used when needed. Unlike hydraulic systems, which use incompressible fluids, pneumatic systems rely on the compressibility of gases to transmit force and motion.
Pneumatic actuators, such as cylinders and motors, are the primary components responsible for converting compressed air energy into mechanical motion. Pneumatic cylinders, also known as air cylinders, consist of a cylindrical barrel with a piston inside. When compressed air is introduced into one side of the cylinder, it pushes the piston, causing linear motion. This linear motion can be used for tasks such as lifting, clamping, or pushing objects.
Similarly, pneumatic motors convert compressed air energy into rotary motion, allowing for the operation of various machinery and equipment. These motors are lightweight, compact, and offer high power-to-weight ratios, making them suitable for applications where space and weight constraints are critical.
Control valves play a crucial role in pneumatic systems, regulating the flow of compressed air and directing it to different actuators as needed. Directional control valves determine the direction of airflow, while pressure control valves maintain the desired pressure levels within the system. Proportional valves offer precise control by modulating airflow based on input signals, enabling fine adjustments to actuator speed and position.
Pneumatic systems find widespread use in industries such as manufacturing, automotive, pharmaceuticals, and packaging. Automated assembly lines rely on pneumatic actuators for tasks such as part positioning, gripping, and sorting. Pneumatic tools, such as drills, impact wrenches, and paint sprayers, utilize compressed air for powering their operation. In the automotive industry, pneumatic systems are employed in brake systems, suspension systems, and tire inflation.
Maintenance of pneumatic systems involves regular inspection of components, ensuring proper lubrication, and addressing leaks or pressure losses. Proper filtration of compressed air is essential to prevent contamination and ensure the longevity of system components. Additionally, safety measures such as pressure relief valves and lockout/tagout procedures are implemented to protect personnel and equipment from potential hazards.
In conclusion, pneumatic systems offer a versatile and cost-effective solution for power transmission and motion control in various engineering applications. Their simplicity, reliability, and ease of operation make them indispensable in industries where rapid and precise motion is required. Understanding the principles and components of pneumatic systems is essential for engineers and technicians working in fields such as manufacturing, automation, and robotics.
Pascal’s Law
Pascal’s Law, named after the French mathematician and physicist Blaise Pascal, is a fundamental principle in fluid mechanics that describes how pressure changes in a fluid are transmitted undiminished throughout the fluid and to the walls of its container. The law is the cornerstone of hydraulic and pneumatic systems, forming the basis for their operation and enabling the transmission of force and motion over distances.
Pascal’s Law states that when pressure is applied to a confined fluid in a closed system, the pressure change is transmitted equally in all directions within the fluid. In other words, if pressure is exerted at one point in the fluid, that pressure is distributed uniformly throughout the fluid and transmitted to all points within the system. Additionally, this pressure is also transmitted to the walls of the container that holds the fluid.
Mathematically, Pascal’s Law can be expressed as P = F/A, where P is the pressure exerted on the fluid, F is the force applied to the fluid, and A is the area over which the force is applied. This relationship illustrates that pressure is directly proportional to force and inversely proportional to the area over which the force is distributed.
In practical terms, Pascal’s Law allows hydraulic and pneumatic systems to generate and transmit large forces and motions using relatively small input forces. For example, in a hydraulic system, a small force applied to a small piston can generate a much larger force on a larger piston connected to the same system, allowing for the amplification of force and the performance of heavy-duty tasks such as lifting heavy loads or bending metal.
Pascal’s Law has numerous applications across various industries, including automotive, aerospace, construction, and manufacturing. Hydraulic systems, such as hydraulic presses, cranes, and excavators, rely on Pascal’s Law to generate the force required for their operation. Similarly, pneumatic systems, including pneumatic tools, actuators, and control systems, utilize Pascal’s Law to transmit compressed air pressure and achieve desired mechanical motion.
Understanding Pascal’s Law is essential for engineers and technicians working with fluid power systems, as it provides the foundational principles necessary for designing, analyzing, and troubleshooting hydraulic and pneumatic systems. By applying Pascal’s Law, engineers can optimize system performance, ensure safety, and achieve desired outcomes in various engineering applications.
Pressure Generation
Pressure generation is a fundamental aspect of fluid mechanics and plays a crucial role in various engineering applications, particularly in hydraulic and pneumatic systems. Pressure is defined as the force exerted per unit area and is measured in units such as Pascals (Pa), pounds per square inch (psi), or bar.
In fluid power systems, pressure is generated by applying a force to a confined fluid within a closed system. This force can be generated through mechanical means, such as by a hydraulic pump or a pneumatic compressor, or by other methods such as gravity or external pressure sources. Regardless of the method used, the key objective is to increase the energy stored in the fluid, resulting in an increase in pressure.
In hydraulic systems, pressure generation typically involves using a hydraulic pump to pressurize hydraulic fluid, usually oil, to a desired level. The pump applies mechanical force to the fluid, causing it to flow and build up pressure within the system. The generated pressure is then utilized to perform mechanical work, such as actuating hydraulic cylinders or motors to produce motion or force.
Similarly, in pneumatic systems, pressure generation is achieved by compressing air or inert gases using a pneumatic compressor. The compressor applies mechanical energy to the gas molecules, reducing their volume and increasing their pressure. The pressurized gas is stored in reservoirs or tanks and can be directed to pneumatic actuators to produce mechanical motion or force as required.
The ability to generate and control pressure is essential for the proper operation of fluid power systems. The level of pressure required depends on the specific application and dictates the type and capacity of the components used within the system. For example, high-pressure hydraulic systems are often used in heavy-duty applications where substantial force is required, such as in construction equipment or metal forming processes. Conversely, low-pressure pneumatic systems are suitable for lighter-duty applications where precise control and rapid motion are essential, such as in robotics or assembly lines.
Maintaining the desired pressure level within a fluid power system is critical for ensuring optimal performance and safety. Pressure regulation is achieved using various control mechanisms, including pressure relief valves, pressure switches, and regulators, which monitor and adjust the pressure as needed to prevent overpressure conditions and system failures.
In summary, pressure generation is a fundamental concept in fluid power engineering, enabling the transmission of force and motion in hydraulic and pneumatic systems. Understanding the principles of pressure generation is essential for engineers and technicians working with fluid power systems, as it forms the basis for designing, operating, and maintaining efficient and reliable hydraulic and pneumatic systems.
Pascal’s Law
Pascal’s Law, named after the French mathematician Blaise Pascal, is a foundational principle in fluid mechanics that describes the behavior of fluids under pressure. This law is fundamental to understanding the operation of hydraulic systems and plays a crucial role in various engineering applications.
The essence of Pascal’s Law lies in the concept of pressure transmission within a confined fluid. It states that when pressure is applied to a fluid in a closed container, that pressure is transmitted undiminished throughout the fluid and acts equally in all directions. In simpler terms, any change in pressure applied to a fluid in an enclosed system will be distributed uniformly throughout the fluid and will be felt by all parts of the container’s walls.
Pascal’s Law has profound implications for hydraulic systems, where it forms the basis for their operation. In a hydraulic system, a small force exerted on a small piston can generate a much larger force on a larger piston, provided that both pistons are interconnected and filled with the same fluid. This phenomenon is due to the pressure being transmitted equally throughout the fluid, resulting in a force multiplication effect.
The applications of Pascal’s Law are widespread across numerous industries. Hydraulic systems are commonly found in heavy machinery, such as construction equipment, cranes, and hydraulic presses, where they provide the necessary force for lifting, pushing, or bending heavy loads. Additionally, hydraulic brakes in vehicles utilize Pascal’s Law to transmit force from the brake pedal to the brake calipers, allowing for effective braking action.
Understanding Pascal’s Law is essential for engineers working with fluid power systems, as it provides the theoretical foundation for designing, analyzing, and troubleshooting hydraulic systems. By applying Pascal’s Law, engineers can predict and control the behavior of fluids under pressure, ensuring the efficient and reliable operation of hydraulic systems in various engineering applications.
Force Multiplication in Hydraulic Systems
Force multiplication is a fundamental concept in hydraulic systems that enables the generation of large forces using relatively small input forces. This principle is based on Pascal’s Law, which states that pressure applied to a confined fluid is transmitted undiminished throughout the fluid and to the walls of its container.
In hydraulic systems, force multiplication occurs through the use of interconnected pistons of different sizes within hydraulic cylinders. When a force is applied to a small piston, it generates pressure within the fluid, which is transmitted equally throughout the system. This pressure acts on a larger piston connected to the same fluid, resulting in a proportionally larger force being exerted on the larger piston.
Force multiplication is utilized in various hydraulic applications where high forces are required, such as in construction equipment (e.g., hydraulic excavators, bulldozers), manufacturing machinery (e.g., hydraulic presses, injection molding machines), and aerospace systems (e.g., hydraulic actuators for flight control surfaces).
Understanding force multiplication in hydraulic systems is crucial for engineers designing hydraulic systems, as it enables them to optimize system efficiency, select appropriate components, and ensure the system can meet the required force output for a given application. Additionally, proper maintenance and troubleshooting of hydraulic systems involve consideration of force multiplication principles to diagnose and address issues related to force transmission within the system.
Hydraulic Fluid
Hydraulic fluid is a specialized liquid medium used in hydraulic systems to transmit power and lubricate components. It serves as the primary means of energy transmission within hydraulic systems, providing the necessary force to actuate hydraulic cylinders, motors, and other components. Hydraulic fluids are carefully formulated to meet specific performance requirements and operating conditions, making them integral to the efficient and reliable operation of hydraulic systems.
Several characteristics distinguish hydraulic fluids and determine their suitability for particular applications:
- Viscosity: Hydraulic fluids exhibit a specific viscosity range that influences their flow characteristics and lubricating properties. Proper viscosity ensures efficient power transmission and protects components from wear and frictional damage.
- Viscosity Index: The viscosity index measures the fluid’s viscosity variation with temperature changes. Hydraulic fluids with high viscosity index maintain consistent performance across a wide temperature range, ensuring system reliability in diverse operating conditions.
- Chemical Stability: Hydraulic fluids must resist chemical degradation and oxidation to maintain their performance and prolong service life. Stable fluids prevent the formation of sludge, varnish, and other contaminants that can compromise system efficiency and reliability.
- Corrosion Resistance: Hydraulic fluids should protect system components from corrosion caused by exposure to moisture, oxygen, and other contaminants. Corrosion-resistant fluids prevent damage to critical components, extending system longevity and reliability.
- Foaming Characteristics: Excessive foaming reduces hydraulic system efficiency and can lead to cavitation, component wear, and performance degradation. Hydraulic fluids with low foaming tendencies maintain system integrity and ensure consistent performance under dynamic operating conditions.
- Compatibility: Hydraulic fluids must be compatible with system materials, seals, and components to prevent deterioration and ensure long-term reliability. Incompatible fluids can cause seal swelling, material degradation, and system malfunctions, compromising overall performance.
- Fire Resistance: In applications where fire hazards are present, such as in industrial settings or aircraft hydraulic systems, fire-resistant hydraulic fluids are essential to mitigate the risk of fire and ensure personnel safety.
Common types of hydraulic fluids include mineral oils, synthetic oils, water-based fluids, and biodegradable fluids. Each type offers specific advantages and is selected based on factors such as operating temperature range, environmental considerations, and performance requirements.
Proper maintenance of hydraulic fluid involves regular monitoring of fluid condition, filtration, and periodic replacement to ensure optimal system performance and longevity. Contaminated or degraded fluid can impair system efficiency, cause component wear, and lead to costly downtime and repairs.
In summary, hydraulic fluid is a critical component of hydraulic systems, serving as the primary medium for power transmission and component lubrication. Understanding the characteristics and requirements of hydraulic fluids is essential for selecting the appropriate fluid type and ensuring the reliable operation of hydraulic systems in various industrial applications.
Hydraulic Pump
A hydraulic pump is a mechanical device used to convert mechanical power into hydraulic energy by pressurizing hydraulic fluid. It serves as the heart of a hydraulic system, providing the force necessary to move fluid through the system and create the pressure required for actuating hydraulic cylinders, motors, and other components. Hydraulic pumps are integral to various applications across industries, including construction, manufacturing, agriculture, and aerospace.
There are several types of hydraulic pumps, each with its unique operating principles, advantages, and applications:
- Gear Pumps: Gear pumps utilize meshing gears to trap and pressurize hydraulic fluid, which is then discharged through the outlet port. They are simple in design, cost-effective, and suitable for applications requiring low to moderate pressure and flow rates.
- Vane Pumps: Vane pumps consist of vanes mounted on a rotor that rotates within a housing. As the rotor spins, centrifugal force pushes the vanes outward, trapping and pressurizing fluid between the vanes and the housing. Vane pumps offer higher efficiency and quieter operation compared to gear pumps and are commonly used in medium-pressure applications.
- Piston Pumps: Piston pumps feature reciprocating pistons housed within cylinders, which move back and forth to draw in and pressurize hydraulic fluid. Piston pumps offer high efficiency, precise control, and the ability to generate high pressure and flow rates, making them ideal for demanding applications where performance is critical.
Hydraulic pumps can further be classified based on their operating principles as:
- Fixed Displacement Pumps: These pumps deliver a constant volume of fluid per rotation, regardless of system pressure. Common types include gear pumps and vane pumps.
- Variable Displacement Pumps: Variable displacement pumps allow for the adjustment of output flow rate or pressure based on system requirements. This flexibility is achieved through mechanisms such as swash plates (in axial piston pumps) or tilting cylinders (in bent-axis piston pumps), allowing for precise control over system performance.
Proper selection of a hydraulic pump is essential to ensure optimal system performance and efficiency. Factors such as required flow rate, pressure rating, operating conditions (temperature, environment), and system compatibility must be considered when choosing the appropriate pump for a specific application.
Maintenance of hydraulic pumps involves regular inspection, monitoring of fluid levels and condition, and addressing issues such as leaks, wear, or contamination. Proper maintenance practices help prolong pump life, prevent costly downtime, and ensure the reliable operation of hydraulic systems.
In summary, hydraulic pumps play a vital role in converting mechanical power into hydraulic energy, enabling the operation of hydraulic systems in various industrial applications. Understanding the types, operating principles, and selection criteria of hydraulic pumps is essential for engineers and technicians working with hydraulic systems to achieve optimal performance and reliability.
Hydraulic Valve
Hydraulic valves are essential components in hydraulic systems, serving to control the flow, direction, and pressure of hydraulic fluid within the system. These valves play a crucial role in regulating the operation of hydraulic actuators, such as cylinders and motors, and are integral to achieving precise control and efficient performance in hydraulic systems.
There are several types of hydraulic valves, each designed to perform specific functions within the system:
- Directional Control Valves: Directional control valves, also known as directional valves, regulate the direction of hydraulic fluid flow within the system. They typically have multiple ports and positions, allowing the operator to control the direction of fluid flow and consequently the direction of motion of hydraulic actuators. Directional control valves are available in various configurations, including spool valves, poppet valves, and rotary valves, each suitable for different applications and operating conditions.
- Pressure Control Valves: Pressure control valves regulate the pressure of hydraulic fluid within the system to maintain it within specified limits. These valves protect system components from overpressure conditions, prevent damage due to excessive loads, and ensure safe and efficient operation. Common types of pressure control valves include relief valves, pressure-reducing valves, and sequence valves.
- Flow Control Valves: Flow control valves regulate the rate of fluid flow within the system, allowing for precise control over the speed and movement of hydraulic actuators. These valves are essential for achieving uniform motion, controlling acceleration and deceleration, and optimizing energy efficiency. Flow control valves can be either fixed or adjustable and are available in various configurations, including throttle valves, needle valves, and flow divider valves.
- Check Valves: Check valves, also known as non-return valves, allow fluid to flow in one direction while preventing reverse flow. They ensure that hydraulic actuators maintain their position and prevent unintended movement when pressure is released. Check valves are commonly used in applications where backflow prevention is critical, such as in hydraulic circuits with multiple actuators or in load-holding applications.
- Proportional Valves: Proportional valves provide precise control over fluid flow, pressure, and direction by modulating their output based on input signals. These valves offer continuous and variable control over system parameters, allowing for fine adjustments to be made to achieve desired performance characteristics. Proportional valves are used in applications where accurate control, smooth operation, and dynamic response are required, such as in closed-loop control systems and servo applications.
Hydraulic valves are selected based on factors such as system requirements, operating conditions, flow rates, pressure ratings, and compatibility with other system components. Proper installation, maintenance, and troubleshooting of hydraulic valves are essential to ensure optimal system performance, reliability, and safety.
In summary, hydraulic valves are critical components in hydraulic systems, providing control over fluid flow, direction, and pressure to achieve precise and efficient operation. Understanding the different types of hydraulic valves, their functions, and their applications is essential for engineers and technicians working with hydraulic systems to design, operate, and maintain hydraulic systems effectively.
Hydraulic Control
Hydraulic control is the process of regulating and managing the flow, pressure, and direction of hydraulic fluid within a hydraulic system to achieve desired mechanical outcomes. It involves the use of various control components, such as valves, pumps, actuators, and sensors, to ensure precise and efficient operation of hydraulic machinery and equipment.
Key aspects of hydraulic control include:
- Flow Control: Flow control involves regulating the rate of hydraulic fluid flow within the system to achieve specific performance requirements. This can be accomplished using flow control valves, which adjust the size of the flow passage or restrict the flow rate to control the speed and movement of hydraulic actuators.
- Pressure Control: Pressure control is essential for maintaining hydraulic system integrity and ensuring the safety of system components. Pressure control valves, such as relief valves, pressure-reducing valves, and sequence valves, are used to regulate hydraulic fluid pressure within predetermined limits and protect system components from damage due to excessive pressure.
- Directional Control: Directional control refers to managing the direction of hydraulic fluid flow within the system to control the movement of hydraulic actuators. Directional control valves, including spool valves, poppet valves, and rotary valves, are employed to direct fluid flow to different hydraulic actuators and control the direction of their movement.
- Position Control: Position control involves regulating the position of hydraulic actuators, such as cylinders and motors, to achieve precise movement and positioning of mechanical components. This can be accomplished using proportional valves, servo valves, or closed-loop control systems that monitor actuator position and adjust control inputs accordingly to maintain desired positions.
- Load Control: Load control is the ability to regulate the force exerted by hydraulic actuators to accommodate varying loads or resistances encountered during operation. Load-sensing systems, pressure-compensated control valves, and load-holding valves are commonly used to adjust hydraulic system performance based on load conditions and ensure consistent operation under changing load demands.
- Feedback Control: Feedback control systems use sensors and feedback mechanisms to monitor hydraulic system parameters, such as pressure, flow, temperature, and position, and provide real-time feedback to the control system. This enables automatic adjustment of control inputs to maintain desired system performance and respond dynamically to changing operating conditions.
Hydraulic control systems can vary in complexity depending on the specific application requirements and performance criteria. Advanced control strategies, such as proportional control, servo control, and closed-loop feedback control, are employed in sophisticated hydraulic systems to achieve precise motion control, dynamic response, and energy efficiency.
Effective hydraulic control requires careful system design, component selection, installation, calibration, and maintenance to ensure optimal performance, reliability, and safety. Engineers and technicians responsible for hydraulic systems must possess a thorough understanding of hydraulic control principles and techniques to design, operate, and maintain hydraulic systems effectively in various industrial applications.
Hydraulic Accumulator
A hydraulic accumulator is a device used in hydraulic systems to store and release hydraulic energy in the form of pressurized fluid. It serves as a reservoir for hydraulic fluid under pressure, enabling the system to store energy during periods of low demand and release it rapidly when needed. Hydraulic accumulators play a crucial role in improving system efficiency, stability, and performance, particularly in applications with fluctuating loads or intermittent operation.
Key components and characteristics of hydraulic accumulators include:
- Cylinder: The main body of the accumulator, typically cylindrical in shape, houses the hydraulic fluid and separates it from the gas or fluid used to provide the compressive force.
- Piston or Bladder: Inside the cylinder, a piston or bladder separates the hydraulic fluid from the compressible gas or fluid. As hydraulic fluid enters the accumulator, it compresses the gas or fluid, storing potential energy in the form of pressurized gas or fluid.
- Gas or Fluid Chamber: The space above the piston or bladder contains the compressible gas or fluid, which provides the counterforce to the hydraulic fluid pressure. This chamber allows the accumulator to store energy by compressing the gas or fluid, which expands when the stored energy is released.
- Check Valve: A check valve located at the inlet/outlet port of the accumulator allows hydraulic fluid to enter and exit the accumulator while preventing backflow.
- Gas Valve: In gas-charged accumulators, a gas valve allows for the adjustment of gas pressure to suit specific system requirements.
Hydraulic accumulators offer several benefits in hydraulic systems:
- Energy Storage: Accumulators store hydraulic energy, allowing the system to respond quickly to sudden demands for power or compensate for fluctuations in flow or pressure.
- Shock Absorption: Accumulators absorb pressure spikes and hydraulic shocks, protecting system components from damage and reducing wear and tear.
- Emergency Power Source: In the event of a power failure or pump shutdown, accumulators can provide temporary hydraulic power to critical system functions, such as emergency braking or lifting.
- Peak Power Augmentation: By releasing stored energy rapidly, accumulators can supplement the hydraulic pump’s output during peak demand periods, enhancing system performance and responsiveness.
Hydraulic accumulators are commonly used in various industrial applications, including:
- Mobile Equipment: Such as excavators, cranes, and forklifts, where accumulator-based systems provide responsive and efficient hydraulic power for lifting, braking, and steering.
- Machine Tools: Where accumulators absorb shock and vibration, improving machining precision and extending tool life.
- Pressurization Systems: In hydraulic presses, molding machines, and test rigs, where accumulators maintain constant pressure and compensate for flow fluctuations.
Proper selection, sizing, installation, and maintenance of hydraulic accumulators are essential to ensure optimal performance, reliability, and safety in hydraulic systems. Engineers and technicians working with hydraulic systems must have a thorough understanding of accumulator principles and applications to design, operate, and maintain hydraulic systems effectively in various industrial settings.
Hydraulic Cylinder
A hydraulic cylinder is a mechanical actuator used to produce linear motion and force in hydraulic systems. It converts hydraulic energy into mechanical energy by applying fluid pressure against a piston within a cylindrical housing, generating linear movement along the axis of the cylinder. Hydraulic cylinders are essential components in various industrial applications, including construction equipment, manufacturing machinery, agricultural implements, and aerospace systems.
Key components and characteristics of hydraulic cylinders include:
- Cylinder Barrel: The main body of the hydraulic cylinder, typically made of high-strength steel, houses the piston and provides structural support.
- Piston: A cylindrical or disk-shaped component that divides the cylinder into two chambers: the rod side (or head end) and the cap side (or blind end). The piston is sealed against the cylinder barrel to prevent fluid leakage between the two chambers.
- Rod: A rod extends from the piston through a sealed opening in one end of the cylinder barrel, transmitting the force generated by hydraulic pressure to the external load. The rod is typically made of hardened steel and is subject to bending and torsional forces during operation.
- Seals: O-ring or lip seals are used to prevent fluid leakage between the piston and cylinder barrel and around the rod. Proper seal selection and maintenance are critical to ensure hydraulic cylinder performance and longevity.
- Ports: Hydraulic fluid enters and exits the cylinder through ports located on either end of the cylinder barrel. Directional control valves regulate the flow of fluid into and out of the cylinder, controlling the direction of motion and speed of the piston.
- End Caps: End caps are securely attached to the cylinder barrel and house the ports and seals. They provide structural support and help maintain cylinder alignment during operation.
Hydraulic cylinders operate based on Pascal’s Law, which states that pressure applied to a confined fluid is transmitted undiminished in all directions. When hydraulic fluid is pumped into one chamber of the cylinder, it applies pressure against the piston, causing it to move linearly along the cylinder barrel. This movement exerts force on the rod, which can be used to lift, push, or pull external loads, depending on the application.
Hydraulic cylinders come in various types and configurations to suit different application requirements:
- Single-Acting Cylinders: These cylinders have hydraulic pressure applied to one side of the piston, with the return stroke typically achieved by a spring or external force. They are commonly used in applications where the load is moved in one direction only, such as lifting platforms or dump truck beds.
- Double-Acting Cylinders: These cylinders have hydraulic pressure applied to both sides of the piston, allowing for bidirectional movement and force generation. They are widely used in applications requiring precise control over both extension and retraction, such as in hydraulic presses, machine tools, and construction equipment.
Proper selection, sizing, installation, and maintenance of hydraulic cylinders are essential to ensure optimal performance, reliability, and safety in hydraulic systems. Engineers and technicians working with hydraulic systems must have a thorough understanding of hydraulic cylinder principles and applications to design, operate, and maintain hydraulic systems effectively in various industrial settings.
Hydraulic Fluid Contamination
Hydraulic fluid contamination refers to the presence of foreign substances or particles within the hydraulic fluid, which can adversely affect the performance, reliability, and lifespan of hydraulic systems. Contamination can occur during fluid handling, storage, or operation and can manifest in various forms, including solid particles, water, air, and chemical contaminants.
Common sources of hydraulic fluid contamination include:
- External Contaminants: Dirt, dust, debris, and other particulate matter can enter the hydraulic system through breather vents, seals, hoses, fittings, or reservoir openings. External contaminants are often introduced during maintenance, repairs, or operation in harsh environments such as construction sites or industrial facilities.
- Internal Contaminants: Internal sources of contamination include wear particles, corrosion products, and degradation by-products generated within the hydraulic system itself. Components such as pumps, valves, cylinders, and hoses can wear over time, releasing particles into the hydraulic fluid. Additionally, chemical degradation of the fluid due to high temperatures or oxidation can produce harmful by-products.
- Water Contamination: Water can enter the hydraulic system through condensation, leaks, or improper maintenance practices. Water contamination can lead to fluid degradation, corrosion of system components, and reduced lubricating properties, resulting in decreased system performance and reliability.
- Air Contamination: Air entrainment, or the presence of air bubbles in the hydraulic fluid, can occur due to cavitation, agitation, or improper system design. Air bubbles can cause reduced system efficiency, increased noise and vibration, and decreased component life due to fluid oxidation and loss of lubrication.
- Chemical Contaminants: Chemical contaminants such as acids, solvents, and cleaning agents can enter the hydraulic system through improper fluid handling, maintenance, or accidental spills. Chemical contamination can degrade fluid properties, cause corrosion of system components, and compromise system safety and performance.
Effects of hydraulic fluid contamination include:
- Reduced Efficiency: Contaminants can interfere with the smooth operation of hydraulic components, causing increased friction, wear, and energy losses within the system. This can lead to reduced system efficiency and increased energy consumption.
- Component Wear: Abrasive particles and contaminants can accelerate wear and tear on hydraulic components, leading to premature failure and downtime. Excessive wear can result in leaks, reduced system performance, and costly repairs or replacements.
- Fluid Degradation: Contaminants can degrade the quality and performance of hydraulic fluid, leading to decreased lubricating properties, increased viscosity, and reduced thermal stability. Degraded fluid can compromise system performance and reliability and necessitate more frequent fluid changes and maintenance.
- System Failure: Severe contamination can lead to system malfunctions, component failures, and catastrophic system damage. Contaminants such as water, air, and chemical contaminants can cause corrosion, erosion, and cavitation within the system, resulting in leaks, loss of pressure, and system failure.
Preventing hydraulic fluid contamination involves implementing proper maintenance practices, including:
- Regularly inspecting and replacing hydraulic fluid filters to remove contaminants.
- Ensuring proper fluid storage and handling procedures to prevent external contamination.
- Performing routine maintenance and inspections to identify and address internal sources of contamination.
- Using high-quality hydraulic fluids that meet system requirements and performance specifications.
- Implementing proper system design and component selection to minimize the risk of air entrainment, cavitation, and fluid degradation.
- Monitoring fluid condition and performing regular fluid analysis to detect contamination and degradation early and take corrective action.
By implementing proactive measures to prevent hydraulic fluid contamination, engineers and technicians can ensure the reliable operation, longevity, and performance of hydraulic systems in various industrial applications.
Hydraulic Filter
A hydraulic filter is a critical component in hydraulic systems designed to remove contaminants from hydraulic fluid, ensuring the cleanliness and integrity of the fluid and protecting system components from damage and premature wear. Hydraulic filters trap solid particles, water, air, and other contaminants present in the fluid, preventing them from circulating through the system and causing damage to pumps, valves, cylinders, and other hydraulic components.
Key components and characteristics of hydraulic filters include:
- Filter Element: The filter element is the primary component responsible for trapping contaminants in the hydraulic fluid. It consists of a porous medium, such as paper, mesh, or synthetic fibers, through which the hydraulic fluid flows. Contaminants are captured within the filter element, allowing only clean fluid to pass through.
- Filter Housing: The filter housing encloses the filter element and provides structural support and protection. It is typically made of metal or plastic and is designed to withstand hydraulic pressure and fluid flow rates while maintaining the integrity of the filter assembly.
- End Caps and Seals: End caps and seals are used to secure the filter element within the filter housing and prevent bypass or leakage of unfiltered fluid. They ensure proper sealing and alignment of the filter assembly and prevent fluid from bypassing the filter element.
- Filter Rating: The filter rating refers to the size of particles that the filter can effectively capture and retain. It is typically expressed in microns (µm) and indicates the minimum particle size that the filter can remove with a specified efficiency. Common filter ratings range from 1 µm to 100 µm, with finer ratings providing higher levels of filtration.
- Bypass Valve: Some hydraulic filters are equipped with a bypass valve that allows fluid to bypass the filter element under certain conditions, such as when the filter becomes clogged or the fluid viscosity is too high. The bypass valve prevents excessive pressure drop across the filter and ensures continuous fluid flow to critical system components, albeit with reduced filtration efficiency.
Hydraulic filters play a crucial role in maintaining the cleanliness and performance of hydraulic systems by:
- Removing solid particles, debris, and contaminants from the hydraulic fluid, preventing them from causing abrasion, erosion, or blockages in system components.
- Removing water and moisture from the hydraulic fluid, preventing corrosion, oxidation, and degradation of system components and fluid properties.
- Removing air and entrained gases from the hydraulic fluid, preventing cavitation, foaming, and reduced system efficiency and performance.
- Extending the service life of hydraulic system components, including pumps, valves, cylinders, and seals, by reducing wear and preventing damage caused by contaminants.
Proper selection, installation, and maintenance of hydraulic filters are essential to ensure optimal filtration performance and system reliability. Engineers and technicians must consider factors such as flow rate, pressure rating, filter rating, and compatibility with hydraulic fluid when selecting hydraulic filters for specific applications. Regular inspection, replacement, and monitoring of hydraulic filters are necessary to maintain proper filtration efficiency and protect hydraulic systems from contamination-related issues.
Hydraulic Hose
A hydraulic hose is a flexible, reinforced conduit used to transmit hydraulic fluid between hydraulic components, such as pumps, valves, cylinders, and motors, within a hydraulic system. It serves as a critical link in the hydraulic circuit, providing a pathway for the flow of pressurized fluid while accommodating movement, vibration, and thermal expansion within the system.
Key components and characteristics of hydraulic hoses include:
- Inner Tube: The inner tube is the innermost layer of the hydraulic hose and comes into direct contact with the hydraulic fluid. It is typically made of synthetic rubber or thermoplastic material resistant to the specific hydraulic fluid being used. The inner tube provides a smooth surface for fluid flow and prevents leakage.
- Reinforcement: The reinforcement layer(s) provide(s) strength, stability, and flexibility to the hydraulic hose, allowing it to withstand high-pressure hydraulic pulses and bending stresses without collapsing or rupturing. Common reinforcement materials include braided steel wire, spiral-wound steel wire, or textile fibers.
- Outer Cover: The outer cover is the outermost layer of the hydraulic hose and serves to protect the inner tube and reinforcement layers from environmental factors, abrasion, UV radiation, and mechanical damage. It is typically made of synthetic rubber, thermoplastic, or elastomeric material with excellent abrasion resistance and weathering properties.
- Fittings and Couplings: Fittings and couplings are used to connect hydraulic hoses to hydraulic components, forming leak-proof seals and providing a secure attachment point. Common types of fittings include crimped, swaged, or threaded fittings, which are selected based on hose size, pressure rating, and application requirements.
- Size and Pressure Rating: Hydraulic hoses are available in various sizes and pressure ratings to accommodate different flow rates, operating pressures, and application requirements. Hose size is determined by the inner diameter (ID) of the hose, typically measured in inches or millimeters, while pressure rating indicates the maximum allowable working pressure of the hose in pounds per square inch (psi) or bar.
- Temperature Range: Hydraulic hoses are designed to operate within specific temperature ranges, ranging from extreme cold to high heat environments. The temperature range depends on the materials used in the construction of the hose and the intended application. Specialized hoses with high-temperature or low-temperature capabilities are available for extreme operating conditions.
Hydraulic hoses are classified into several types based on construction and application:
- Wire-Reinforced Hoses: These hoses feature one or more layers of braided or spiral-wound steel wire reinforcement for high-pressure applications, such as heavy-duty machinery and industrial equipment.
- Textile-Reinforced Hoses: Textile-reinforced hoses utilize layers of high-strength textile fibers, such as nylon or polyester, for low to medium-pressure applications, such as agricultural machinery and mobile equipment.
- Thermoplastic Hoses: Thermoplastic hoses are lightweight, flexible hoses made of thermoplastic materials, offering excellent chemical resistance and flexibility for various industrial and mobile hydraulic applications.
Proper selection, installation, and maintenance of hydraulic hoses are essential to ensure optimal performance, reliability, and safety in hydraulic systems. Engineers and technicians must consider factors such as hose size, pressure rating, temperature range, compatibility with hydraulic fluid, and environmental conditions when selecting hydraulic hoses for specific applications. Regular inspection, replacement, and monitoring of hydraulic hoses are necessary to detect signs of wear, leakage, or damage and prevent costly downtime and hydraulic system failures.
Hydraulic Actuator
A hydraulic actuator is a device used in hydraulic systems to convert hydraulic energy into mechanical motion or force. It utilizes the pressure and flow of hydraulic fluid to generate linear or rotary motion, enabling the actuation of various mechanical components, such as valves, levers, arms, and machinery. Hydraulic actuators play a vital role in a wide range of industrial, automotive, aerospace, and construction applications, providing precise control, high force output, and reliable performance.
Key components and characteristics of hydraulic actuators include:
- Cylinder: In linear hydraulic actuators, a cylinder is used to convert fluid pressure into linear motion. The cylinder typically consists of a cylindrical barrel, a piston, and a rod. When hydraulic fluid is pressurized and applied to one side of the piston, it moves linearly, extending or retracting the rod depending on the direction of fluid flow.
- Motor: In rotary hydraulic actuators, a hydraulic motor is used to convert fluid pressure into rotary motion. The motor typically consists of a rotor, stator, and hydraulic ports. Pressurized hydraulic fluid enters the motor through ports, causing the rotor to rotate within the stator, generating rotary motion.
- Seals: Seals are used to prevent fluid leakage and maintain pressure within the hydraulic actuator. They are typically made of elastomeric materials, such as rubber or polyurethane, and are installed at various points within the actuator to ensure proper sealing between moving components.
- Valves: Hydraulic valves control the flow of hydraulic fluid into and out of the actuator, regulating its movement and speed. Directional control valves, flow control valves, and pressure control valves are commonly used to control hydraulic actuators based on system requirements.
- Mounting Arrangements: Hydraulic actuators are mounted or integrated into mechanical systems using various mounting arrangements, such as flange mounts, clevis mounts, trunnion mounts, or foot mounts. These mounting arrangements provide secure attachment points and ensure proper alignment and operation of the actuator within the system.
Hydraulic actuators can be classified into two main types based on their mode of operation:
- Linear Hydraulic Actuators: Linear actuators produce linear motion along a single axis and are commonly used in applications such as lifting, pushing, pulling, and positioning. They include hydraulic cylinders, telescopic cylinders, and hydraulic presses.
- Rotary Hydraulic Actuators: Rotary actuators produce rotary motion around a central axis and are used in applications such as valve actuation, material handling, and machinery operation. They include hydraulic motors, rotary vane actuators, and rotary hydraulic cylinders.
Hydraulic actuators offer several advantages over other types of actuators, including:
- High Force Output: Hydraulic actuators can generate high forces and torque outputs, making them suitable for applications requiring heavy lifting, pushing, or pulling.
- Precise Control: Hydraulic systems offer precise control over actuator speed, position, and force, allowing for accurate and repeatable motion control in various applications.
- Compact Design: Hydraulic actuators have a high power-to-weight ratio and can be designed in compact sizes, making them suitable for applications with limited space or weight constraints.
Proper selection, installation, and maintenance of hydraulic actuators are essential to ensure optimal performance, reliability, and safety in hydraulic systems. Engineers and technicians must consider factors such as actuator type, size, force output, speed, stroke length, and compatibility with hydraulic fluid when selecting hydraulic actuators for specific applications. Regular inspection, lubrication, and preventive maintenance are necessary to detect signs of wear, leakage, or damage and prevent costly downtime and hydraulic system failures.
Hydraulic Power Unit (HPU)
A Hydraulic Power Unit (HPU) is a self-contained system that generates, controls, and distributes hydraulic power to various components within a hydraulic system. It serves as the power source for hydraulic machinery and equipment, providing the necessary pressure, flow, and direction of hydraulic fluid to actuate hydraulic cylinders, motors, valves, and other hydraulic components. Hydraulic power units are widely used in various industrial, mobile, and marine applications where precise control, high force output, and reliable performance are required.
Key components and characteristics of hydraulic power units include:
- Prime Mover: The prime mover is the component responsible for generating mechanical energy to drive the hydraulic pump within the power unit. Common prime movers include electric motors, internal combustion engines (diesel or gasoline), and hydraulic motors. The selection of the prime mover depends on factors such as power requirements, operating environment, and energy source availability.
- Hydraulic Pump: The hydraulic pump is the primary component responsible for generating hydraulic pressure by converting mechanical energy from the prime mover into fluid energy. Various types of hydraulic pumps are used in hydraulic power units, including gear pumps, vane pumps, piston pumps, and axial piston pumps. The pump provides the necessary flow rate and pressure to meet the demands of the hydraulic system.
- Reservoir: The reservoir, also known as the hydraulic fluid tank, stores hydraulic fluid and provides a supply of fluid to the hydraulic pump. It also serves as a heat exchanger, dissipating heat generated during system operation and helping to maintain fluid temperature within acceptable limits. The reservoir is equipped with breather vents, filters, and level indicators to ensure proper fluid management and contamination control.
- Valves and Manifolds: Valves and manifolds control the flow, pressure, and direction of hydraulic fluid within the power unit and the hydraulic system. Directional control valves, pressure control valves, flow control valves, and check valves are commonly used to regulate fluid flow and pressure based on system requirements. Manifolds provide a compact and efficient means of connecting hydraulic components within the power unit and facilitating fluid distribution.
- Filtration System: The filtration system is essential for maintaining the cleanliness and integrity of the hydraulic fluid within the power unit. It consists of hydraulic filters and strainers that remove contaminants, such as dirt, debris, and particles, from the fluid to prevent damage to system components and ensure reliable operation. Proper filtration helps extend the service life of hydraulic components and reduce maintenance costs.
- Control System: The control system includes electrical, electronic, or hydraulic controls that regulate the operation of the hydraulic power unit and the hydraulic system. Control systems may include switches, sensors, relays, PLCs (Programmable Logic Controllers), and HMI (Human-Machine Interface) panels for monitoring system parameters, controlling pump operation, and providing user interface functionalities.
Hydraulic power units can be classified into several types based on their configuration, power source, and application:
- Standard Power Units: Standard power units are pre-engineered, off-the-shelf units designed for general-purpose hydraulic applications. They are available in various configurations, capacities, and power ratings to meet common hydraulic system requirements.
- Custom Power Units: Custom power units are specially designed and engineered to meet specific application requirements, such as unique performance specifications, environmental conditions, or space constraints. They are tailored to the needs of a particular application and may include specialized components, features, or controls.
- Portable Power Units: Portable power units are compact, lightweight units designed for mobile or temporary hydraulic applications, such as maintenance, repair, or rescue operations. They are equipped with wheels, handles, and integrated reservoirs for easy transport and deployment in the field.
Proper selection, installation, and maintenance of hydraulic power units are essential to ensure optimal performance, reliability, and safety in hydraulic systems. Engineers and technicians must consider factors such as system requirements, operating conditions, power ratings, control options, and environmental considerations when selecting hydraulic power units for specific applications. Regular inspection, preventive maintenance, and fluid analysis are necessary to detect and address issues such as leaks, contamination, and component wear and ensure the long-term integrity and performance of hydraulic power units and hydraulic systems.
Hydraulic Pressure
Hydraulic pressure is the force exerted by hydraulic fluid within a confined space or hydraulic system, resulting from the application of hydraulic force or the resistance to fluid flow. It is a fundamental concept in hydraulic systems and plays a crucial role in determining the performance, efficiency, and safety of hydraulic machinery and equipment.
Key aspects and characteristics of hydraulic pressure include:
- Force Transmission: Hydraulic pressure is used to transmit force from one point to another within a hydraulic system. When hydraulic fluid is pressurized by a hydraulic pump, the force is transmitted through the fluid to actuate hydraulic actuators, such as cylinders or motors, causing them to move or exert force on external loads.
- Pascal’s Law: Hydraulic pressure operates according to Pascal’s Law, which states that pressure applied to a confined fluid is transmitted undiminished in all directions. This principle allows hydraulic pressure to be effectively transmitted through hydraulic fluid to various points within a hydraulic system, enabling precise control and manipulation of mechanical components.
- Pressure Generation: Hydraulic pressure is generated by hydraulic pumps, which convert mechanical energy into hydraulic energy by pressurizing hydraulic fluid. The pump creates flow resistance within the system, causing pressure to build up, which is then transmitted through the fluid to actuate hydraulic components.
- Pressure Measurement: Hydraulic pressure is typically measured in pounds per square inch (psi), bar, or pascals (Pa). Pressure sensors, gauges, or transducers are used to measure hydraulic pressure at various points within the hydraulic system, providing operators with real-time feedback on system performance and operating conditions.
- Pressure Control: Hydraulic pressure is controlled and regulated using hydraulic valves, such as relief valves, pressure-reducing valves, and sequence valves. These valves adjust the flow rate, restrict fluid passage, or release excess pressure to maintain hydraulic pressure within specified limits and protect system components from damage due to overpressure conditions.
- Pressure Loss: Hydraulic pressure may experience losses due to friction, flow resistance, leakage, and other factors within the hydraulic system. Pressure losses can affect system efficiency, performance, and responsiveness and must be minimized through proper system design, component selection, and maintenance.
Hydraulic pressure is a critical parameter in hydraulic systems and directly influences system performance, efficiency, and safety. Engineers and technicians must carefully consider factors such as pressure requirements, flow rates, system design, component compatibility, and operating conditions when designing, operating, and maintaining hydraulic systems to ensure optimal performance and reliability. Proper pressure control, monitoring, and maintenance are essential to prevent overpressure conditions, system failures, and safety hazards in hydraulic machinery and equipment.
Hydraulic Fluid
Hydraulic fluid, also known as hydraulic oil or hydraulic fluid, is a specially formulated fluid used in hydraulic systems to transmit power, lubricate moving parts, and dissipate heat. It serves as the medium through which hydraulic force is transferred within the system, enabling the operation of hydraulic machinery and equipment in various industrial, mobile, and marine applications.
Key characteristics and properties of hydraulic fluid include:
- Viscosity: Viscosity refers to the resistance of a fluid to flow and is a critical property of hydraulic fluid. Hydraulic fluids are designed to have a specific viscosity range that ensures proper lubrication and hydraulic performance across a wide range of operating temperatures and conditions. Low-viscosity fluids flow more easily at low temperatures, while high-viscosity fluids provide better lubrication and film strength at high temperatures.
- Wear Protection: Hydraulic fluid must provide effective wear protection to reduce friction, wear, and abrasion between moving parts within the hydraulic system. Anti-wear additives, such as zinc dialkyldithiophosphate (ZDDP) and zinc di-n-butyl dithiophosphate (ZDDP), are commonly used to protect system components from wear and extend their service life.
- Oxidation Stability: Hydraulic fluid must resist oxidation and degradation when exposed to high temperatures, air, and moisture. Oxidation stability additives, such as antioxidants and anti-oxidation inhibitors, help prevent the formation of sludge, varnish, and deposits within the hydraulic system, maintaining fluid cleanliness and performance over time.
- Corrosion Protection: Hydraulic fluid must protect system components from corrosion and rust, particularly in systems exposed to moisture or harsh operating environments. Corrosion inhibitors, such as rust inhibitors and metal deactivators, are added to hydraulic fluid formulations to protect metal surfaces and extend component life.
- Foam Resistance: Hydraulic fluid must resist foaming, cavitation, and air entrainment to ensure proper hydraulic performance and component operation. Foam inhibitors and anti-foam additives are used to reduce foam formation and entrained air within the hydraulic system, maintaining system efficiency and stability.
- Water Separation: Hydraulic fluid must have the ability to separate from water and moisture to prevent emulsification and degradation of fluid properties. Water separation additives, such as demulsifiers and surfactants, help facilitate the separation of water from hydraulic fluid, allowing for efficient water removal and system protection.
- Compatibility: Hydraulic fluid must be compatible with system materials, seals, hoses, and components to prevent swelling, degradation, and leakage. Compatibility testing and compatibility charts provided by fluid manufacturers help ensure proper fluid selection and compatibility with system components.
Hydraulic fluids are available in various formulations and types, including mineral-based fluids, synthetic fluids, and water-based fluids, each offering different performance characteristics and suitability for specific applications. Proper fluid selection is essential to ensure optimal system performance, reliability, and longevity in hydraulic systems.
Regular monitoring, testing, and maintenance of hydraulic fluid are necessary to ensure proper fluid condition and performance. This includes routine fluid analysis, filtration, and periodic fluid replacement to remove contaminants, maintain proper fluid properties, and extend the service life of hydraulic systems. Proper fluid management practices, such as proper storage, handling, and disposal, are also important to ensure safe and efficient operation of hydraulic systems.
Hydraulic Valve
A hydraulic valve is a mechanical device used to control the flow, direction, and pressure of hydraulic fluid within a hydraulic system. It regulates the movement of hydraulic actuators, such as cylinders and motors, by directing the flow of fluid to different paths or blocking fluid flow altogether. Hydraulic valves play a crucial role in maintaining precise control, safety, and efficiency in hydraulic machinery and equipment across various industrial, mobile, and marine applications.
Key components and characteristics of hydraulic valves include:
- Valve Body: The valve body is the main housing of the hydraulic valve, containing internal passages, ports, and chambers through which hydraulic fluid flows. It provides structural support and serves as a mounting point for valve components.
- Valve Element: The valve element, such as a spool, poppet, or rotary disc, is the moving part of the valve that controls the flow of hydraulic fluid. It is actuated by external forces, such as mechanical actuators, solenoids, or pilot pressure, to open, close, or modulate fluid flow through the valve.
- Ports: Ports are openings in the valve body through which hydraulic fluid enters and exits the valve. Ports are connected to hydraulic lines, hoses, or components within the hydraulic system, allowing fluid to be directed to and from different parts of the system.
- Actuation Mechanism: The actuation mechanism is the mechanism responsible for moving the valve element to control fluid flow. It may include manual levers, knobs, handles, hydraulic actuators, pneumatic actuators, electric solenoids, or proportional control devices, depending on the type of valve and application requirements.
- Seals and Gaskets: Seals and gaskets are used to prevent leakage of hydraulic fluid from the valve and ensure proper sealing between moving parts. They are typically made of elastomeric materials, such as rubber or polyurethane, and are installed at critical points within the valve to maintain fluid integrity and prevent contamination.
- Valve Types: Hydraulic valves are available in various types and configurations to suit different application requirements, including:
- Directional Control Valves: These valves control the direction of hydraulic fluid flow within the system, allowing fluid to be directed to different hydraulic actuators or circuits. Common types include spool valves, poppet valves, and rotary valves.
- Pressure Control Valves: These valves regulate hydraulic fluid pressure within the system, maintaining pressure within predetermined limits to protect system components from damage. Examples include relief valves, pressure-reducing valves, and sequence valves.
- Flow Control Valves: These valves control the rate of hydraulic fluid flow within the system, allowing precise adjustment of flow rates to match system requirements. Types include flow control valves, throttle valves, and check valves.
- Proportional Control Valves: These valves provide variable control over flow, pressure, or direction of hydraulic fluid using proportional solenoids or pilot control mechanisms. They offer precise and proportional control over hydraulic system parameters, enabling advanced motion control and automation.
Hydraulic valves must be selected, installed, and maintained properly to ensure optimal performance, reliability, and safety in hydraulic systems. Factors such as valve type, size, flow capacity, pressure rating, actuation method, and compatibility with hydraulic fluid must be considered when selecting hydraulic valves for specific applications. Regular inspection, testing, and maintenance of hydraulic valves are necessary to detect and address issues such as leakage, wear, or malfunction and ensure the long-term integrity and performance of hydraulic systems.
Hydraulic Accumulator
A hydraulic accumulator is a device used in hydraulic systems to store and release hydraulic energy, providing a source of pressure and flow for various hydraulic components. It consists of a pressure vessel containing compressed gas (such as nitrogen) and hydraulic fluid, separated by a movable piston or bladder. Hydraulic accumulators are utilized in hydraulic systems to improve energy efficiency, absorb shocks and pressure fluctuations, supplement pump flow, and provide emergency backup power in case of pump failure.
Key components and characteristics of hydraulic accumulators include:
- Pressure Vessel: The pressure vessel is a cylindrical container that holds the compressed gas and hydraulic fluid. It is typically made of steel or composite materials capable of withstanding high pressure and fluid volumes. The pressure vessel is designed to be robust and leak-proof to ensure safe operation within the hydraulic system.
- Gas Charge: The gas charge, usually nitrogen, occupies the upper portion of the pressure vessel and is separated from the hydraulic fluid by a movable piston or bladder. The gas charge provides the stored energy potential in the accumulator and exerts pressure on the hydraulic fluid when compressed.
- Hydraulic Fluid: The hydraulic fluid fills the lower portion of the pressure vessel and surrounds the gas charge. It acts as a medium for storing and transferring hydraulic energy within the accumulator. Common hydraulic fluids used in accumulators include mineral oil, synthetic oil, and water-based fluids, depending on the application requirements.
- Piston or Bladder: The piston or bladder separates the gas charge from the hydraulic fluid and allows for the expansion and contraction of the gas volume as pressure changes occur. Pistons are typically used in piston-type accumulators, while bladder-type accumulators utilize a flexible bladder made of elastomeric material.
- Valves: Valves are used to control the flow of hydraulic fluid into and out of the accumulator, allowing for charging, discharging, and pressure regulation. Common valve types include inlet valves, outlet valves, check valves, and safety valves, which ensure proper operation and prevent overpressure conditions within the accumulator.
- Mounting and Installation: Hydraulic accumulators are mounted within the hydraulic system using appropriate brackets, supports, or mounting flanges. They are installed at strategic locations to optimize energy storage and delivery and minimize pressure fluctuations and shocks within the system.
Hydraulic accumulators are classified into several types based on their construction and operation:
- Piston-Type Accumulators: Piston accumulators consist of a cylindrical pressure vessel with a movable piston separating the gas and hydraulic fluid. The piston moves up and down within the cylinder as pressure changes occur, compressing or expanding the gas charge.
- Bladder-Type Accumulators: Bladder accumulators utilize a flexible bladder made of elastomeric material to separate the gas and hydraulic fluid. The bladder expands and contracts within the pressure vessel as pressure changes occur, providing a means of storing and releasing hydraulic energy.
- Diaphragm-Type Accumulators: Diaphragm accumulators use a flexible diaphragm instead of a bladder to separate the gas and hydraulic fluid. The diaphragm flexes in response to pressure changes, allowing for energy storage and release within the accumulator.
Hydraulic accumulators offer several advantages in hydraulic systems, including:
- Energy Storage: Accumulators store hydraulic energy, allowing for the release of stored energy during peak demand periods or when additional power is required.
- Pressure Stabilization: Accumulators help stabilize hydraulic system pressure by absorbing pressure spikes and fluctuations, ensuring smooth and consistent operation of hydraulic components.
- Emergency Backup: Accumulators provide emergency backup power in case of pump failure or power loss, allowing critical hydraulic functions to be maintained until normal operation is restored.
Proper selection, sizing, installation, and maintenance of hydraulic accumulators are essential to ensure optimal performance, reliability, and safety in hydraulic systems. Engineers and technicians must consider factors such as accumulator type, size, pressure rating, gas precharge pressure, and compatibility with hydraulic fluid when selecting accumulators for specific applications. Regular inspection, testing, and maintenance of accumulators are necessary to detect and address issues such as leakage, bladder degradation, or overpressure conditions and ensure the long-term integrity and performance of hydraulic systems.
Hydraulic Cylinder
A hydraulic cylinder is a mechanical actuator used to generate linear motion or force in hydraulic systems. It converts hydraulic energy into mechanical energy to perform various tasks, such as lifting, pushing, pulling, and positioning heavy loads or machinery. Hydraulic cylinders are essential components in a wide range of industrial, mobile, and marine applications, providing reliable and precise motion control in hydraulic machinery and equipment.
Key components and characteristics of hydraulic cylinders include:
- Cylinder Barrel: The cylinder barrel is the main body of the hydraulic cylinder, housing the piston, piston rod, and hydraulic fluid. It is typically made of high-strength steel or other durable materials capable of withstanding high pressure and loads. The inner surface of the cylinder barrel is honed to ensure smooth movement of the piston and reduce friction.
- Piston: The piston is a cylindrical component that divides the cylinder barrel into two chambers: the rod side and the cap side. It is sealed against the cylinder barrel to prevent leakage of hydraulic fluid between the chambers. The piston is connected to the piston rod and moves linearly within the cylinder barrel in response to changes in hydraulic pressure.
- Piston Rod: The piston rod is a cylindrical shaft that extends from the piston through a sealed opening in the cylinder barrel. It transmits the force generated by the piston to the external load or mechanism being actuated. The piston rod is typically made of hardened steel or other high-strength materials capable of withstanding bending and torsional forces.
- Seals and Gaskets: Seals and gaskets are used to prevent leakage of hydraulic fluid between the piston, piston rod, and cylinder barrel. They are typically made of elastomeric materials, such as rubber or polyurethane, and are installed at various points within the hydraulic cylinder to maintain fluid integrity and prevent contamination.
- End Caps: End caps are mounted on each end of the cylinder barrel to provide structural support and sealing for the hydraulic cylinder. They contain ports for hydraulic fluid inlet and outlet, as well as mounting provisions for attaching the cylinder to external components or machinery.
- Mounting Arrangements: Hydraulic cylinders are mounted or integrated into mechanical systems using various mounting arrangements, such as clevis mounts, trunnion mounts, flange mounts, or foot mounts. These mounting arrangements provide secure attachment points and ensure proper alignment and operation of the cylinder within the system.
Hydraulic cylinders are available in various types and configurations to suit different application requirements, including:
- Single-Acting Cylinders: Single-acting cylinders exert force in one direction only, typically using hydraulic pressure to extend the piston rod. A spring or gravity returns the piston rod to its original position when hydraulic pressure is released.
- Double-Acting Cylinders: Double-acting cylinders exert force in both directions, using hydraulic pressure to extend and retract the piston rod. Hydraulic fluid is supplied alternately to the rod side and cap side of the cylinder to control the direction of motion.
- Telescopic Cylinders: Telescopic cylinders consist of multiple nested stages that extend and retract telescopically, providing a long stroke length in a compact package. They are commonly used in applications where space constraints or long stroke lengths are required.
Proper selection, sizing, installation, and maintenance of hydraulic cylinders are essential to ensure optimal performance, reliability, and safety in hydraulic systems. Engineers and technicians must consider factors such as cylinder type, size, stroke length, force output, speed, and mounting configuration when selecting hydraulic cylinders for specific applications. Regular inspection, lubrication, and preventive maintenance are necessary to detect signs of wear, leakage, or damage and prevent costly downtime and hydraulic system failures.
Hydraulic Motor
A hydraulic motor is a mechanical device used to convert hydraulic pressure and fluid flow into rotational mechanical power. It serves as the actuator in hydraulic systems to drive machinery, equipment, and vehicles by providing rotary motion. Hydraulic motors are widely used in various industrial, mobile, and marine applications where high torque, variable speed, and precise control are required.
Key components and characteristics of hydraulic motors include:
- Rotor: The rotor is the rotating component of the hydraulic motor that converts hydraulic energy into mechanical energy. It typically consists of a shaft or spindle connected to an output mechanism, such as gears, pistons, or vanes, that interacts with the hydraulic fluid to produce rotational motion.
- Stator: The stator is the stationary component of the hydraulic motor that contains the hydraulic fluid and provides a housing for the rotor. It is typically mounted within the hydraulic system and acts as a support structure for the rotor, guiding the flow of hydraulic fluid and controlling the direction of rotation.
- Hydraulic Ports: Hydraulic ports are openings in the hydraulic motor through which hydraulic fluid enters and exits the motor. Pressurized hydraulic fluid is supplied to the motor through inlet ports, causing the rotor to rotate, while outlet ports allow fluid to exit the motor after energy conversion has occurred.
- Actuation Mechanism: The actuation mechanism controls the flow of hydraulic fluid into and out of the motor, regulating the speed, direction, and torque output of the motor. It may include valves, throttles, or control devices that adjust the flow rate and pressure of hydraulic fluid to match the requirements of the application.
- Seals and Bearings: Seals and bearings are used to prevent leakage of hydraulic fluid and provide support and alignment for the rotating components of the hydraulic motor. Seals prevent fluid from escaping between the rotor and stator, while bearings reduce friction and wear on moving parts.
- Mounting Arrangements: Hydraulic motors are mounted or integrated into mechanical systems using various mounting arrangements, such as flange mounts, foot mounts, or shaft mounts. These mounting arrangements provide secure attachment points and ensure proper alignment and operation of the motor within the system.
Hydraulic motors are classified into several types based on their operating principle and construction, including:
- Gear Motors: Gear motors utilize meshing gears to transfer hydraulic energy from the fluid to the rotor, producing rotational motion. They are compact, efficient, and suitable for low to moderate torque applications.
- Vane Motors: Vane motors use sliding vanes or blades to interact with the hydraulic fluid and convert energy into rotational motion. They offer high efficiency, smooth operation, and variable speed control, making them ideal for medium to high torque applications.
- Piston Motors: Piston motors use reciprocating pistons to generate rotary motion by alternately pressurizing and displacing hydraulic fluid within the motor. They offer high torque output, precise control, and variable displacement capabilities, making them suitable for heavy-duty applications.
Hydraulic motors offer several advantages over other types of motors, including:
- High Torque Output: Hydraulic motors can generate high torque output at low speeds, making them ideal for applications requiring high starting torque or heavy load handling.
- Variable Speed Control: Hydraulic motors offer variable speed control by adjusting the flow rate and pressure of hydraulic fluid, allowing for precise speed regulation and control over machine operation.
- Compact Design: Hydraulic motors have a high power-to-weight ratio and can be designed in compact sizes, making them suitable for applications with limited space or weight constraints.
Proper selection, sizing, installation, and maintenance of hydraulic motors are essential to ensure optimal performance, reliability, and safety in hydraulic systems. Engineers and technicians must consider factors such as motor type, size, speed, torque, efficiency, and compatibility with hydraulic fluid when selecting hydraulic motors for specific applications. Regular inspection, lubrication, and preventive maintenance are necessary to detect signs of wear, leakage, or damage and prevent costly downtime and hydraulic system failures.
Hydraulic Pump
A hydraulic pump is a mechanical device used to generate flow and pressure of hydraulic fluid within a hydraulic system. It serves as the primary power source for hydraulic machinery and equipment, providing the force necessary to actuate hydraulic cylinders, motors, valves, and other components. Hydraulic pumps are essential components in various industrial, mobile, and marine applications where precise control, high pressure, and reliable performance are required.
Key components and characteristics of hydraulic pumps include:
- Pump Housing: The pump housing, also known as the pump body or casing, contains the internal components of the hydraulic pump and provides structural support and protection. It is typically made of durable materials such as cast iron, aluminum, or steel and is designed to withstand high pressure and loads.
- Rotating Element: The rotating element, such as gears, pistons, or vanes, is the component responsible for generating flow and pressure within the hydraulic pump. It interacts with the hydraulic fluid to create hydraulic energy and transmit it to the hydraulic system. Different types of hydraulic pumps use different types of rotating elements, each with its advantages and limitations.
- Inlet Port: The inlet port is the opening through which hydraulic fluid enters the pump from the hydraulic reservoir or supply line. It directs fluid into the pump’s suction chamber, where it is pressurized and sent to the pump’s discharge side.
- Outlet Port: The outlet port is the opening through which pressurized hydraulic fluid exits the pump and is directed to the hydraulic system’s components. It delivers flow and pressure to actuate hydraulic cylinders, motors, valves, and other devices within the system.
- Seals and Bearings: Seals and bearings are used to prevent leakage of hydraulic fluid and provide support and alignment for the rotating elements of the hydraulic pump. Seals prevent fluid from escaping between moving parts, while bearings reduce friction and wear on pump components.
- Drive Mechanism: The drive mechanism is the method by which the hydraulic pump is powered and driven. It may include a motor, engine, or other power source that drives the rotating element of the pump to generate hydraulic pressure and flow.
Hydraulic pumps are classified into several types based on their operating principle and construction, including:
- Gear Pumps: Gear pumps use meshing gears to create flow and pressure within the hydraulic system. They are simple, compact, and cost-effective, making them suitable for low to moderate pressure and flow applications.
- Piston Pumps: Piston pumps use reciprocating pistons to generate flow and pressure by alternately pressurizing and displacing hydraulic fluid within the pump. They offer high efficiency, variable displacement capabilities, and precise control, making them suitable for high-pressure applications.
- Vane Pumps: Vane pumps use sliding vanes or blades to create flow and pressure by displacing hydraulic fluid within the pump. They offer smooth operation, low noise levels, and moderate pressure and flow capabilities, making them suitable for a wide range of applications.
Hydraulic pumps play a critical role in hydraulic systems by providing the energy necessary to operate hydraulic machinery and equipment. Proper selection, sizing, installation, and maintenance of hydraulic pumps are essential to ensure optimal performance, reliability, and safety in hydraulic systems. Engineers and technicians must consider factors such as pump type, size, flow rate, pressure rating, efficiency, and compatibility with hydraulic fluid when selecting hydraulic pumps for specific applications. Regular inspection, lubrication, and preventive maintenance are necessary to detect signs of wear, leakage, or damage and prevent costly downtime and hydraulic system failures.
Hydraulic Filter
A hydraulic filter is a crucial component in hydraulic systems designed to remove contaminants and impurities from hydraulic fluid, ensuring the cleanliness and proper operation of hydraulic machinery and equipment. It plays a vital role in maintaining system performance, reliability, and longevity by preventing damage to sensitive hydraulic components and minimizing the risk of system failures.
Key components and characteristics of hydraulic filters include:
- Filter Element: The filter element is the heart of the hydraulic filter, responsible for capturing and retaining contaminants present in the hydraulic fluid. It is typically made of a porous material, such as cellulose, synthetic fibers, metal mesh, or micro-glass fibers, with specific filtration ratings to trap particles of varying sizes. The filter element may be cylindrical, pleated, or disc-shaped, depending on the filter design and application requirements.
- Filter Housing: The filter housing is the outer casing or enclosure that holds the filter element and directs hydraulic fluid through the filtration process. It is typically made of metal, plastic, or other durable materials capable of withstanding hydraulic pressure and fluid flow. The housing provides structural support, protection, and sealing for the filter element and ensures proper alignment within the hydraulic system.
- Inlet and Outlet Ports: The inlet and outlet ports are openings in the filter housing through which hydraulic fluid enters and exits the filter. Pressurized hydraulic fluid flows into the filter through the inlet port, passes through the filter element, and exits the filter through the outlet port, having been cleaned of contaminants. The ports may be threaded, flanged, or connected to hydraulic lines or hoses, depending on the filter design and installation requirements.
- Bypass Valve: The bypass valve is a safety feature built into some hydraulic filters to prevent excessive pressure drop across the filter element in case of clogging or blockage. It allows a controlled amount of unfiltered fluid to bypass the filter element and continue circulating within the hydraulic system, ensuring continuous operation and preventing damage to sensitive components during filter maintenance or replacement.
- Indicator or Gauge: Some hydraulic filters are equipped with an indicator or gauge to monitor filter condition and performance. The indicator may be a visual indicator, such as a colored pop-up flag or a pressure gauge, that provides a visual indication of filter clogging or pressure drop. This allows operators to monitor filter condition and schedule maintenance or replacement as needed to ensure optimal system performance.
Hydraulic filters are available in various types and configurations to suit different application requirements, including:
- Suction Strainers: Suction strainers are located at the inlet port of hydraulic pumps to prevent large particles and debris from entering the pump and causing damage. They typically consist of a wire mesh or perforated screen that traps contaminants while allowing hydraulic fluid to flow freely.
- In-line Filters: In-line filters are installed in hydraulic lines or hoses to remove contaminants from hydraulic fluid as it circulates through the system. They can be installed in various orientations, such as in-line, T-line, or manifold-mounted, and are available in disposable or reusable cartridge styles.
- Return Line Filters: Return line filters are installed in the return line of hydraulic systems to remove contaminants before hydraulic fluid returns to the reservoir. They help maintain cleanliness and integrity of hydraulic fluid and prevent contamination of sensitive system components.
Proper selection, sizing, installation, and maintenance of hydraulic filters are essential to ensure optimal performance, reliability, and safety in hydraulic systems. Engineers and technicians must consider factors such as filter type, size, filtration rating, flow capacity, pressure rating, and compatibility with hydraulic fluid when selecting hydraulic filters for specific applications. Regular inspection, monitoring, and replacement of hydraulic filters are necessary to ensure proper filtration and prevent contamination-related issues in hydraulic systems.
Hydraulic Reservoir
A hydraulic reservoir, also known as a hydraulic tank or hydraulic fluid reservoir, is a container used to store hydraulic fluid in a hydraulic system. It serves several essential functions, including storing hydraulic fluid, dissipating heat, removing air and contaminants, and providing a reservoir for fluid expansion and contraction. Hydraulic reservoirs are integral components of hydraulic systems and play a crucial role in maintaining system performance, reliability, and longevity.
Key components and characteristics of hydraulic reservoirs include:
- Reservoir Body: The reservoir body is the main container or enclosure that holds the hydraulic fluid. It is typically made of steel, aluminum, or plastic and is designed to withstand hydraulic pressure, fluid volume, and environmental conditions. The reservoir body may be cylindrical, rectangular, or custom-shaped, depending on space constraints and installation requirements.
- Fluid Level Sight Gauge: The fluid level sight gauge is a transparent or translucent tube or window installed on the side of the reservoir to visually indicate the fluid level inside the reservoir. It allows operators to monitor fluid level and determine when fluid needs to be added or replenished. Some reservoirs may also include electronic level sensors or switches for automated monitoring and control.
- Filler Breather Cap: The filler breather cap is a component installed on top of the reservoir to provide access for filling and replenishing hydraulic fluid. It typically includes a breather vent to allow air to enter and exit the reservoir as fluid level changes and to prevent pressure buildup inside the reservoir. The filler breather cap may also incorporate a filter or strainer to prevent contaminants from entering the reservoir during fluid replenishment.
- Return Line Connection: The return line connection is an opening or port located at the top or side of the reservoir through which hydraulic fluid returns from the hydraulic system. It is connected to the return line of hydraulic components or valves, allowing fluid to flow back into the reservoir after performing work in the system. The return line connection may include a diffuser or baffle to minimize fluid agitation and foaming.
- Drain Port: The drain port is an opening or port located at the bottom of the reservoir to facilitate draining and maintenance of hydraulic fluid. It allows operators to remove old or contaminated fluid from the reservoir and replace it with fresh fluid during routine maintenance or servicing. The drain port may include a plug, valve, or quick-disconnect fitting for easy access and operation.
- Heat Exchanger: Some hydraulic reservoirs are equipped with built-in heat exchangers or cooling fins to dissipate heat generated during system operation. The heat exchanger helps maintain optimal fluid temperature and prevents overheating, which can degrade fluid properties and reduce system performance and efficiency.
Hydraulic reservoirs are available in various sizes, shapes, and configurations to suit different application requirements, including:
- Integral Reservoirs: Integral reservoirs are built into hydraulic components or machinery, such as hydraulic pumps, motors, or cylinders, and serve as an integrated part of the system. They are compact and space-saving, ideal for applications with limited space or weight constraints.
- Stand-Alone Reservoirs: Stand-alone reservoirs are separate containers or tanks mounted externally to hydraulic systems and connected via hoses or tubing. They provide flexibility in system layout and installation and allow for easy access and maintenance of hydraulic fluid.
Proper selection, sizing, installation, and maintenance of hydraulic reservoirs are essential to ensure optimal performance, reliability, and safety in hydraulic systems. Engineers and technicians must consider factors such as reservoir capacity, shape, mounting options, fluid compatibility, and environmental conditions when selecting hydraulic reservoirs for specific applications. Regular inspection, cleaning, and fluid analysis are necessary to maintain proper fluid level, cleanliness, and temperature within hydraulic reservoirs and prevent contamination-related issues in hydraulic systems.
Hydraulic Hose
A hydraulic hose is a flexible tube designed to transport hydraulic fluid between hydraulic components, such as pumps, valves, cylinders, and motors, within a hydraulic system. It serves as the conduit through which hydraulic pressure, flow, and energy are transmitted, enabling the operation of hydraulic machinery and equipment in various industrial, mobile, and marine applications.
Key components and characteristics of hydraulic hoses include:
- Inner Tube: The inner tube is the innermost layer of the hydraulic hose and comes into direct contact with hydraulic fluid. It is typically made of synthetic rubber, thermoplastic, or synthetic elastomeric materials resistant to hydraulic fluid, temperature, and pressure. The inner tube provides a smooth, seamless surface for fluid flow and prevents leakage or absorption of hydraulic fluid.
- Reinforcement Layer: The reinforcement layer provides structural support and strength to the hydraulic hose, allowing it to withstand high pressure, bending, and vibration within the hydraulic system. It may consist of one or more layers of braided wire, spiral wire, or textile fibers embedded within or wrapped around the inner tube. The reinforcement layer enhances hose durability, flexibility, and resistance to kinking, abrasion, and external damage.
- Outer Cover: The outer cover is the outermost layer of the hydraulic hose and provides protection against environmental factors, abrasion, UV radiation, chemicals, and mechanical damage. It is typically made of synthetic rubber, thermoplastic, or elastomeric materials with excellent weathering and abrasion resistance. The outer cover may also be reinforced with additional layers for added strength and durability.
- Fittings and Connectors: Fittings and connectors are used to attach hydraulic hoses to hydraulic components, such as pumps, valves, cylinders, and motors, and create sealed connections for fluid transfer. They come in various types, sizes, and configurations, including threaded fittings, flange fittings, quick-disconnect couplings, and crimped or reusable fittings. Proper selection and installation of fittings and connectors are essential to ensure leak-free connections and optimal performance of hydraulic hoses.
- Hose Assembly: A hose assembly consists of a hydraulic hose and associated fittings and connectors assembled together to form a complete hydraulic line or circuit. Hose assemblies are customized to specific lengths, sizes, and configurations to suit the requirements of the hydraulic system and application. They are typically assembled and crimped or swaged by trained technicians using specialized equipment to ensure proper fit and integrity.
Hydraulic hoses are available in various types, sizes, pressure ratings, and temperature ranges to suit different application requirements, including:
- Low-Pressure Hoses: Low-pressure hoses are designed for applications with operating pressures up to 3000 psi and are commonly used for hydraulic return lines, suction lines, and low-pressure fluid transfer.
- Medium-Pressure Hoses: Medium-pressure hoses are designed for applications with operating pressures ranging from 3000 psi to 6000 psi and are used for hydraulic systems, mobile equipment, and industrial machinery.
- High-Pressure Hoses: High-pressure hoses are designed for applications with operating pressures exceeding 6000 psi and are used in heavy-duty hydraulic systems, construction equipment, mining machinery, and hydraulic power units.
Proper selection, sizing, installation, and maintenance of hydraulic hoses are essential to ensure optimal performance, reliability, and safety in hydraulic systems. Engineers and technicians must consider factors such as hose type, size, pressure rating, temperature rating, bend radius, compatibility with hydraulic fluid, and environmental conditions when selecting hydraulic hoses for specific applications. Regular inspection, testing, and replacement of hydraulic hoses are necessary to detect signs of wear, damage, or leakage and prevent hydraulic system failures and safety hazards.
Hydraulic Fluid
Hydraulic fluid, also known as hydraulic oil or hydraulic fluid, is a specialized liquid used in hydraulic systems to transmit power, lubricate components, dissipate heat, and protect against corrosion and wear. It serves as the medium through which hydraulic energy is transferred within the system, enabling the operation of hydraulic machinery and equipment in various industrial, mobile, and marine applications.
Key components and characteristics of hydraulic fluid include:
- Base Oil: The base oil is the primary component of hydraulic fluid and provides lubrication and hydraulic transmission properties. It is typically derived from refined petroleum crude oil or synthetic sources, such as synthetic hydrocarbons, esters, or glycols. Base oils are selected based on factors such as viscosity, thermal stability, oxidation resistance, and compatibility with system materials and seals.
- Additives: Additives are chemical compounds added to hydraulic fluid to enhance its performance and properties. Common additives include anti-wear agents, antioxidants, corrosion inhibitors, viscosity modifiers, anti-foaming agents, and anti-rust agents. Additives help improve fluid stability, lubricity, cleanliness, and longevity, and protect hydraulic components against wear, oxidation, and degradation.
- Viscosity: Viscosity is a measure of a fluid’s resistance to flow and deformation and is a critical property of hydraulic fluid. Proper viscosity ensures efficient hydraulic energy transmission, lubrication, and component protection within the system. Hydraulic fluids are available in different viscosity grades, such as ISO VG (viscosity grade), to suit specific operating temperatures, flow rates, and pressure ranges.
- Temperature Stability: Temperature stability refers to the ability of hydraulic fluid to maintain its viscosity and performance characteristics over a wide range of temperatures. Hydraulic systems operate in various environmental conditions, from extreme cold to high heat, and require fluid that can withstand temperature fluctuations without compromising performance. Synthetic hydraulic fluids offer superior temperature stability compared to mineral-based fluids and are suitable for demanding applications.
- Water Content: Water content in hydraulic fluid can lead to fluid degradation, corrosion, and reduced lubrication and performance. Hydraulic fluids should have low water content to maintain system integrity and prevent damage to hydraulic components. Water-contaminated hydraulic fluid should be promptly drained and replaced with fresh fluid to avoid system failure and damage.
- Compatibility: Hydraulic fluid compatibility refers to its ability to interact safely with system materials, seals, hoses, and components without causing deterioration or degradation. Proper fluid compatibility ensures system reliability, longevity, and performance. It is essential to use hydraulic fluid recommended by equipment manufacturers and avoid mixing different types or brands of fluid to prevent compatibility issues.
Hydraulic fluids are available in various types and formulations to suit different application requirements and operating conditions, including:
- Mineral-Based Hydraulic Fluids: Mineral-based hydraulic fluids, also known as petroleum-based fluids, are derived from refined petroleum crude oil and are widely used in hydraulic systems. They offer good lubrication, temperature stability, and cost-effectiveness and are suitable for general-purpose hydraulic applications.
- Synthetic Hydraulic Fluids: Synthetic hydraulic fluids are formulated from synthetic base oils and additives and offer superior performance and properties compared to mineral-based fluids. They provide excellent temperature stability, oxidation resistance, wear protection, and compatibility with system materials. Synthetic hydraulic fluids are used in high-performance hydraulic systems, extreme operating conditions, and environmentally sensitive applications.
Proper selection, handling, storage, and maintenance of hydraulic fluid are essential to ensure optimal performance, reliability, and safety in hydraulic systems. Engineers and technicians must consider factors such as fluid type, viscosity grade, temperature stability, water content, compatibility, and manufacturer recommendations when selecting hydraulic fluid for specific applications. Regular fluid analysis, monitoring, and replacement are necessary to maintain proper fluid condition and prevent contamination, degradation, and hydraulic system failures.
Hydraulic Valve
A hydraulic valve is a mechanical device used to control the flow, pressure, and direction of hydraulic fluid within a hydraulic system. It serves as the key component for regulating the operation of hydraulic machinery and equipment, enabling precise control and manipulation of hydraulic energy to perform various tasks in industrial, mobile, and marine applications.
Key components and characteristics of hydraulic valves include:
- Valve Body: The valve body is the main housing or enclosure that contains the internal components of the hydraulic valve and provides structural support and protection. It is typically made of metal, such as steel or aluminum, and is designed to withstand high pressure, fluid flow, and environmental conditions. The valve body may include inlet and outlet ports, mounting provisions, and connection points for hydraulic lines or hoses.
- Internal Components: Hydraulic valves contain various internal components, such as spools, poppets, discs, balls, and seats, that control the flow, pressure, and direction of hydraulic fluid within the valve. These components move or actuate in response to changes in hydraulic pressure, flow rate, or external signals, such as manual, electrical, or mechanical inputs.
- Actuation Mechanism: The actuation mechanism is the method by which the hydraulic valve is operated and controlled. It may include manual levers, knobs, or handles for manual operation, solenoids or electric motors for electrical operation, or pneumatic actuators for pneumatic operation. The actuation mechanism controls the position or movement of internal components within the valve to regulate fluid flow and pressure according to system requirements.
- Flow Path: The flow path refers to the internal passages or channels within the hydraulic valve through which hydraulic fluid flows. It includes inlet and outlet ports, control orifice, and flow passages that direct fluid flow through the valve. The flow path may be configured to provide various flow control functions, such as on/off, proportional, directional, or pressure control.
- Spool or Poppet: The spool or poppet is a movable element within the hydraulic valve that controls fluid flow by blocking, diverting, or allowing flow through the valve. Spool valves use cylindrical or tapered spools that slide within a valve body to regulate flow, while poppet valves use spring-loaded discs or balls that seat against valve openings to control flow. Spools and poppets are actuated by external forces or signals to change valve position and function.
Hydraulic valves are classified into several types based on their function, configuration, and operation, including:
- Directional Control Valves: Directional control valves regulate the direction of hydraulic fluid flow within a hydraulic system, allowing fluid to be directed to specific actuators or hydraulic components. They include various types such as spool valves, poppet valves, rotary valves, and check valves, and can be manually operated, electrically actuated, or mechanically controlled.
- Pressure Control Valves: Pressure control valves regulate the pressure of hydraulic fluid within a hydraulic system, ensuring that it stays within safe operating limits. They include relief valves, pressure reducing valves, sequence valves, and pressure-compensated valves, which control pressure by diverting excess fluid flow or adjusting flow rates in response to changes in system pressure.
- Flow Control Valves: Flow control valves regulate the rate of hydraulic fluid flow within a hydraulic system, allowing precise control of actuator speed and movement. They include throttle valves, flow control valves, and flow dividers, which restrict or regulate fluid flow through adjustable orifices, valves, or bypass circuits.
Proper selection, sizing, installation, and maintenance of hydraulic valves are essential to ensure optimal performance, reliability, and safety in hydraulic systems. Engineers and technicians must consider factors such as valve type, size, flow capacity, pressure rating, actuation method, and compatibility with hydraulic fluid when selecting hydraulic valves for specific applications. Regular inspection, testing, and adjustment of hydraulic valves are necessary to ensure proper function, responsiveness, and efficiency in hydraulic systems.
Hydraulic Accumulator
A hydraulic accumulator is a device used to store hydraulic energy in the form of pressurized fluid, typically hydraulic oil, for later use in hydraulic systems. It serves as a supplementary power source to improve system performance, efficiency, and responsiveness by providing temporary energy storage, pressure stabilization, and shock absorption capabilities.
Key components and characteristics of hydraulic accumulators include:
- Pressure Vessel: The pressure vessel is the main housing or container of the hydraulic accumulator, designed to withstand high pressure and store pressurized hydraulic fluid. It is typically cylindrical or spherical in shape and made of durable materials, such as steel or aluminum, capable of withstanding hydraulic pressure and fluid volume. The pressure vessel may be welded or bolted together and equipped with end caps, seals, and fittings to contain hydraulic fluid and maintain pressure.
- Diaphragm or Piston: The diaphragm or piston is the movable element within the hydraulic accumulator that separates the hydraulic fluid from a gas or spring chamber. It flexes or moves in response to changes in hydraulic pressure, compressing or expanding the gas or spring to store or release hydraulic energy. Diaphragm-type accumulators use a flexible elastomeric diaphragm, while piston-type accumulators use a solid piston to separate the fluid and gas chambers.
- Gas or Spring Chamber: The gas or spring chamber is the space within the hydraulic accumulator containing compressed gas or a spring mechanism. It serves as the energy storage medium and provides the force necessary to compress or expand the diaphragm or piston when hydraulic fluid is introduced or discharged from the accumulator. Compressed gas, such as nitrogen, is commonly used due to its compressibility and stability over a wide pressure range.
- Inlet and Outlet Ports: The inlet and outlet ports are openings or connections on the hydraulic accumulator through which hydraulic fluid enters and exits the accumulator. Pressurized hydraulic fluid is supplied to the accumulator through the inlet port, compressing the gas or spring and storing energy. When hydraulic energy is required, fluid is discharged from the accumulator through the outlet port, releasing stored energy to the hydraulic system.
- Safety Features: Hydraulic accumulators may include safety features to prevent over-pressurization, leakage, or failure during operation. These features may include pressure relief valves, rupture discs, burst plugs, or pressure gauges that monitor and control accumulator pressure within safe limits. Proper sizing, installation, and maintenance of safety features are essential to ensure accumulator integrity and prevent safety hazards.
Hydraulic accumulators are classified into several types based on their operating principle and construction, including:
- Diaphragm Accumulators: Diaphragm accumulators use a flexible elastomeric diaphragm to separate the hydraulic fluid and gas chambers. They offer compact size, high energy storage capacity, and excellent gas-sealing properties, making them suitable for a wide range of hydraulic applications.
- Piston Accumulators: Piston accumulators use a solid piston to separate the hydraulic fluid and gas chambers. They offer precise control of gas precharge pressure, fast response times, and high efficiency, making them ideal for high-performance hydraulic systems and applications requiring rapid energy release.
- Bladder Accumulators: Bladder accumulators use a flexible elastomeric bladder to separate the hydraulic fluid and gas chambers. They offer high energy storage capacity, low gas permeation, and compatibility with a wide range of fluids and gases, making them suitable for demanding hydraulic systems and harsh operating conditions.
Hydraulic accumulators play a critical role in hydraulic systems by providing energy storage, pressure stabilization, and shock absorption capabilities. Proper selection, sizing, installation, and maintenance of hydraulic accumulators are essential to ensure optimal performance, reliability, and safety in hydraulic systems. Engineers and technicians must consider factors such as accumulator type, size, pressure rating, gas precharge, fluid compatibility, and safety features when selecting hydraulic accumulators for specific applications. Regular inspection, testing, and maintenance of hydraulic accumulators are necessary to detect signs of wear, leakage, or failure and prevent hydraulic system downtime and safety hazards.
Hydraulic Cylinder
A hydraulic cylinder is a mechanical actuator that converts hydraulic energy into linear mechanical force and motion. It consists of a cylindrical barrel, piston, and rod assembly, which work together to exert force in a straight-line direction. Hydraulic cylinders are widely used in various industrial, mobile, and marine applications to perform tasks such as lifting, pushing, pulling, and positioning heavy loads or equipment.
Key components and characteristics of hydraulic cylinders include:
- Cylinder Barrel: The cylinder barrel is the main body or housing of the hydraulic cylinder, typically made of high-strength steel or aluminum alloy. It provides structural support and contains the internal components of the cylinder, such as the piston, seals, and hydraulic fluid. The cylinder barrel is precision machined to ensure smooth operation, precise alignment, and minimal friction between moving parts.
- Piston: The piston is a cylindrical or disc-shaped component that divides the cylinder barrel into two chambers: the rod side and the cap side. It is fitted with seals to prevent leakage of hydraulic fluid and transmits hydraulic pressure from one chamber to the other to generate linear motion. The piston may be single-acting, with hydraulic pressure acting on one side only, or double-acting, with hydraulic pressure acting on both sides for bi-directional movement.
- Piston Rod: The piston rod is a cylindrical shaft that extends from the piston through a sealed opening in the cylinder barrel. It transmits the force generated by the hydraulic pressure to external loads or equipment and provides the connection point for mounting attachments, such as end fittings, clevises, or eye brackets. The piston rod is typically made of hardened and chrome-plated steel to resist wear, corrosion, and damage.
- Seals: Seals are used to prevent leakage of hydraulic fluid and maintain pressure and integrity within the hydraulic cylinder. They are located at various points along the cylinder assembly, including the piston, piston rod, and cylinder end caps, and are exposed to hydraulic fluid and high pressures during operation. Common types of seals used in hydraulic cylinders include O-rings, lip seals, piston seals, and rod seals, which are selected based on factors such as temperature, pressure, fluid compatibility, and application requirements.
- End Caps: The end caps are cylindrical or disc-shaped components attached to the ends of the cylinder barrel to enclose and seal the cylinder assembly. They provide mounting surfaces for attaching the hydraulic cylinder to external equipment or structures and may include ports or connections for hydraulic fluid inlet and outlet. End caps are typically made of steel or aluminum and are securely fastened to the cylinder barrel using bolts or welds.
Hydraulic cylinders are classified into several types based on their construction, mounting, and application, including:
- Single-Acting Cylinders: Single-acting cylinders utilize hydraulic pressure to act on one side of the piston, generating linear motion in one direction. They rely on external forces, such as gravity or springs, to return the piston to its original position when hydraulic pressure is released.
- Double-Acting Cylinders: Double-acting cylinders utilize hydraulic pressure to act on both sides of the piston, generating bi-directional linear motion. They are commonly used in applications requiring precise control over both extension and retraction movements.
- Telescopic Cylinders: Telescopic cylinders consist of multiple nested stages or sleeves that extend and retract telescopically, providing a compact and extended stroke length. They are used in applications with limited space or where extended reach is required, such as dump trucks, cranes, and aerial lifts.
Proper selection, sizing, installation, and maintenance of hydraulic cylinders are essential to ensure optimal performance, reliability, and safety in hydraulic systems. Engineers and technicians must consider factors such as cylinder type, size, stroke length, bore diameter, rod diameter, operating pressure, mounting configuration, and application requirements when selecting hydraulic cylinders for specific tasks. Regular inspection, lubrication, and preventive maintenance are necessary to detect signs of wear, leakage, or damage and prevent hydraulic cylinder failure and safety hazards.
Hydraulic Motor
A hydraulic motor is a mechanical device that converts hydraulic pressure and flow into rotational mechanical energy, providing power to drive various types of machinery and equipment. It operates in a similar manner to a hydraulic pump but in reverse, utilizing the energy of pressurized hydraulic fluid to produce rotary motion instead of linear motion. Hydraulic motors are widely used in industrial, mobile, and marine applications to drive machinery such as conveyor belts, winches, cranes, and rotary actuators.
Key components and characteristics of hydraulic motors include:
- Rotor: The rotor is the rotating element of the hydraulic motor, typically consisting of a shaft and one or more gears, pistons, vanes, or rollers that interact with pressurized hydraulic fluid to generate rotational motion. The rotor is housed within a fixed stator and is connected to the driven equipment or load, transferring mechanical energy from the hydraulic motor to perform work.
- Stator: The stator is the stationary outer housing or casing that surrounds the rotor and provides support and containment for the internal components of the hydraulic motor. It contains inlet and outlet ports for hydraulic fluid and directs fluid flow to interact with the rotor to generate rotational motion. The stator may be made of cast iron, aluminum, or steel and is designed to withstand hydraulic pressure and loads.
- Internal Components: Hydraulic motors contain various internal components, such as gears, pistons, vanes, or rollers, that interact with pressurized hydraulic fluid to generate rotary motion. These components may operate on different principles, including gear motors, piston motors, vane motors, and roller motors, each with its advantages and limitations in terms of efficiency, speed, torque, and operating characteristics.
- Inlet and Outlet Ports: The inlet and outlet ports are openings or connections on the hydraulic motor through which pressurized hydraulic fluid enters and exits the motor. Hydraulic fluid is supplied to the motor through the inlet port, where it interacts with the internal components to generate rotational motion. Fluid exits the motor through the outlet port after transferring energy to the rotor.
- Seals and Bearings: Seals and bearings are used to prevent leakage of hydraulic fluid and provide support and alignment for the rotating components of the hydraulic motor. Seals prevent fluid from escaping between moving parts, while bearings reduce friction and wear on motor components. Proper sealing and lubrication are essential to ensure efficient operation and longevity of hydraulic motors.
Hydraulic motors are classified into several types based on their operating principle and construction, including:
- Gear Motors: Gear motors utilize meshing gears to transfer energy from pressurized hydraulic fluid to the rotor, generating rotational motion. They are compact, cost-effective, and suitable for low to moderate torque and speed applications.
- Piston Motors: Piston motors use reciprocating pistons to transfer energy from pressurized hydraulic fluid to the rotor, generating high torque and speed. They offer high efficiency, variable displacement capabilities, and precise control, making them suitable for high-performance applications.
- Vane Motors: Vane motors use sliding vanes or blades to transfer energy from pressurized hydraulic fluid to the rotor, generating smooth and continuous rotation. They offer low noise levels, moderate torque and speed capabilities, and good efficiency, making them suitable for a wide range of applications.
Proper selection, sizing, installation, and maintenance of hydraulic motors are essential to ensure optimal performance, reliability, and safety in hydraulic systems. Engineers and technicians must consider factors such as motor type, size, displacement, speed, torque, efficiency, and compatibility with hydraulic fluid when selecting hydraulic motors for specific applications. Regular inspection, lubrication, and preventive maintenance are necessary to detect signs of wear, leakage, or damage and prevent hydraulic motor failure and performance degradation.
Hydraulic Pump
A hydraulic pump is a mechanical device that converts mechanical energy into hydraulic energy by pressurizing hydraulic fluid, typically oil. It plays a crucial role in hydraulic systems by generating the flow and pressure required to power hydraulic machinery and equipment. Hydraulic pumps are widely used in various industrial, mobile, and marine applications to provide the force necessary for lifting, pushing, pulling, and moving heavy loads or materials.
Key components and characteristics of hydraulic pumps include:
- Pump Housing: The pump housing, also known as the pump casing or body, is the outer enclosure that contains the internal components of the hydraulic pump. It provides structural support, protection, and alignment for the pump components and is typically made of cast iron, aluminum, or steel to withstand hydraulic pressure and loads.
- Rotating Element: The rotating element of the hydraulic pump is responsible for generating hydraulic pressure and flow by moving hydraulic fluid from the pump inlet to the pump outlet. It may consist of gears, pistons, vanes, or screws that rotate within the pump housing to trap and pressurize hydraulic fluid. The rotating element may be driven by an external power source, such as an electric motor or an internal combustion engine, via a shaft or coupling.
- Inlet and Outlet Ports: The inlet and outlet ports are openings or connections on the hydraulic pump through which hydraulic fluid enters and exits the pump. Hydraulic fluid is drawn into the pump through the inlet port, where it is pressurized and discharged through the outlet port at a higher pressure and flow rate. Inlet and outlet ports may be threaded, flanged, or connected to hydraulic lines or hoses, depending on the pump design and installation requirements.
- Seals and Bearings: Seals and bearings are used to prevent leakage of hydraulic fluid and provide support and alignment for the rotating components of the hydraulic pump. Seals prevent fluid from escaping between moving parts, while bearings reduce friction and wear on pump components. Proper sealing and lubrication are essential to ensure efficient operation and longevity of hydraulic pumps.
- Control Mechanism: Some hydraulic pumps include a control mechanism to regulate flow rate, pressure, or direction of hydraulic fluid. Control mechanisms may include variable displacement mechanisms, such as swashplate, axial piston, or radial piston designs, that adjust pump output according to system demand. Control valves or regulators may also be incorporated into the pump design to provide additional control over pump operation.
Hydraulic pumps are classified into several types based on their operating principle and construction, including:
- Gear Pumps: Gear pumps utilize meshing gears to transfer hydraulic fluid from the pump inlet to the pump outlet, generating flow and pressure. They are simple in design, cost-effective, and suitable for low to moderate pressure and flow applications.
- Piston Pumps: Piston pumps use reciprocating pistons to transfer hydraulic fluid from the pump inlet to the pump outlet, generating high pressure and flow. They offer high efficiency, variable displacement capabilities, and precise control, making them suitable for high-performance applications.
- Vane Pumps: Vane pumps use sliding vanes or blades to transfer hydraulic fluid from the pump inlet to the pump outlet, generating smooth and continuous flow. They offer low noise levels, moderate pressure and flow capabilities, and good efficiency, making them suitable for a wide range of applications.
Proper selection, sizing, installation, and maintenance of hydraulic pumps are essential to ensure optimal performance, reliability, and safety in hydraulic systems. Engineers and technicians must consider factors such as pump type, size, displacement, speed, pressure, flow rate, efficiency, and compatibility with hydraulic fluid when selecting hydraulic pumps for specific applications. Regular inspection, lubrication, and preventive maintenance are necessary to detect signs of wear, leakage, or damage and prevent hydraulic pump failure and performance degradation.
Hydraulic Filter
A hydraulic filter is a device used to remove contaminants and impurities from hydraulic fluid, ensuring clean and reliable operation of hydraulic systems. It plays a critical role in maintaining the performance, efficiency, and longevity of hydraulic components by preventing abrasive particles, dirt, debris, and other contaminants from circulating and causing damage or wear.
Key components and characteristics of hydraulic filters include:
- Filter Element: The filter element is the heart of the hydraulic filter and is responsible for capturing and retaining contaminants from the hydraulic fluid. It may consist of a porous media, such as cellulose, paper, synthetic fibers, wire mesh, or metal screens, arranged in layers or pleats to maximize surface area and filtration efficiency. The filter element traps particles of various sizes as hydraulic fluid passes through, preventing them from circulating and causing damage to hydraulic components.
- Filter Housing: The filter housing, also known as the filter body or casing, encloses and supports the filter element and provides a sealed environment for filtration. It is typically made of metal or plastic and is designed to withstand hydraulic pressure, fluid flow, and environmental conditions. The filter housing may include inlet and outlet ports, mounting provisions, and connection points for hydraulic lines or hoses.
- Bypass Valve: Some hydraulic filters are equipped with a bypass valve or bypass indicator that activates when the filter becomes clogged or reaches its maximum filtration capacity. The bypass valve allows hydraulic fluid to bypass the filter element temporarily, ensuring uninterrupted flow to hydraulic components and preventing system damage due to restricted flow or pressure drop. Bypass indicators provide visual or audible alerts when the filter is bypassing fluid, indicating the need for filter replacement or maintenance.
- Micron Rating: The micron rating of a hydraulic filter refers to the size of particles it can effectively capture and retain during filtration. It is a measure of the filter’s efficiency in removing contaminants from hydraulic fluid and is typically expressed in microns (μm). Hydraulic filters are available in various micron ratings to suit different application requirements, with finer ratings capable of capturing smaller particles and providing higher levels of filtration.
- Filter Media: Hydraulic filters use different types of filter media, including depth media, surface media, and combination media, to achieve effective filtration of hydraulic fluid. Depth media filters rely on the depth of the media to trap particles throughout the thickness of the filter element, while surface media filters capture particles on the surface of the media. Combination media filters combine both depth and surface filtration mechanisms to achieve optimal filtration efficiency.
Hydraulic filters are classified into several types based on their construction, filtration mechanism, and application, including:
- Suction Strainers: Suction strainers are located at the inlet port of hydraulic pumps or reservoirs and prevent large particles and debris from entering the hydraulic system. They are typically made of wire mesh or perforated metal screens and provide coarse filtration to protect pump components from damage.
- Inline Filters: Inline filters are installed in hydraulic lines or hoses and provide fine filtration of hydraulic fluid as it flows through the system. They are available in various configurations, including cartridge filters, spin-on filters, and filter assemblies, and offer efficient removal of contaminants to protect hydraulic components from wear and damage.
- Return Line Filters: Return line filters are located in the return line of hydraulic systems and remove contaminants from hydraulic fluid returning to the reservoir. They are designed to handle high flow rates and provide continuous filtration to maintain clean hydraulic fluid and prevent contamination buildup in the system.
Proper selection, sizing, installation, and maintenance of hydraulic filters are essential to ensure optimal performance, reliability, and safety in hydraulic systems. Engineers and technicians must consider factors such as filter type, micron rating, flow capacity, pressure rating, compatibility with hydraulic fluid, and maintenance requirements when selecting hydraulic filters for specific applications. Regular inspection, replacement, and monitoring of hydraulic filters are necessary to maintain proper filtration efficiency, prevent contamination-related issues, and extend the service life of hydraulic components.
Hydraulic Reservoir
A hydraulic reservoir, also known as a hydraulic tank or hydraulic oil reservoir, is a storage container used to hold hydraulic fluid within a hydraulic system. It serves as a vital component for storing, cooling, filtering, and supplying hydraulic fluid to various hydraulic components, such as pumps, valves, cylinders, and motors, while also providing space for air separation and fluid expansion.
Key components and characteristics of hydraulic reservoirs include:
- Reservoir Body: The reservoir body is the main enclosure or container that holds hydraulic fluid and provides structural support and protection for the internal components of the reservoir. It is typically made of metal, such as steel or aluminum, or plastic, and is designed to withstand hydraulic pressure, fluid volume, and environmental conditions. The reservoir body may be cylindrical, rectangular, or custom-shaped, depending on space constraints and installation requirements.
- Fluid Level Indicator: A fluid level indicator is a device used to monitor the hydraulic fluid level inside the reservoir and provide visual or electrical feedback to operators or control systems. Common types of fluid level indicators include sight glasses, dipsticks, float switches, and level sensors, which indicate whether the fluid level is within the desired operating range and alert when fluid levels are low or high.
- Breather Filter: A breather filter is a component mounted on the top of the reservoir to allow air to enter and exit the reservoir while preventing contaminants, moisture, and airborne particles from entering the hydraulic system. Breather filters typically include a desiccant or silica gel to absorb moisture from incoming air and maintain hydraulic fluid cleanliness and integrity.
- Filtration System: Some hydraulic reservoirs include a filtration system or filter assembly to remove contaminants and impurities from hydraulic fluid as it enters or exits the reservoir. Filters may be located at the reservoir inlet or outlet ports and help maintain hydraulic fluid cleanliness, extend component life, and prevent system contamination.
- Cooling System: Hydraulic reservoirs may incorporate a cooling system or heat exchanger to dissipate heat generated during hydraulic system operation and maintain hydraulic fluid within the desired temperature range. Cooling systems may include air-cooled or water-cooled heat exchangers, fans, radiators, or heat sinks that transfer heat from the hydraulic fluid to the surrounding environment.
- Fluid Sight Glass: A fluid sight glass is a transparent window or viewport mounted on the side of the reservoir to allow visual inspection of hydraulic fluid condition, level, and cleanliness. Sight glasses provide operators and maintenance personnel with a clear view of the hydraulic fluid inside the reservoir and help detect signs of contamination, foaming, or discoloration.
Hydraulic reservoirs are available in various sizes, shapes, capacities, and configurations to suit different hydraulic system requirements and installation constraints. They may be mounted vertically, horizontally, or custom-configured to fit specific equipment layouts and space limitations. Proper sizing, installation, and maintenance of hydraulic reservoirs are essential to ensure optimal performance, reliability, and safety in hydraulic systems. Engineers and technicians must consider factors such as reservoir capacity, fluid volume, operating temperature, fluid level control, filtration requirements, and cooling needs when selecting hydraulic reservoirs for specific applications. Regular inspection, cleaning, and maintenance of hydraulic reservoirs and associated components are necessary to maintain proper fluid levels, cleanliness, and temperature control and prevent contamination, overheating, or system failures.
Hydraulic Hose
A hydraulic hose is a flexible tube used to convey hydraulic fluid from one component to another within a hydraulic system. It serves as a vital link for transmitting pressurized hydraulic fluid between hydraulic pumps, valves, cylinders, motors, and other hydraulic components, enabling the operation of machinery and equipment in various industrial, mobile, and marine applications.
Key components and characteristics of hydraulic hoses include:
- Inner Tube: The inner tube is the innermost layer of the hydraulic hose and is in direct contact with the hydraulic fluid. It is typically made of synthetic rubber or thermoplastic material resistant to hydraulic oil, abrasion, and chemical exposure. The inner tube provides a barrier between the hydraulic fluid and other layers of the hose and prevents fluid leakage or absorption.
- Reinforcement: The reinforcement layer(s) of the hydraulic hose provide structural strength and support to withstand internal pressure, external forces, and bending or flexing during operation. Common reinforcement materials include braided steel wire, spiral-wound steel wire, textile fibers, or synthetic yarns, which are embedded or woven into the hose construction to increase strength and flexibility.
- Cover: The cover is the outermost layer of the hydraulic hose and protects the inner tube and reinforcement layers from environmental factors, abrasion, UV radiation, and mechanical damage. It is typically made of synthetic rubber, thermoplastic, or elastomeric material resistant to oil, weathering, ozone, and abrasion. The cover may also include additional features such as color-coded markings, branding, or protective sleeves for identification and added durability.
- End Fittings: End fittings, also known as hydraulic couplings or connectors, are attached to the ends of the hydraulic hose to facilitate connection to hydraulic components and equipment. They provide a leak-proof seal and secure attachment to hydraulic ports, pumps, valves, cylinders, or motors and may be crimped, swaged, or threaded onto the hose ends. End fittings are available in various types, sizes, and configurations, including straight, elbow, tee, flange, quick-connect, and reusable fittings, to suit different installation requirements and system designs.
- Pressure Rating: The pressure rating of a hydraulic hose refers to the maximum pressure it can safely withstand while conveying hydraulic fluid. It is determined by factors such as hose construction, reinforcement type, material strength, and design standards and is typically expressed in pounds per square inch (psi) or bar. Hydraulic hoses are available in various pressure ratings to suit different application requirements, ranging from low-pressure to high-pressure applications.
- Temperature Range: The temperature range of a hydraulic hose refers to the range of temperatures at which it can safely operate without degradation or failure. It is determined by factors such as hose material, reinforcement type, and fluid compatibility and is typically specified by the manufacturer. Hydraulic hoses are designed to withstand a wide range of temperatures, from extreme cold to high heat, depending on the application and environmental conditions.
Hydraulic hoses are classified into several types based on their construction, reinforcement, and application, including:
- Wire Braided Hoses: Wire braided hoses feature one or more layers of braided steel wire reinforcement for moderate to high-pressure applications. They offer flexibility, durability, and resistance to abrasion and are suitable for general-purpose hydraulic systems.
- Wire Spiral Hoses: Wire spiral hoses feature multiple layers of spiral-wound steel wire reinforcement for high-pressure applications. They offer superior strength, stability, and resistance to flexing and are commonly used in heavy-duty hydraulic systems and equipment.
- Thermoplastic Hoses: Thermoplastic hoses are made of synthetic thermoplastic materials such as nylon, polyethylene, or polyurethane and are reinforced with textile fibers or braided steel wire for moderate-pressure applications. They offer flexibility, lightweight, and resistance to abrasion, chemicals, and UV radiation, making them suitable for mobile and marine hydraulic systems.
Proper selection, installation, and maintenance of hydraulic hoses are essential to ensure optimal performance, reliability, and safety in hydraulic systems. Engineers and technicians must consider factors such as hose type, size, length, pressure rating, temperature range, fluid compatibility, end fittings, and installation requirements when selecting hydraulic hoses for specific applications. Regular inspection, testing, and replacement of hydraulic hoses are necessary to detect signs of wear, leakage, or damage and prevent hydraulic system failures and safety hazards.
Hydraulic Actuator
A hydraulic actuator is a mechanical device used to convert hydraulic energy into mechanical motion or force. It plays a crucial role in hydraulic systems by translating the pressure and flow of hydraulic fluid into linear or rotary motion to perform various tasks, such as lifting, pushing, pulling, rotating, or controlling the movement of loads or equipment.
Key components and characteristics of hydraulic actuators include:
- Cylinder: In a hydraulic actuator, the cylinder is the main housing or enclosure that contains the moving components, such as pistons, rods, or plungers, and provides support and guidance for their motion. It is typically made of high-strength materials, such as steel or aluminum, and is designed to withstand hydraulic pressure, fluid flow, and mechanical loads. The cylinder may be single-acting, with hydraulic pressure acting on one side only, or double-acting, with hydraulic pressure acting on both sides for bi-directional movement.
- Piston: The piston is a cylindrical or disc-shaped component that divides the cylinder into two chambers: the rod side and the cap side. It is fitted with seals to prevent leakage of hydraulic fluid and transmits hydraulic pressure from one chamber to the other to generate linear motion. The piston may be single-acting, with hydraulic pressure acting on one side only, or double-acting, with hydraulic pressure acting on both sides for bi-directional movement.
- Rod: The rod is a cylindrical shaft that extends from the piston through a sealed opening in the cylinder and provides the connection point for external loads or equipment. It transmits the force generated by the hydraulic pressure to perform work and may be equipped with end fittings, clevises, or eye brackets for mounting attachments. The rod is typically made of hardened and chrome-plated steel to resist wear, corrosion, and damage.
- Seals: Seals are used to prevent leakage of hydraulic fluid and maintain pressure and integrity within the hydraulic actuator. They are located at various points along the cylinder, piston, and rod and are exposed to hydraulic fluid and high pressures during operation. Common types of seals used in hydraulic actuators include O-rings, lip seals, piston seals, and rod seals, which are selected based on factors such as temperature, pressure, fluid compatibility, and application requirements.
- Mounting Provisions: Hydraulic actuators may include mounting provisions or attachment points for securing the actuator to external structures, frames, or equipment. Mounting provisions may include flanges, clevises, eye brackets, or trunnion mounts that allow for easy installation and alignment of the actuator in various orientations. Proper mounting and alignment are essential to ensure smooth operation and performance of hydraulic actuators.
Hydraulic actuators are classified into several types based on their operating principle, construction, and application, including:
- Hydraulic Cylinders: Hydraulic cylinders use the linear motion of pistons and rods to perform lifting, pushing, pulling, or positioning tasks. They are commonly used in applications such as material handling, construction equipment, agricultural machinery, and industrial automation.
- Hydraulic Motors: Hydraulic motors use the rotary motion of gears, pistons, vanes, or rollers to perform rotational tasks such as driving conveyors, winches, cranes, and rotary actuators. They are commonly used in applications requiring continuous rotation or variable speed control.
- Hydraulic Rotary Actuators: Hydraulic rotary actuators convert hydraulic pressure into rotary motion to control the position or movement of rotary components such as valves, dampers, gates, or shafts. They are commonly used in industrial, marine, and aerospace applications requiring precise positioning or torque control.
Proper selection, sizing, installation, and maintenance of hydraulic actuators are essential to ensure optimal performance, reliability, and safety in hydraulic systems. Engineers and technicians must consider factors such as actuator type, size, stroke length, bore diameter, rod diameter, operating pressure, mounting configuration, and application requirements when selecting hydraulic actuators for specific tasks. Regular inspection, lubrication, and preventive maintenance are necessary to detect signs of wear, leakage, or damage and prevent hydraulic actuator failure and performance degradation.
Hydraulic Valve
A hydraulic valve is a mechanical device used to control the flow, pressure, and direction of hydraulic fluid within a hydraulic system. It plays a critical role in regulating the operation of hydraulic components such as pumps, cylinders, motors, and actuators, allowing for precise control and manipulation of fluid power to perform various tasks in industrial, mobile, and marine applications.
Key components and characteristics of hydraulic valves include:
- Valve Body: The valve body is the main housing or enclosure that contains the internal components of the hydraulic valve and provides structural support and protection. It is typically made of metal, such as steel or aluminum, and is designed to withstand hydraulic pressure, fluid flow, and mechanical loads. The valve body may include inlet and outlet ports, mounting provisions, and connection points for hydraulic lines or hoses.
- Internal Components: Hydraulic valves contain various internal components, including spools, poppets, discs, balls, or plungers, that control the flow, pressure, and direction of hydraulic fluid. These components are arranged within the valve body and interact with each other to open, close, or modulate fluid flow in response to external signals or commands. Internal components may be actuated mechanically, electrically, pneumatically, or hydraulically, depending on the valve type and application.
- Actuation Mechanism: The actuation mechanism of a hydraulic valve determines how it is operated or controlled to change its position or configuration. Hydraulic valves may be actuated manually, using hand levers, knobs, or handles, or automatically, using solenoids, electric motors, pneumatic actuators, or hydraulic actuators. Actuation mechanisms may be simple on-off devices or complex proportional or servo-controlled systems that provide precise and variable control over fluid flow, pressure, and direction.
- Valve Types: Hydraulic valves are available in various types and configurations to suit different application requirements and operating conditions. Common types of hydraulic valves include:
- Directional Control Valves: Directional control valves regulate the flow of hydraulic fluid to control the direction of movement of hydraulic actuators, such as cylinders or motors. They include spool valves, poppet valves, and rotary valves, with configurations such as 2-way, 3-way, 4-way, and 5-way valves to control single or multiple hydraulic circuits.
- Pressure Control Valves: Pressure control valves regulate the pressure of hydraulic fluid within a hydraulic system to prevent overloading, overpressure, or damage to system components. They include relief valves, pressure-reducing valves, sequence valves, and pressure-compensated valves, which maintain hydraulic pressure within safe and desired limits.
- Flow Control Valves: Flow control valves regulate the rate of flow of hydraulic fluid within a hydraulic system to control the speed, force, or timing of hydraulic actuators. They include throttle valves, needle valves, check valves, and flow regulators, which adjust fluid flow rates based on system demand and load conditions.
- Proportional and Servo Valves: Proportional and servo valves provide precise and variable control over fluid flow, pressure, and direction in hydraulic systems. They use electronic or hydraulic signals to modulate the position or movement of internal components, allowing for fine-tuning and optimization of system performance.
Proper selection, sizing, installation, and maintenance of hydraulic valves are essential to ensure optimal performance, reliability, and safety in hydraulic systems. Engineers and technicians must consider factors such as valve type, size, flow capacity, pressure rating, actuation mechanism, control method, compatibility with hydraulic fluid, and application requirements when selecting hydraulic valves for specific tasks. Regular inspection, testing, and maintenance of hydraulic valves are necessary to detect signs of wear, leakage, or malfunction and prevent hydraulic system failures and safety hazards.
Hydraulic Accumulator
A hydraulic accumulator is a device used to store hydraulic energy in the form of pressurized fluid, providing a temporary reservoir of hydraulic power for use in hydraulic systems. It plays a crucial role in maintaining system pressure, damping pressure fluctuations, absorbing shocks, and supplementing pump flow during peak demand periods, enhancing the performance, efficiency, and reliability of hydraulic equipment.
Key components and characteristics of hydraulic accumulators include:
- Pressure Vessel: The pressure vessel is the main enclosure or container that holds hydraulic fluid and provides a sealed environment for pressurization. It is typically made of high-strength materials such as steel, aluminum, or composite materials and is designed to withstand high pressures and loads. The pressure vessel may be cylindrical, spherical, or bladder-shaped, depending on the accumulator type and application requirements.
- Gas Chamber: The gas chamber is an enclosed space within the pressure vessel that contains a compressible gas, such as nitrogen or air. It acts as a cushion or spring, absorbing hydraulic fluid under pressure and providing the force necessary to maintain system pressure during periods of low demand or pump downtime. The gas chamber may be separated from the hydraulic fluid by a flexible bladder, piston, or diaphragm to prevent mixing and ensure gas purity.
- Fluid Connection Ports: Hydraulic accumulators include fluid connection ports or fittings for connecting to hydraulic lines or circuits within the system. These ports allow hydraulic fluid to enter and exit the accumulator during charging and discharging cycles, facilitating energy storage and release as needed. Fluid connection ports may be threaded, flanged, or welded onto the pressure vessel, depending on the accumulator design and installation requirements.
- Gas Charging Valve: The gas charging valve is a port or fitting used to introduce or remove gas from the gas chamber to adjust accumulator pressure. It allows for periodic maintenance, inspection, and recharging of the accumulator with the appropriate gas to maintain desired pressure levels. Gas charging valves may include manual or automatic valves, pressure gauges, and safety devices to ensure proper operation and prevent overcharging or overpressure conditions.
- Safety Devices: Hydraulic accumulators may include safety devices or features to prevent overpressure, overcharging, or leakage and ensure safe operation within the hydraulic system. Common safety devices include pressure relief valves, rupture discs, burst plugs, and pressure sensors that monitor and control accumulator pressure and activate protective measures if pressure exceeds safe limits.
Hydraulic accumulators are classified into several types based on their operating principle, construction, and application, including:
- Bladder Accumulators: Bladder accumulators use a flexible elastomeric bladder to separate hydraulic fluid from the gas chamber, allowing for efficient energy storage and release. They offer high energy density, fast response times, and minimal gas absorption into hydraulic fluid, making them suitable for dynamic applications such as mobile equipment, machine tools, and industrial machinery.
- Piston Accumulators: Piston accumulators use a movable piston to separate hydraulic fluid from the gas chamber, providing reliable energy storage and release. They offer high pressure and flow capacity, low gas permeation, and long service life, making them suitable for heavy-duty applications such as hydraulic presses, cranes, and marine systems.
- Diaphragm Accumulators: Diaphragm accumulators use a flexible diaphragm to separate hydraulic fluid from the gas chamber, providing compact energy storage and release. They offer high efficiency, low gas permeation, and compatibility with a wide range of fluids, making them suitable for aerospace, automotive, and process industry applications.
Proper selection, sizing, installation, and maintenance of hydraulic accumulators are essential to ensure optimal performance, reliability, and safety in hydraulic systems. Engineers and technicians must consider factors such as accumulator type, size, pressure rating, gas precharge, fluid volume, operating temperature, and application requirements when selecting hydraulic accumulators for specific tasks. Regular inspection, testing, and maintenance of hydraulic accumulators are necessary to detect signs of wear, leakage, or malfunction and prevent hydraulic system failures and safety hazards.
Hydraulic Filter Element
The hydraulic filter element is a crucial component within hydraulic filtration systems, responsible for capturing and retaining contaminants and impurities from hydraulic fluid to maintain system cleanliness and performance. It serves as the primary barrier against particles, debris, and other contaminants that can cause wear, damage, and malfunction in hydraulic components.
Key features and characteristics of hydraulic filter elements include:
- Filtration Media: The filtration media is the material within the filter element that captures and retains contaminants from the hydraulic fluid. Common types of filtration media include cellulose, paper, synthetic fibers, wire mesh, metal screens, and microglass fibers, each offering different levels of filtration efficiency, dirt-holding capacity, and fluid compatibility.
- Micron Rating: The micron rating of a hydraulic filter element refers to the size of particles it can effectively capture and retain during filtration. It is a measure of the filter’s efficiency in removing contaminants from hydraulic fluid and is typically expressed in microns (μm). Hydraulic filter elements are available in various micron ratings to suit different application requirements, with finer ratings capable of capturing smaller particles.
- Construction: Hydraulic filter elements are typically constructed as cylindrical or pleated cartridges, with the filtration media enclosed within a rigid or flexible frame. The construction design influences factors such as surface area, flow rate, dirt-holding capacity, and compatibility with different types of filter housings and systems.
- End Caps: The end caps of a hydraulic filter element provide structural support and sealing to ensure proper fit and alignment within the filter housing. They may be made of metal, plastic, or rubber and are designed to withstand hydraulic pressure, fluid flow, and mechanical loads without deformation or leakage.
- Seals: Seals are used to prevent leakage of hydraulic fluid and ensure a tight seal between the filter element and the filter housing. They may be made of elastomeric materials such as Buna-N, Viton, or EPDM and are positioned at the end caps or interface points of the filter element to maintain integrity and efficiency.
- Compatibility: Hydraulic filter elements must be compatible with the hydraulic fluid used in the system to ensure proper filtration performance and system reliability. Factors such as fluid type, viscosity, temperature, pressure, and chemical additives must be considered when selecting filter elements to prevent degradation, swelling, or failure.
Hydraulic filter elements are available in various configurations, sizes, shapes, and materials to suit different filter housings, system designs, and operating conditions. Proper selection, installation, and maintenance of hydraulic filter elements are essential to ensure optimal filtration performance, system cleanliness, and component longevity in hydraulic systems. Regular inspection, replacement, and monitoring of filter elements are necessary to maintain proper filtration efficiency, prevent contamination-related issues, and extend the service life of hydraulic components.
Hydraulic Fluid
Hydraulic fluid is a specialized liquid medium used to transmit power in hydraulic systems, where it serves as the primary means of transferring force from one component to another. It plays a critical role in hydraulic systems by transmitting energy, lubricating moving parts, dissipating heat, sealing hydraulic components, and protecting against corrosion and wear.
Key characteristics and properties of hydraulic fluids include:
- Viscosity: Viscosity refers to the resistance of a fluid to flow and is a critical property of hydraulic fluids. The viscosity of hydraulic fluid affects its ability to flow through hydraulic components, such as pumps, valves, cylinders, and motors, and influences system efficiency, performance, and response. Hydraulic fluids are available in different viscosity grades, ranging from low-viscosity fluids for high-speed applications to high-viscosity fluids for high-pressure applications.
- Viscosity Index: The viscosity index (VI) is a measure of how a hydraulic fluid’s viscosity changes with temperature variations. A higher VI indicates that the fluid’s viscosity changes less with temperature, providing better lubrication and protection over a wider temperature range. Hydraulic fluids with a high VI are preferred for applications where temperature fluctuations are significant.
- Fluid Base: Hydraulic fluids may be formulated using different base stocks, including mineral oils, synthetic oils, and water-based fluids. Mineral oil-based hydraulic fluids are the most common and offer good lubrication, stability, and compatibility with hydraulic system components. Synthetic hydraulic fluids offer improved performance in extreme temperatures, higher pressures, and harsh operating conditions. Water-based hydraulic fluids are environmentally friendly and used in applications where fire resistance, biodegradability, or non-toxicity are required.
- Additives: Additives are chemical compounds added to hydraulic fluids to enhance their performance, stability, and properties. Common additives include anti-wear agents, anti-foam agents, corrosion inhibitors, oxidation stabilizers, viscosity modifiers, and friction modifiers. Additives help improve lubrication, reduce wear, prevent oxidation, control foam formation, and extend the service life of hydraulic components.
- Fire Resistance: Fire resistance is an important property of hydraulic fluids, especially in applications where fire hazards are present, such as in steel mills, foundries, and mining operations. Fire-resistant hydraulic fluids are formulated to withstand high temperatures without igniting or sustaining combustion, reducing the risk of fire and minimizing damage to equipment and personnel.
- Biodegradability: Biodegradability refers to the ability of hydraulic fluids to break down into harmless substances by natural biological processes, such as microbial action. Environmentally friendly hydraulic fluids are formulated with biodegradable base stocks and additives to minimize environmental impact and comply with regulations governing spills, leaks, and disposal.
- Compatibility: Hydraulic fluids must be compatible with system components such as seals, hoses, valves, pumps, and cylinders to ensure proper operation and longevity. Compatibility issues can lead to seal swelling, material degradation, leakage, and reduced performance. It is essential to select hydraulic fluids that are compatible with the materials used in the hydraulic system and to avoid mixing different types of fluids.
Hydraulic fluids are selected based on factors such as system requirements, operating conditions, temperature range, pressure range, environmental considerations, and regulatory compliance. Proper handling, storage, and maintenance of hydraulic fluids are essential to ensure system reliability, performance, and safety. Regular fluid analysis, filtration, and replacement are necessary to maintain proper fluid cleanliness, viscosity, and performance and prevent contamination-related issues in hydraulic systems.
Hydraulic Pump
A hydraulic pump is a mechanical device used to generate flow and create pressure within a hydraulic system, enabling the transmission of hydraulic power to perform work. It serves as the heart of a hydraulic system by converting mechanical energy, typically from an electric motor or an internal combustion engine, into hydraulic energy in the form of fluid flow and pressure.
Key components and characteristics of hydraulic pumps include:
- Pump Types: Hydraulic pumps are available in various types, each with unique operating principles, performance characteristics, and applications. Common types of hydraulic pumps include:
- Gear Pumps: Gear pumps use intermeshing gears to create flow and generate pressure by trapping and displacing hydraulic fluid between the gear teeth. They are simple in design, cost-effective, and suitable for low to medium-pressure applications.
- Vane Pumps: Vane pumps use sliding vanes or blades to create flow and generate pressure by eccentrically rotating within a cylindrical chamber. They offer smooth and quiet operation, good efficiency, and moderate pressure capability.
- Piston Pumps: Piston pumps use reciprocating pistons or plungers to create flow and generate pressure by alternately drawing in and displacing hydraulic fluid within cylinder bores. They offer high efficiency, high pressure capability, and variable displacement options for precise control and performance.
- Displacement: Displacement refers to the volume of hydraulic fluid displaced by the pump per revolution or stroke and is typically measured in cubic inches or cubic centimeters. Hydraulic pumps may be fixed displacement, where the output flow rate is constant, or variable displacement, where the output flow rate can be adjusted to match system demand.
- Pressure Rating: The pressure rating of a hydraulic pump refers to the maximum pressure it can generate within the hydraulic system. It is typically measured in pounds per square inch (psi) or bar and is determined by factors such as pump design, construction, materials, and operating conditions. Hydraulic pumps are available in various pressure ratings to suit different application requirements, ranging from low-pressure to high-pressure systems.
- Flow Rate: The flow rate of a hydraulic pump refers to the volume of hydraulic fluid delivered by the pump per unit of time and is typically measured in gallons per minute (gpm) or liters per minute (lpm). The flow rate of a hydraulic pump depends on factors such as pump displacement, rotational speed, and operating pressure, and it determines the system’s ability to perform work and meet operational requirements.
- Drive Shaft: The drive shaft is the component of the hydraulic pump that connects to the prime mover, such as an electric motor or an internal combustion engine, to transmit mechanical power to the pump. It may be splined, keyed, or threaded to ensure proper alignment and secure attachment between the pump and the prime mover.
- Mounting Configuration: Hydraulic pumps may have different mounting configurations to accommodate various installation requirements and space constraints. Common mounting configurations include flange mounting, foot mounting, vertical mounting, and horizontal mounting, which allow for flexible integration into hydraulic systems and equipment.
Hydraulic pumps are selected based on factors such as system requirements, flow rate, pressure rating, displacement, speed, efficiency, and application considerations. Proper installation, operation, and maintenance of hydraulic pumps are essential to ensure optimal performance, reliability, and longevity in hydraulic systems. Regular inspection, lubrication, and preventive maintenance are necessary to detect signs of wear, leakage, or malfunction and prevent hydraulic pump failure and system downtime.
Hydraulic Motor
A hydraulic motor is a mechanical device used to convert hydraulic energy into rotational mechanical energy, providing power to drive machinery and equipment in hydraulic systems. It serves as the counterpart to hydraulic pumps, utilizing pressurized hydraulic fluid to generate rotary motion and perform work.
Key components and characteristics of hydraulic motors include:
- Motor Types: Hydraulic motors are available in various types, each with unique operating principles, performance characteristics, and applications. Common types of hydraulic motors include:
- Gear Motors: Gear motors use intermeshing gears to convert hydraulic pressure into rotational motion. They are simple in design, cost-effective, and suitable for low to medium-speed applications.
- Vane Motors: Vane motors use sliding vanes or blades to convert hydraulic pressure into rotational motion by eccentrically rotating within a cylindrical chamber. They offer smooth operation, good efficiency, and moderate speed capability.
- Piston Motors: Piston motors use reciprocating pistons or plungers to convert hydraulic pressure into rotational motion by alternately pushing against a swash plate or cam within the motor housing. They offer high efficiency, high torque capability, and variable displacement options for precise control and performance.
- Displacement: Displacement refers to the volume of hydraulic fluid displaced by the motor per revolution and is typically measured in cubic inches or cubic centimeters. Hydraulic motors may be fixed displacement, where the output speed is constant, or variable displacement, where the output speed can be adjusted to match system demand.
- Torque Output: Torque output refers to the rotational force generated by the hydraulic motor and is typically measured in pound-feet (lb-ft) or Newton-meters (Nm). The torque output of a hydraulic motor depends on factors such as motor displacement, pressure, and efficiency and determines the motor’s ability to drive loads and perform work.
- Speed: The speed of a hydraulic motor refers to the rotational speed at which it operates and is typically measured in revolutions per minute (rpm). The speed of a hydraulic motor depends on factors such as motor displacement, pressure, flow rate, and load conditions and determines the motor’s ability to drive machinery and equipment at the desired speed.
- Mounting Configuration: Hydraulic motors may have different mounting configurations to accommodate various installation requirements and space constraints. Common mounting configurations include flange mounting, foot mounting, shaft mounting, and face mounting, which allow for flexible integration into hydraulic systems and equipment.
- Seals and Bearings: Hydraulic motors include seals and bearings to prevent leakage of hydraulic fluid and ensure smooth operation and longevity. Seals are used to contain hydraulic fluid within the motor housing and prevent contamination from entering, while bearings support and guide rotating components to minimize friction and wear.
Hydraulic motors are selected based on factors such as system requirements, torque output, speed, displacement, efficiency, and application considerations. Proper installation, operation, and maintenance of hydraulic motors are essential to ensure optimal performance, reliability, and longevity in hydraulic systems. Regular inspection, lubrication, and preventive maintenance are necessary to detect signs of wear, leakage, or malfunction and prevent hydraulic motor failure and system downtime.
Hydraulic Reservoir
A hydraulic reservoir, also known as a hydraulic tank or hydraulic oil reservoir, is a container or vessel used to store hydraulic fluid in a hydraulic system. It serves as a vital component by providing several functions essential for the proper operation and maintenance of hydraulic systems.
Key components and characteristics of hydraulic reservoirs include:
- Fluid Storage: The primary function of a hydraulic reservoir is to store hydraulic fluid for use in the hydraulic system. The reservoir holds an adequate volume of hydraulic fluid to meet the system’s operational requirements, ensuring a constant and reliable supply of fluid for transmission of power and lubrication of components.
- Fluid Cooling: Hydraulic reservoirs often incorporate cooling mechanisms or features to dissipate heat generated during system operation. Excessive heat can degrade hydraulic fluid and reduce system performance and efficiency. Cooling methods may include the use of cooling fins, heat exchangers, fans, or circulating coolants to maintain optimal operating temperatures.
- Air Separation: Hydraulic reservoirs may include provisions for separating air or gases from the hydraulic fluid to prevent aeration or cavitation within the system. Aeration occurs when air bubbles are introduced into the hydraulic fluid, leading to decreased performance, increased noise, and potential damage to components. Air separation devices such as baffles, diffusers, or air vents help maintain fluid purity and system integrity.
- Fluid Filtration: Hydraulic reservoirs often incorporate filtration systems or elements to remove contaminants, debris, and impurities from the hydraulic fluid. Clean fluid is essential for maintaining system reliability, performance, and longevity. Filtration systems may include suction strainers, in-tank filters, or offline filtration units to ensure proper fluid cleanliness and protect system components from damage.
- Fluid Level Monitoring: Hydraulic reservoirs may include sight gauges, dipsticks, level switches, or electronic sensors to monitor fluid levels and ensure proper fluid management within the system. Monitoring fluid levels helps prevent overfilling or underfilling of the reservoir, which can lead to operational issues and damage to components.
- Fluid Breathing: Hydraulic reservoirs must allow for the expansion and contraction of hydraulic fluid due to temperature changes and system operation. Breather caps, vents, or expansion chambers provide a means for the reservoir to “breathe” and accommodate fluid volume changes without creating pressure build-up or vacuum conditions within the system.
- Construction and Material: Hydraulic reservoirs are typically constructed from materials such as steel, aluminum, or plastic, chosen for their strength, durability, and resistance to corrosion and environmental conditions. Reservoirs may be fabricated as integral components of hydraulic equipment or as standalone units mounted separately within the system.
Hydraulic reservoirs are designed and selected based on factors such as system requirements, fluid volume, temperature range, pressure rating, space constraints, and environmental considerations. Proper installation, maintenance, and inspection of hydraulic reservoirs are essential to ensure optimal system performance, fluid cleanliness, and component longevity. Regular monitoring of fluid levels, temperature, and cleanliness, along with periodic servicing and fluid analysis, help detect and prevent issues that could lead to system failure or downtime.
Hydraulic Cylinder
A hydraulic cylinder is a mechanical actuator used to produce linear motion and force by converting hydraulic energy into mechanical energy. It plays a crucial role in various applications across industries, including construction, manufacturing, agriculture, and aerospace, where precise and powerful linear motion is required.
Key components and characteristics of hydraulic cylinders include:
- Cylinder Barrel: The cylinder barrel is the main cylindrical housing that contains the moving components of the hydraulic cylinder, such as the piston, rod, and seals. It is typically made of high-strength materials such as steel or aluminum and is designed to withstand hydraulic pressure and mechanical loads.
- Piston: The piston is a cylindrical or disc-shaped component that divides the cylinder into two chambers: the rod side and the cap side. It is fitted with seals to prevent leakage of hydraulic fluid and transmits hydraulic pressure from one chamber to the other to generate linear motion. The piston may be single-acting, with hydraulic pressure acting on one side only, or double-acting, with hydraulic pressure acting on both sides for bi-directional movement.
- Rod: The rod is a cylindrical shaft that extends from the piston through a sealed opening in the cylinder and provides the connection point for external loads or equipment. It transmits the force generated by the hydraulic pressure to perform work and may be equipped with end fittings, clevises, or eye brackets for mounting attachments. The rod is typically made of hardened and chrome-plated steel to resist wear, corrosion, and damage.
- Seals: Seals are used to prevent leakage of hydraulic fluid and maintain pressure and integrity within the hydraulic cylinder. They are located at various points along the cylinder, piston, and rod and are exposed to hydraulic fluid and high pressures during operation. Common types of seals used in hydraulic cylinders include O-rings, lip seals, piston seals, and rod seals, which are selected based on factors such as temperature, pressure, fluid compatibility, and application requirements.
- Mounting Provisions: Hydraulic cylinders may include mounting provisions or attachment points for securing the cylinder to external structures, frames, or equipment. Mounting provisions may include flanges, clevises, eye brackets, or trunnion mounts that allow for easy installation and alignment of the cylinder in various orientations. Proper mounting and alignment are essential to ensure smooth operation and performance of hydraulic cylinders.
Hydraulic cylinders are classified into several types based on their construction, operating principle, and application, including:
- Single-Acting Cylinders: Single-acting cylinders have hydraulic pressure applied to one side of the piston, generating linear motion in one direction only. The return stroke is typically accomplished by a spring or external force.
- Double-Acting Cylinders: Double-acting cylinders have hydraulic pressure applied to both sides of the piston, allowing for bi-directional linear motion. Hydraulic fluid is alternately supplied and exhausted from the cylinder to extend and retract the piston.
- Telescopic Cylinders: Telescopic cylinders consist of multiple nested stages that extend and retract in a telescoping fashion, providing long stroke lengths in a compact package. They are commonly used in applications with limited space or long travel requirements, such as dump trucks, cranes, and aerial platforms.
Proper selection, sizing, installation, and maintenance of hydraulic cylinders are essential to ensure optimal performance, reliability, and safety in hydraulic systems. Engineers and technicians must consider factors such as cylinder type, size, stroke length, bore diameter, rod diameter, operating pressure, mounting configuration, and application requirements when selecting hydraulic cylinders for specific tasks. Regular inspection, lubrication, and preventive maintenance are necessary to detect signs of wear, leakage, or damage and prevent hydraulic cylinder failure and performance degradation.
Hydraulic Hose
A hydraulic hose is a flexible conduit used to transmit hydraulic fluid from one component to another within a hydraulic system. It serves as a vital link in hydraulic systems, facilitating the transfer of pressurized fluid to actuate cylinders, motors, valves, and other hydraulic components.
Key components and characteristics of hydraulic hoses include:
- Inner Tube: The inner tube is the innermost layer of the hydraulic hose and comes into direct contact with the hydraulic fluid. It is typically made of synthetic rubber or thermoplastic materials that are resistant to the type of hydraulic fluid being used and compatible with the operating pressure and temperature range of the system. The inner tube provides a barrier against fluid leakage and erosion caused by hydraulic pressure and fluid abrasion.
- Reinforcement: The reinforcement layer(s) provide structural support and reinforcement to the hydraulic hose, allowing it to withstand high pressures and mechanical loads without bursting or collapsing. Common reinforcement materials include high-tensile steel wire, textile fibers, or synthetic yarns arranged in braided, spiral, or helical patterns. The reinforcement layer(s) enhance the hose’s strength, flexibility, and resistance to kinking, twisting, and fatigue.
- Cover: The cover is the outermost layer of the hydraulic hose and serves as a protective barrier against external environmental conditions, abrasion, impact, UV radiation, and chemical exposure. It is typically made of synthetic rubber, thermoplastic, or elastomeric materials that are resistant to oil, weathering, ozone, and abrasion. The cover may be smooth, wrapped, or corrugated, depending on the application requirements and operating conditions.
- Fittings: Fittings are end connectors or couplings attached to the hydraulic hose to facilitate connection to hydraulic components such as pumps, cylinders, valves, and reservoirs. They provide a secure and leak-free interface between the hose and the component, ensuring proper fluid transfer and system integrity. Common types of hose fittings include threaded connectors, flanges, quick couplings, and crimped or reusable fittings, which are selected based on factors such as hose size, pressure rating, and connection method.
- Size and Length: Hydraulic hoses are available in various sizes and lengths to suit different system requirements, flow rates, pressure ratings, and installation configurations. The size of a hydraulic hose refers to its inside diameter (ID), while the length refers to the distance between the end fittings. Proper selection of hose size and length is essential to ensure optimal fluid flow, system performance, and compatibility with hydraulic components.
- Pressure Rating: The pressure rating of a hydraulic hose refers to the maximum pressure it can withstand within the operating temperature range specified by the manufacturer. It is typically measured in pounds per square inch (psi) or bar and is determined by factors such as hose construction, reinforcement type, and materials. Hydraulic hoses are available in various pressure ratings, ranging from low-pressure to high-pressure applications.
Hydraulic hoses are selected based on factors such as system requirements, operating pressure, temperature range, fluid compatibility, environmental conditions, and application considerations. Proper installation, routing, and maintenance of hydraulic hoses are essential to ensure optimal performance, reliability, and safety in hydraulic systems. Regular inspection, testing, and replacement of hoses are necessary to detect signs of wear, leakage, or damage and prevent hydraulic system failures and safety hazards.
Hydraulic Filtration System
A hydraulic filtration system is an essential component within hydraulic systems, designed to remove contaminants and impurities from hydraulic fluid to maintain system cleanliness, reliability, and performance. It plays a critical role in preventing wear, damage, and malfunction of hydraulic components by ensuring that the hydraulic fluid meets specified cleanliness standards.
Key components and characteristics of hydraulic filtration systems include:
- Filter Elements: Filter elements are the primary components responsible for capturing and retaining contaminants from the hydraulic fluid. They consist of porous media, such as paper, cellulose, synthetic fibers, or microglass, arranged in pleated, cylindrical, or disc-shaped configurations. Filter elements are selected based on factors such as filtration efficiency, dirt-holding capacity, fluid compatibility, and system requirements.
- Filter Housing: The filter housing is the enclosure or vessel that contains the filter elements and provides structural support and protection. It is typically made of materials such as steel, aluminum, or plastic and is designed to withstand hydraulic pressure, fluid flow, and environmental conditions. The filter housing includes inlet and outlet ports, mounting provisions, and connection points for hydraulic lines or hoses.
- Bypass Valve: The bypass valve is a safety feature incorporated into hydraulic filters to prevent excessive pressure drop across the filter element. It allows hydraulic fluid to bypass the filter element if the pressure drop exceeds a predetermined threshold, ensuring continuous flow to critical components and preventing system shutdown in case of filter clogging or saturation. The bypass valve may be integral to the filter housing or installed as a separate bypass assembly.
- Differential Pressure Gauge: The differential pressure gauge is a monitoring device used to measure the pressure drop across the filter element and indicate the condition of the filter. It consists of two pressure ports connected to the inlet and outlet of the filter housing, with a gauge or indicator displaying the pressure difference between the two points. An increase in pressure drop indicates that the filter is becoming clogged and requires maintenance or replacement.
- Fluid Sampling Ports: Fluid sampling ports are access points provided on the filter housing to extract samples of hydraulic fluid for analysis and testing. Fluid sampling allows for periodic assessment of fluid cleanliness, condition, and contamination levels, helping to identify potential issues and implement preventive maintenance measures to maintain system reliability and performance.
- Filter Maintenance: Proper maintenance of hydraulic filtration systems is essential to ensure optimal performance and longevity. Maintenance tasks include regular inspection of filter elements, housing integrity, and bypass valve operation; periodic replacement of filter elements based on manufacturer recommendations or fluid analysis results; and monitoring of differential pressure readings to detect signs of filter clogging or saturation.
- System Integration: Hydraulic filtration systems are integrated into hydraulic circuits or systems at strategic locations to effectively remove contaminants and protect critical components. Filtration systems may be installed upstream of pumps, valves, actuators, and other sensitive equipment to prevent damage and ensure reliable operation. Multiple filtration stages with different filtration ratings may be used to achieve desired cleanliness levels and meet specific application requirements.
Proper selection, sizing, installation, and maintenance of hydraulic filtration systems are essential to ensure optimal performance, reliability, and safety in hydraulic systems. Engineers and technicians must consider factors such as filtration efficiency, dirt-holding capacity, pressure rating, flow rate, fluid compatibility, environmental conditions, and system requirements when designing and implementing hydraulic filtration systems. Regular inspection, testing, and maintenance of filtration systems are necessary to detect signs of filter degradation, contamination buildup, or bypass valve malfunction and prevent hydraulic system failures and safety hazards.
Hydraulic Valve
A hydraulic valve is a mechanical device used to control the flow, direction, and pressure of hydraulic fluid within a hydraulic system. It serves as a crucial component in regulating the operation of hydraulic cylinders, motors, actuators, and other hydraulic components to achieve desired performance, functionality, and safety.
Key components and characteristics of hydraulic valves include:
- Valve Types: Hydraulic valves are available in various types, each with specific functions, operating principles, and applications. Common types of hydraulic valves include:
- Directional Control Valves: Directional control valves control the flow of hydraulic fluid and determine the direction of motion in hydraulic circuits. They include spool valves, poppet valves, and rotary valves, with configurations such as 2-way, 3-way, 4-way, and 5-way valves to control single or multiple hydraulic actuators.
- Pressure Control Valves: Pressure control valves regulate hydraulic pressure within the system to maintain desired pressure levels and protect components from overpressure conditions. They include relief valves, pressure reducing valves, pressure sequence valves, and counterbalance valves, which release excess pressure, reduce incoming pressure, or maintain pressure balance as needed.
- Flow Control Valves: Flow control valves regulate the flow rate of hydraulic fluid to control the speed and direction of hydraulic actuators. They include throttle valves, needle valves, and flow regulators, which restrict or regulate fluid flow to achieve precise control and synchronization of hydraulic system components.
- Proportional Valves: Proportional valves provide variable control of flow, pressure, or direction in proportion to an input signal, such as voltage, current, or pressure. They offer precise and smooth control of hydraulic system parameters and are commonly used in applications requiring fine-tuning and dynamic response, such as servo systems and motion control.
- Valve Actuation: Hydraulic valves are actuated using mechanical, electrical, pneumatic, or hydraulic methods to open, close, or modulate fluid flow within the system. Actuation methods include manual levers, push buttons, solenoids, pilot valves, servo valves, and proportional control systems, depending on the valve type, application requirements, and control strategy.
- Valve Construction: Hydraulic valves are typically constructed from materials such as steel, aluminum, brass, or plastic, chosen for their strength, durability, and corrosion resistance. Valve components may include a valve body, spool or poppet, seals, springs, actuators, ports, and connections, assembled into compact and robust units suitable for harsh operating environments.
- Seals and Gaskets: Seals and gaskets are used to prevent leakage of hydraulic fluid and ensure proper sealing and functionality of hydraulic valves. They are made of elastomeric materials such as Buna-N, Viton, or polyurethane and are designed to withstand high pressures, temperatures, and fluid compatibility requirements.
- Mounting and Installation: Hydraulic valves are mounted and installed within hydraulic circuits or systems using threaded connections, flanges, manifolds, or subplates. Proper mounting and installation are essential to ensure proper alignment, sealing, and operation of valves and prevent leakage, vibration, or damage during system operation.
Hydraulic valves are selected based on factors such as system requirements, flow rate, pressure rating, valve type, actuation method, and application considerations. Proper integration, calibration, and maintenance of hydraulic valves are essential to ensure optimal performance, reliability, and safety in hydraulic systems. Regular inspection, testing, and adjustment of valves are necessary to detect signs of wear, leakage, or malfunction and prevent hydraulic system failures and safety hazards.
Hydraulic Actuator
A hydraulic actuator is a mechanical device used to convert hydraulic energy into mechanical motion or force. It plays a crucial role in various applications across industries, providing powerful and precise movement for machinery, equipment, and systems.
Key components and characteristics of hydraulic actuators include:
- Actuator Types: Hydraulic actuators are available in various types, each with specific functions, operating principles, and applications. Common types of hydraulic actuators include:
- Hydraulic Cylinders: Hydraulic cylinders are linear actuators that produce linear motion in response to hydraulic pressure. They consist of a cylindrical housing (barrel), a piston, and a rod, with hydraulic fluid acting on one side of the piston to extend or retract the rod and produce linear movement. Hydraulic cylinders are widely used in applications such as industrial machinery, construction equipment, agricultural implements, and aerospace systems.
- Hydraulic Motors: Hydraulic motors are rotary actuators that produce rotational motion in response to hydraulic pressure. They consist of a rotor, stator, and fluid ports, with hydraulic fluid entering and exiting the motor to drive the rotor and produce rotary movement. Hydraulic motors are used in applications such as winches, cranes, conveyors, and vehicle propulsion systems.
- Operating Principle: Hydraulic actuators operate based on the principles of Pascal’s law, which states that pressure exerted at any point in a confined fluid is transmitted undiminished in all directions. Hydraulic fluid is pressurized by a pump and directed to the actuator, where it applies force to produce motion or torque. The actuator’s design and configuration determine the direction, speed, and force of the resulting mechanical output.
- Force and Torque: Hydraulic actuators generate force (in the case of cylinders) or torque (in the case of motors) to perform work and overcome resistance. The force or torque produced by the actuator depends on factors such as hydraulic pressure, piston or rotor size, operating conditions, and mechanical efficiency. Actuators are selected based on their ability to provide the required force or torque to move or manipulate loads within the system.
- Control and Feedback: Hydraulic actuators may be controlled manually, electrically, pneumatically, or hydraulically, depending on the application requirements and control strategy. Control systems may include valves, sensors, actuators, and feedback mechanisms to regulate fluid flow, pressure, direction, and speed and achieve precise positioning, speed control, and force/torque modulation.
- Construction and Materials: Hydraulic actuators are typically constructed from materials such as steel, aluminum, bronze, or cast iron, chosen for their strength, durability, and corrosion resistance. Actuator components may include cylinders, pistons, rods, seals, bearings, valves, and housings, assembled into robust and reliable units suitable for demanding operating environments.
- Mounting and Installation: Hydraulic actuators are mounted and installed within machinery, equipment, or systems using mounting brackets, flanges, clevises, or other attachment methods. Proper mounting and installation are essential to ensure alignment, stability, and structural integrity and prevent vibration, misalignment, or damage during operation.
Hydraulic actuators are selected based on factors such as application requirements, load characteristics, speed, force/torque requirements, space constraints, environmental conditions, and regulatory compliance. Proper integration, calibration, and maintenance of hydraulic actuators are essential to ensure optimal performance, reliability, and safety in hydraulic systems. Regular inspection, lubrication, and preventive maintenance are necessary to detect signs of wear, leakage, or malfunction and prevent hydraulic actuator failure and system downtime.
Hydraulic Press
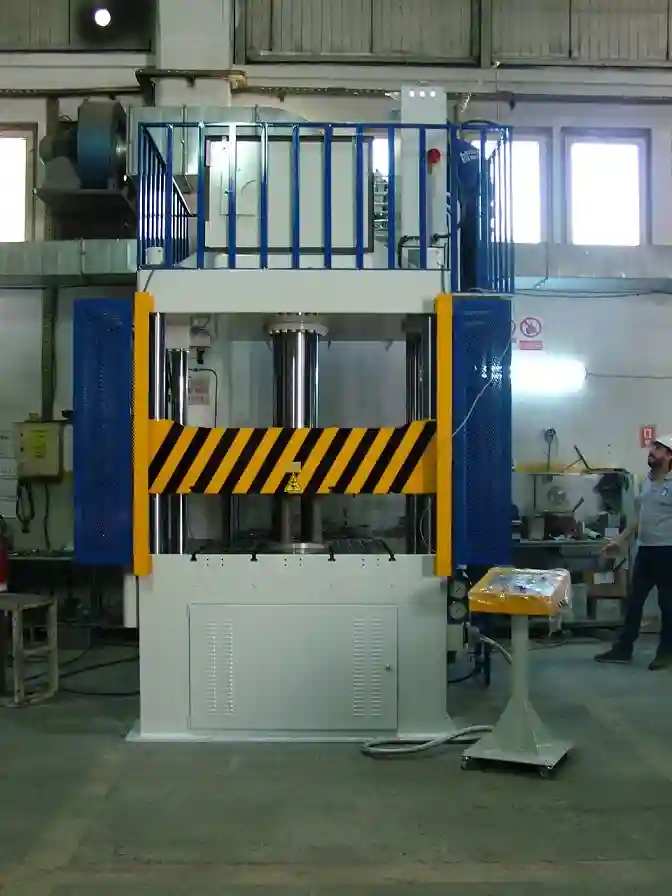
A hydraulic press is a machine that uses a hydraulic cylinder to generate a compressive force. It operates based on Pascal’s Law, which states that when pressure is applied to a confined fluid, the pressure change occurs throughout the entire fluid. In a hydraulic press, this principle is used to multiply the input force applied by the user, producing a much larger output force that can be used for various industrial tasks.
Hydraulic presses are widely employed across different industries due to their ability to exert significant force with great precision and control. These machines are essential for tasks that involve compressing, shaping, or manipulating materials, especially metals. For example, they are often used to form car parts, assemble machinery, and create complex metal shapes that would otherwise require considerable manual labor.
The hydraulic press consists of a few essential components: a hydraulic cylinder, a piston, a hydraulic pump, and hydraulic fluid. The machine functions by pushing hydraulic fluid into the cylinder, which in turn pushes the piston down, applying pressure to the object being worked on. The amount of force the press can apply is determined by the size of the piston and the hydraulic system’s pressure level.
Hydraulic presses are versatile and come in various sizes, ranging from small tabletop units to massive industrial machines capable of generating thousands of tons of force. Their ability to produce immense pressure efficiently has made them invaluable in modern manufacturing and engineering processes. In addition to their use in industries like automotive, aerospace, and manufacturing, hydraulic presses are also found in smaller, more specialized applications such as plastic molding, metal forming, and even recycling industries for crushing waste materials.
The advantages of hydraulic presses are numerous. They are generally more compact than mechanical presses, requiring less space and offering smoother, more controlled operations. Additionally, they provide a higher degree of flexibility and can be used for a wider range of materials, including metals, plastics, and composites.
In summary, hydraulic presses are crucial machines in the modern industrial landscape. Their efficiency, precision, and ability to generate enormous force make them an indispensable tool across a wide array of sectors. As technology continues to advance, hydraulic presses are also evolving, becoming more energy-efficient and integrating with smart manufacturing systems to meet the growing demands of modern industry.
History and Evolution of Hydraulic Presses
2.1. Origins of Hydraulic Technology
The history of hydraulic technology can be traced back to ancient times, long before the invention of the modern hydraulic press. Early civilizations such as the Egyptians, Greeks, and Romans developed rudimentary hydraulic systems to manage water resources, including aqueducts, irrigation channels, and waterwheels. These innovations, while focused primarily on water flow, laid the groundwork for the more sophisticated hydraulic systems that would emerge in later centuries.
However, the application of hydraulics to generate mechanical force didn’t come until much later. It was during the Renaissance that scientists and inventors began to develop a deeper understanding of fluid mechanics. One of the key figures in the development of hydraulic principles was Blaise Pascal, a French mathematician and physicist, who formulated Pascal’s Law in the 17th century. Pascal’s Law states that pressure applied to a confined fluid is transmitted equally in all directions. This principle is foundational to the function of hydraulic presses, enabling them to multiply the force applied to the system.
2.2. The Invention of the Hydraulic Press
The development of the hydraulic press as we know it today is credited to Joseph Bramah, an English inventor, in the late 18th century. Bramah was primarily known for his work on the development of locks, but his interest in hydraulics led him to design the first hydraulic press in 1795. His invention, which was called the Bramah press, was a breakthrough in industrial machinery and provided a practical demonstration of Pascal’s Law.
Bramah’s hydraulic press was revolutionary because it allowed for the exertion of massive amounts of force using only a small input effort. By applying pressure to a small piston, the press could generate a significantly larger force on a larger piston, which was used to compress or shape materials. This principle of force multiplication made Bramah’s hydraulic press far more efficient than any mechanical press that had been developed up until that time.
The Bramah press found immediate use in industrial applications such as metal forming, stamping, and forging. It allowed manufacturers to shape metals and other materials with precision and ease, leading to the widespread adoption of hydraulic presses across a range of industries.
2.3. Evolution through Industrial Revolutions
The hydraulic press underwent significant evolution during the First Industrial Revolution (late 18th to early 19th century). As industries began to expand and new technologies emerged, there was a growing need for more efficient machinery capable of handling the increased demand for mass production. Hydraulic presses were instrumental in this process, as they enabled manufacturers to produce large quantities of goods with greater precision and control than was previously possible.
During the Second Industrial Revolution (late 19th to early 20th century), advances in materials science and engineering led to the development of more powerful and durable hydraulic presses. Steel became the material of choice for constructing hydraulic press frames, replacing the cast iron that had been used previously. Additionally, new hydraulic fluids were developed, improving the efficiency and reliability of the press’s hydraulic systems.
The introduction of electric motors and pumps during this period also revolutionized the hydraulic press. Previously, hydraulic systems had relied on manual pumps or steam engines to generate pressure. With the advent of electric power, hydraulic presses could operate more consistently and at higher pressures, allowing for greater force output and increased production capacity.
2.4. Major Milestones in Hydraulic Press Development
As the 20th century progressed, hydraulic presses continued to evolve and become more specialized. Several key developments marked the ongoing improvement of hydraulic press technology:
- Hydraulic Press in Metal Forming (Mid-20th Century) The use of hydraulic presses in metalworking industries expanded significantly during the early to mid-20th century. Presses were now capable of handling extremely high pressures, which made them ideal for tasks like deep drawing, extrusion, and forging. These processes allowed manufacturers to create complex metal parts for industries like automotive, aerospace, and defense.
- Advancement in Control Systems (1960s – 1980s) The integration of programmable logic controllers (PLCs) and computerized numerical control (CNC) systems into hydraulic presses in the 1960s and 1970s greatly enhanced their precision and automation capabilities. These developments allowed operators to control the press with high levels of accuracy, ensuring repeatability and reducing errors in production.
- Servo-Hydraulic Systems (Late 20th Century) In the late 20th century, servo-hydraulic systems were introduced, which combined hydraulic power with electrical control systems. These systems offered significant advantages in terms of energy efficiency and precision. Servo-hydraulic presses allowed for precise control of speed, force, and position, making them ideal for applications requiring fine control, such as plastic molding or the production of delicate components.
- Emergence of Industry 4.0 Integration (21st Century) The 21st century brought with it the rise of Industry 4.0, the concept of smart manufacturing where machines are connected to the internet and can communicate data in real-time. Hydraulic presses have not been exempt from this transformation. Modern presses now feature smart sensors, remote monitoring capabilities, and predictive maintenance algorithms that help optimize performance and reduce downtime.
2.5. The Hydraulic Press Today
Today, hydraulic presses are more advanced than ever, with innovations that allow them to operate with precision, power, and efficiency. Modern presses can exert thousands of tons of force while maintaining tight tolerances, making them indispensable in industries ranging from automotive manufacturing to aerospace and beyond. Additionally, the continued development of energy-efficient systems and sustainable hydraulic fluids reflects the ongoing commitment to making hydraulic presses more environmentally friendly.
The hydraulic press remains a key player in modern industrial processes, and its evolution continues as new technologies such as artificial intelligence, machine learning, and big data are integrated into hydraulic systems to further enhance their capabilities. With this trajectory, hydraulic presses are poised to remain an essential tool in industrial manufacturing for years to come.
Components of a Hydraulic Press
Hydraulic presses are composed of various components that work together to generate and control the immense force required for tasks like metal forming, crushing, and stamping. Understanding the function and role of each part is crucial for grasping how these machines operate. Below, we’ll take an in-depth look at the main components of a hydraulic press.
3.1. Hydraulic System Overview
The hydraulic system is the heart of a hydraulic press. It uses hydraulic fluid to transmit power and amplify force. In essence, this system takes the mechanical input (manual or powered) and converts it into hydraulic pressure, which is used to perform tasks such as compressing, bending, or cutting materials.
A typical hydraulic system includes the following:
- Hydraulic fluid reservoir
- Hydraulic pump
- Cylinder
- Piston
- Control valves
- Pressure gauges
Let’s now examine each component in detail.
3.2. Key Components
3.2.1. Cylinder
The hydraulic cylinder is one of the most critical components of a hydraulic press. It is a mechanical actuator that converts hydraulic energy (pressure from the fluid) into linear motion. The cylinder houses the piston, which moves up and down within it.
- Construction: Typically, hydraulic cylinders are made from high-strength materials such as steel to withstand the immense pressures generated during operation.
- Single-acting vs. double-acting cylinders: In a single-acting cylinder, hydraulic fluid is applied to only one side of the piston, moving it in one direction, with a spring or other mechanism returning it to its original position. In contrast, a double-acting cylinder has fluid applied to both sides of the piston, allowing it to move in both directions, giving greater control and flexibility.
The force generated by the hydraulic press is directly proportional to the surface area of the cylinder and the pressure applied to the fluid.
3.2.2. Piston
The piston is another key part of the press. It is positioned inside the cylinder and moves up and down as hydraulic pressure is applied. The downward movement of the piston is what creates the compressive force used to shape or press materials.
- Force transmission: The piston transfers the hydraulic pressure into mechanical force. The larger the surface area of the piston, the greater the force it can apply. This is why hydraulic presses are capable of exerting much more force than what is applied by the operator or motor driving the system.
- Precision and control: Modern presses are equipped with highly responsive pistons, ensuring that they operate smoothly and with precision, even under significant loads.
3.2.3. Hydraulic Fluid
Hydraulic fluid is the medium that transmits force within the hydraulic system. It plays an essential role in the overall functioning of the hydraulic press, acting not only as a power transmitter but also as a lubricant and coolant.
- Types of hydraulic fluids: There are various types of hydraulic fluids used in presses, including:
- Mineral-based fluids: Most commonly used in general applications due to their affordability and effectiveness.
- Water-based fluids: Used in presses requiring fire resistance, as these fluids are less flammable.
- Synthetic fluids: Offer superior performance and longer lifespan in extreme conditions, such as high temperatures or where high corrosion resistance is needed.
The properties of hydraulic fluids, such as viscosity, thermal stability, and compressibility, affect the performance of the hydraulic press. Fluids must be regularly maintained and replaced to ensure consistent press operation.
3.2.4. Press Frame
The frame of the hydraulic press is its structural backbone, holding all the components together and providing the necessary rigidity to support the press’s operations. The frame design varies depending on the type of press, but the most common designs include:
- H-frame: Shaped like the letter “H”, this frame design provides strong support and is commonly used for heavy-duty applications.
- C-frame: Shaped like a “C”, this design is more compact and is typically used for lighter pressing tasks where space is limited.
- Four-column frame: This design uses four columns to support the press and is typically found in large presses used for manufacturing automotive or aerospace components.
The frame must be robust and durable to withstand the repeated high pressures that the press generates during operation.
3.2.5. Power Source (Hydraulic Pump)
The hydraulic pump is responsible for converting mechanical power into hydraulic energy by moving hydraulic fluid from the reservoir into the system. The pump creates a flow of fluid that allows the press to operate under pressure.
- Types of pumps: There are several different types of hydraulic pumps used in presses:
- Gear pumps: Simple and cost-effective, these pumps are suitable for applications with lower pressure requirements.
- Vane pumps: Known for being quiet and efficient, these pumps are often used in presses that require moderate pressures.
- Piston pumps: These pumps are the most powerful and are typically used in high-pressure hydraulic presses. They offer excellent precision and control.
The pump’s capacity directly affects how quickly the press can build pressure and how much force it can generate.
3.2.6. Valves and Controls
Hydraulic systems in presses use a variety of valves to control the flow and pressure of the fluid, ensuring that the press operates safely and efficiently. The key valves used in a hydraulic press include:
- Directional control valves: These valves control the direction of the fluid flow, determining whether the piston will move up or down.
- Pressure relief valves: To protect the system from over-pressurization, these valves release excess fluid back into the reservoir when pressure exceeds a safe level.
- Flow control valves: These valves regulate the speed of the press by controlling the flow rate of hydraulic fluid into the cylinder.
In modern presses, these valves are often operated electronically, providing precise control over the system’s pressure and motion.
3.2.7. Hydraulic Fluid Reservoir
The reservoir is where the hydraulic fluid is stored when not in use. It is typically a large tank that holds the fluid and allows it to be cooled, filtered, and cleaned before re-entering the hydraulic system.
- Cooling and filtering: Hydraulic fluid heats up during use, so the reservoir is equipped with cooling systems to dissipate heat. Additionally, filters remove impurities from the fluid, which could otherwise damage the system.
A well-maintained reservoir is crucial for preventing contamination and ensuring the longevity of the press.
3.2.8. Pressure Gauges and Sensors
Pressure gauges and sensors provide real-time data about the hydraulic system’s performance, allowing operators to monitor the pressure levels during press operation.
- Manual vs. digital gauges: Older hydraulic presses often use manual gauges, which require visual inspection. However, modern presses are equipped with digital sensors that feed information directly to the control systems, making monitoring more efficient.
- Safety and precision: These sensors are essential for maintaining safe operating conditions and ensuring that the press applies the correct force for each job.
3.3. The Interaction Between Components
All these components work together seamlessly to ensure the efficient operation of the hydraulic press. The pump sends hydraulic fluid from the reservoir into the cylinder, where pressure is applied to the piston. As the piston moves downward, it exerts force on the material placed beneath it. The valves and sensors regulate the flow and pressure of the fluid, while the frame provides structural support to withstand the forces generated by the press.
The effectiveness of a hydraulic press is dependent on the proper functioning and maintenance of each of these components. Any failure in one part of the system can lead to inefficiencies or even dangerous malfunctions. For example, leaks in the hydraulic fluid system can result in a loss of pressure, reducing the press’s ability to perform its tasks.
3.4. Modern Innovations in Hydraulic Components
Recent advancements in hydraulic technology have led to innovations in the components used in presses. Servo-hydraulic systems, for instance, have improved the efficiency and control of hydraulic presses, allowing for faster and more precise operations. Similarly, smart sensors are now being integrated into hydraulic systems, providing real-time feedback on performance and enabling predictive maintenance to avoid breakdowns.
The continuous evolution of these components ensures that hydraulic presses remain a critical tool in industrial processes, offering unmatched force and control for a wide range of applications.
Working Principle of a Hydraulic Press
The hydraulic press operates on the simple yet powerful principle of Pascal’s Law, which states that when pressure is applied to a confined fluid, the pressure is transmitted equally in all directions. This fundamental law of fluid mechanics enables hydraulic presses to amplify a small input force into a much larger output force. By manipulating hydraulic fluid in a sealed system, the hydraulic press can perform tasks such as compressing, bending, shaping, or cutting materials with remarkable efficiency.
In this section, we will explore the detailed working principle of hydraulic presses, focusing on how pressure is generated, how force is amplified, and how hydraulic systems manage energy efficiency and control.
4.1. Pascal’s Law and its Application in Hydraulic Presses
Pascal’s Law is the foundation of hydraulic technology. The law states that the pressure change in an incompressible and confined fluid is uniformly distributed throughout the fluid. The formula for Pascal’s Law is as follows:P=FAP = \frac{F}{A}P=AF
Where:
- P is pressure,
- F is force,
- A is the area over which the force is applied.
In a hydraulic press, this law is applied to multiply force through the use of two pistons—one smaller and one larger—connected by hydraulic fluid within a sealed system. When force is applied to the smaller piston, the pressure created is transmitted through the fluid to the larger piston, which results in a much larger force being exerted.
4.2. How Pressure is Generated
The basic operation of a hydraulic press begins with the generation of hydraulic pressure. This pressure is created by the hydraulic pump, which forces hydraulic fluid from the reservoir into the system. Once the fluid enters the cylinder, it pushes against the piston, causing it to move.
The pump provides the necessary energy to create this pressure. There are several types of hydraulic pumps, including:
- Gear pumps (common in smaller presses),
- Vane pumps (known for their efficiency),
- Piston pumps (used in high-pressure applications).
As the fluid is pushed into the cylinder, it creates a pressurized environment. This pressurized fluid exerts force on the piston, causing it to move in the direction of the applied pressure (usually downwards in most presses).
4.3. Force Amplification: From Small Piston to Large Piston
One of the most important aspects of a hydraulic press is its ability to amplify force. The hydraulic press uses two pistons of different sizes to achieve this amplification. Here’s how it works:
- Small piston: This piston has a smaller surface area and is the point at which the input force is applied, either manually (in smaller presses) or via a motor (in larger presses).
- Large piston: This piston has a much larger surface area and is responsible for generating the output force applied to the material being pressed.
Because Pascal’s Law states that pressure is constant throughout the system, the pressure generated at the small piston is transferred equally to the larger piston. However, since the larger piston has a greater surface area, the force it generates is proportionally larger.
Let’s break this down with an example:
- If you apply 100 Newtons of force to a small piston with a surface area of 1 cm², the pressure created is 100 N/cm².
- That same pressure is applied to the larger piston, which has a surface area of 10 cm². Therefore, the force on the larger piston will be 100 N/cm² × 10 cm² = 1000 Newtons.
This ability to amplify force makes hydraulic presses incredibly powerful. Even small input forces can generate thousands of Newtons of pressure, enabling the press to handle tasks like bending thick metal sheets or crushing large objects.
4.4. Hydraulic Fluid and Energy Transmission
The hydraulic fluid plays a crucial role in the transmission of force within the hydraulic press. As an incompressible medium, the fluid transmits pressure efficiently from the pump to the cylinder without significant losses.
- Types of hydraulic fluids: Commonly used fluids include mineral oils, water-based fluids, and synthetic fluids. The choice of fluid depends on the operating conditions of the press, including temperature, pressure, and the need for fire resistance.
Hydraulic fluid also acts as a lubricant for the moving parts within the system, reducing wear and tear on components like pistons, seals, and valves. Additionally, the fluid helps dissipate heat generated by the system, ensuring that the press operates efficiently even under high loads.
4.5. Control of Pressure and Force
Controlling the pressure within the hydraulic system is essential for ensuring that the press operates safely and efficiently. The pressure and force applied by the press can be controlled using several methods:
- Pressure relief valves: These valves release excess fluid back into the reservoir when the pressure exceeds safe operating limits. This prevents the system from becoming over-pressurized, which could cause damage or pose a safety hazard.
- Directional control valves: These valves direct the flow of hydraulic fluid within the system, determining whether the piston moves up or down. They allow the operator to control the direction of force application.
- Flow control valves: These valves regulate the flow rate of hydraulic fluid, which in turn controls the speed of the press. By adjusting the flow, operators can ensure that the press moves at the desired speed for each task.
In modern presses, these controls are often automated or managed via computer systems, allowing for precise and repeatable operations. Programmable Logic Controllers (PLCs) and Computer Numerical Control (CNC) systems enable operators to set specific pressure, force, and speed parameters, which the press then follows automatically.
4.6. Energy Efficiency and Conservation in Hydraulic Systems
One of the challenges associated with hydraulic presses is energy efficiency. Traditional hydraulic systems can be relatively inefficient because the pump runs continuously, even when the press is not in operation, consuming energy and generating heat. However, recent innovations have improved the energy efficiency of hydraulic presses:
- Variable displacement pumps: These pumps adjust the amount of hydraulic fluid being moved depending on the demand of the system. When the press is idle or requires less pressure, the pump reduces its output, conserving energy.
- Servo-hydraulic systems: These systems combine hydraulic power with electrical control. In servo-hydraulic presses, electric motors control the pump, adjusting its speed to match the force and speed requirements of the press. This results in lower energy consumption, reduced noise, and improved precision.
- Hydraulic accumulators: These devices store energy in the form of pressurized fluid, which can be released when needed. Accumulators help reduce the load on the pump during peak operation, improving overall system efficiency.
These advancements have made modern hydraulic presses far more energy-efficient than their predecessors, making them more environmentally friendly and cost-effective.
4.7. Advantages of Hydraulic Press Operation
The working principle of hydraulic presses offers several key advantages:
- Force multiplication: Hydraulic presses can amplify a relatively small input force into a much larger output force, making them ideal for heavy-duty applications like metal forming and compression.
- Precision: Hydraulic presses allow for precise control over pressure and speed, enabling manufacturers to produce complex, high-quality parts.
- Versatility: Hydraulic presses can be used for a wide range of materials and processes, from metal forming and plastic molding to recycling and waste management.
- Efficiency: Modern presses with servo-hydraulic systems and variable displacement pumps are energy-efficient and can reduce operational costs.
4.8. Limitations of Hydraulic Press Operation
While hydraulic presses offer numerous advantages, they also have some limitations:
- Energy consumption: Traditional hydraulic systems can consume significant amounts of energy, especially if the pump runs continuously.
- Maintenance: Hydraulic systems require regular maintenance, including checking fluid levels, replacing worn seals, and cleaning filters. Contamination in the hydraulic fluid can reduce system performance and lead to component wear.
- Noise: Hydraulic presses, particularly those with older pumps, can generate significant noise during operation, though modern systems are designed to be quieter.
Types of Hydraulic Presses
Hydraulic presses are available in various designs, each suited to specific industrial tasks. Depending on the force required, size, and operational method, different types of hydraulic presses are employed across industries, such as automotive manufacturing, aerospace, metalworking, plastic molding, and recycling. This section will explore the different types of hydraulic presses, comparing their designs and applications.
5.1. Manual Hydraulic Presses
Manual hydraulic presses are the simplest form of hydraulic presses, operated by hand. These presses are typically small, compact, and designed for tasks that require low to moderate pressure, such as small-scale metalworking, pressing bearings, or assembling components. They are often used in workshops, laboratories, and maintenance shops where precision work and control are needed, but high force is not necessary.
- Key features:
- Operated by a hand pump to generate hydraulic pressure.
- Usually consist of a single-acting cylinder that moves in one direction when pressure is applied and returns to its original position using a spring.
- Suitable for small, precise tasks like shaping or straightening metal parts, pressing in or removing bearings, and assembling components.
Advantages:
- Inexpensive and easy to operate.
- No need for external power sources, making them ideal for small workshops or remote locations.
- High precision for small-scale jobs.
Limitations:
- Limited force output compared to powered presses.
- Slower operation due to manual pumping.
- Suitable only for light-duty applications.
5.2. Powered Hydraulic Presses
Powered hydraulic presses are larger and more versatile than manual presses. These presses are driven by either electric or pneumatic systems and can generate much higher forces, making them suitable for industrial applications. There are several types of powered hydraulic presses, each designed for specific tasks.
5.2.1. Electric Hydraulic Presses
Electric hydraulic presses use an electric motor to drive the hydraulic pump, which generates pressure in the hydraulic system. These presses are common in manufacturing and metalworking industries, where consistent, high-force applications are required.
- Key features:
- Powered by an electric motor that drives the hydraulic pump.
- Offers precise control over force and speed through adjustable settings.
- Can be equipped with CNC or programmable control systems for automation.
Applications:
- Metal forming, stamping, and bending.
- Deep drawing processes in the automotive and aerospace industries.
- Precision tasks in plastic molding or die cutting.
Advantages:
- High force generation for heavy-duty applications.
- Precise control over force and speed, ideal for complex, high-volume tasks.
- Can be integrated into automated production lines for efficiency.
Limitations:
- Higher energy consumption compared to manual or pneumatic presses.
- Requires regular maintenance of the electrical and hydraulic systems.
- Can be expensive to purchase and operate.
5.2.2. Pneumatic Hydraulic Presses
Pneumatic hydraulic presses use compressed air to generate hydraulic pressure. While not as powerful as electric hydraulic presses, they are more energy-efficient and often used in applications that do not require extremely high forces.
- Key features:
- Powered by compressed air rather than electricity.
- Ideal for lighter tasks that still require hydraulic force but do not need the high power output of electric presses.
- Used in environments where electrical power may not be readily available or where lower force and speed are acceptable.
Applications:
- Assembly lines for light manufacturing tasks.
- Punching, bending, and forming lighter materials.
- Plastic molding and small-scale metalworking.
Advantages:
- More energy-efficient than electric presses for lighter tasks.
- Lower operating costs due to reduced energy consumption.
- Suitable for environments where electrical systems pose a safety risk (e.g., explosive or flammable environments).
Limitations:
- Limited force output compared to electric-powered presses.
- Slower operational speeds.
- Not suitable for heavy-duty applications.
5.3. Specialized Hydraulic Presses
In addition to manual and powered presses, there are also specialized hydraulic presses designed for specific industrial processes. These presses vary in their frame design, size, and operational capabilities.
5.3.1. H-Frame Presses
H-frame hydraulic presses are named for the shape of their frame, which resembles the letter “H.” They are among the most common hydraulic presses used in industries due to their versatility and ability to handle both light and heavy-duty applications.
- Key features:
- A strong, rigid frame shaped like an “H,” which provides excellent structural support and stability during operation.
- Can be equipped with single or double-acting cylinders.
- Available in both manual and powered configurations, making them suitable for a range of applications.
Applications:
- Metal forming, stamping, and punching.
- Straightening, bending, and assembling large parts.
- Automotive repair (e.g., pressing out bearings or bushings).
Advantages:
- Versatile and capable of handling both small and large tasks.
- High force output, suitable for heavy-duty applications.
- Widely used across many industries due to their robust construction.
Limitations:
- The larger size of some models can take up significant floor space.
- Requires careful alignment during operation to ensure even force distribution.
5.3.2. C-Frame Presses
C-frame hydraulic presses are more compact than H-frame presses and are shaped like the letter “C.” This design provides easy access to the work area from three sides, making C-frame presses ideal for tasks that require loading and unloading materials quickly.
- Key features:
- Open-front design allows for easy access to the pressing area.
- Generally smaller and more compact than H-frame presses.
- Ideal for tasks that require frequent adjustments or quick material changes.
Applications:
- Light to medium-duty applications such as punching, bending, and forming smaller parts.
- Assembling components in the electronics or automotive industries.
- Precision pressing tasks where easy access to the workpiece is necessary.
Advantages:
- Compact and space-efficient.
- Easy access to the pressing area, reducing downtime between tasks.
- Precise control over force and speed for smaller applications.
Limitations:
- Limited to lighter tasks compared to H-frame presses.
- The open design can cause slight deformation under extreme loads, reducing its effectiveness for heavy-duty tasks.
5.3.3. Four-Column Hydraulic Presses
Four-column hydraulic presses have four vertical columns that provide structural support for the press. This design is ideal for applications that require evenly distributed force across a large workpiece.
- Key features:
- Four vertical columns provide excellent stability and even force distribution.
- Can handle large workpieces and high force applications.
- Commonly used in heavy-duty industries such as automotive and aerospace manufacturing.
Applications:
- Metal forming, die cutting, and large-scale forging.
- Deep drawing processes that require precise, even pressure.
- Pressing large or heavy workpieces where even force is critical.
Advantages:
- Excellent stability and even force distribution, reducing the risk of material deformation.
- Capable of handling large workpieces.
- High force output, suitable for heavy-duty industrial tasks.
Limitations:
- Large and heavy, requiring significant floor space.
- Higher energy consumption due to the size and force capabilities of the press.
5.3.4. Bench Presses
Bench hydraulic presses are smaller presses designed to be mounted on a workbench or table. These presses are used for light-duty applications in workshops, laboratories, and small-scale manufacturing environments.
- Key features:
- Small, compact design that fits on a workbench or tabletop.
- Usually operated manually or with a small hydraulic pump.
- Ideal for light-duty tasks where precision is more important than force.
Applications:
- Assembling small components, such as in electronics or jewelry making.
- Light metalworking tasks, such as bending or pressing small parts.
- Laboratory testing and material sample preparation.
Advantages:
- Compact and easy to use in small workspaces.
- Precise control over pressing force.
- Inexpensive and suitable for small-scale tasks.
Limitations:
- Limited force output, unsuitable for large or heavy-duty applications.
- Small size limits the range of tasks that can be performed.
5.4. Comparative Analysis of Hydraulic Press Types
Each type of hydraulic press has its strengths and is suited to particular applications. The following table summarizes the key characteristics of the different press types:
Type | Force Output | Size | Applications | Advantages | Limitations |
---|---|---|---|---|---|
Manual Press | Low | Small | Light assembly, workshops | Low cost, portable | Limited force, slower operation |
Electric Hydraulic Press | High | Medium-Large | Manufacturing, metal forming | High force, precision control, automation | Higher energy consumption, requires maintenance |
Pneumatic Press | Moderate | Medium | Assembly lines, lighter manufacturing | Energy efficient, lower operating costs | Limited force output, slower than electric presses |
H-Frame Press | High | Large | Metal forming, straightening, heavy-duty tasks | High force, versatile | Requires floor space, careful alignment needed |
C-Frame Press | Moderate | Small-Medium | Precision tasks, light to medium duty | Compact, easy access to work area | Limited to lighter tasks, less stable under heavy loads |
Four-Column Press | High | Large | Large-scale metal forming, deep drawing | Excellent force distribution, handles large workpieces | Requires significant space, high energy consumption |
Bench Press | Low | Small | Small-scale assembly, testing | Compact, precise control, low cost | Limited force output, small work area |
Applications of Hydraulic Presses
Hydraulic presses are versatile machines used across a broad range of industries, from automotive manufacturing and aerospace to plastic molding and recycling. Their ability to generate immense force while maintaining precision makes them essential in various industrial processes, including forming, cutting, shaping, and assembling materials. In this section, we’ll explore the key industries and applications where hydraulic presses play a vital role.
6.1. Automotive Industry
The automotive industry heavily relies on hydraulic presses for a variety of tasks, particularly in manufacturing car components, bodywork, and assemblies. The versatility of hydraulic presses allows for the precise and repeatable production of complex metal and plastic parts used in vehicles.
6.1.1. Pressing Car Parts
Hydraulic presses are used to manufacture critical car components such as:
- Chassis parts: The frames that provide structural support for vehicles are often formed using hydraulic presses. The high force generated allows the press to mold thick sheets of metal into the desired shapes.
- Body panels: Hydraulic presses are essential for creating body panels and hoods, where precise shaping is required to ensure proper fit and finish. The metal stamping process uses high-force hydraulic presses to cut, bend, and shape large sheets of metal into the necessary parts.
6.1.2. Brake and Clutch Plates
In the production of brake and clutch plates, hydraulic presses are used to compress and shape friction materials. These materials need to be highly durable and accurately produced to ensure vehicle safety. The controlled force of a hydraulic press ensures that each component meets the required specifications, contributing to the vehicle’s overall performance and reliability.
6.1.3. Assembly and Fabrication
Hydraulic presses also assist in the assembly of vehicle parts, such as joining or securing metal pieces together through compression. This process is particularly important in tasks like fastening bushings, bearings, and seals into their respective housings.
6.2. Manufacturing Industry
In general manufacturing, hydraulic presses are indispensable for processes like metal forming, stamping, forging, and plastic molding. The precision and power of hydraulic presses make them ideal for handling both lightweight and heavy-duty applications.
6.2.1. Metal Forming, Stamping, and Forging
One of the most significant uses of hydraulic presses is in metal forming, where the press shapes and molds metal into complex parts. Stamping and forging are specific methods within this category:
- Stamping: Involves pressing sheet metal into a die to create precise shapes or patterns. Hydraulic presses are used to cut, bend, or punch holes in metal sheets, creating components used in everything from household appliances to industrial machinery.
- Forging: Hydraulic presses apply immense force to a piece of metal, shaping it while it is hot. This process is used to create stronger, more durable parts, such as gears, engine components, and tools. Forging under hydraulic pressure ensures consistent material strength and structural integrity.
6.2.2. Plastic Molding
Hydraulic presses are essential in plastic injection molding and compression molding. In these processes, hydraulic presses:
- Inject molten plastic into molds to create parts with precise dimensions, such as automotive interiors, medical devices, or packaging components.
- In compression molding, plastic material is placed in a mold, and the hydraulic press applies force to shape the plastic. This process is often used to make large plastic components, such as housings or casings.
6.3. Aerospace Industry
The aerospace industry demands highly specialized components with precise dimensions and superior strength-to-weight ratios. Hydraulic presses are essential in forming parts for aircraft, spacecraft, and defense systems.
6.3.1. Shaping Aircraft Parts
In aerospace, hydraulic presses are used for metal forming, extrusion, and deep drawing to shape metal into complex, lightweight parts that meet stringent performance and safety requirements. Examples include:
- Fuselage panels: Large hydraulic presses shape the lightweight aluminum or composite materials used in aircraft fuselages.
- Wings and structural components: Precision is critical when forming aircraft wings and structural components to ensure they meet aerodynamics and load-bearing specifications.
The ability of hydraulic presses to handle materials like titanium and aluminum alloys, which are commonly used in aerospace due to their strength and low weight, makes them indispensable in aircraft manufacturing.
6.3.2. Assembly of Aerospace Systems
Hydraulic presses are also used in assembling and joining parts in aerospace systems. For example:
- Hydraulic riveting presses secure fasteners in aircraft body panels and components.
- Assembly presses are used to install precision bearings, bushings, and other critical components.
6.4. Construction and Engineering
In the construction and heavy engineering industries, hydraulic presses are used for various applications, including compressing construction materials, assembling heavy machinery, and shaping structural components.
6.4.1. Compression of Construction Materials
Hydraulic presses are often used to compress and shape materials such as concrete, brick, and tiles. For example:
- Pre-cast concrete components: Hydraulic presses shape and compress concrete into pre-cast blocks or panels used in building construction.
- Brick and tile production: In this process, the press compresses raw materials like clay or cement into bricks or tiles with consistent shapes and sizes.
6.4.2. Assembly of Heavy Machinery
Hydraulic presses play a crucial role in the assembly of heavy machinery and equipment used in construction. They are often used to press bearings, bushings, or other components into machinery parts like hydraulic cylinders, engines, and gear systems. The controlled application of force ensures that parts are assembled securely and without damaging sensitive components.
6.5. Agricultural Industry
Hydraulic presses also find applications in the agricultural industry, where they are used to process materials and create equipment parts.
6.5.1. Compressing Hay Bales
Hydraulic presses are used in agriculture to compress hay, straw, or other forage into compact bales for easy storage and transport. The press applies significant pressure to reduce the volume of the material while retaining its quality for feeding livestock.
6.5.2. Farm Equipment Assembly
Similar to the automotive and construction industries, hydraulic presses are used in the assembly of farm equipment, such as pressing bearings, bushings, and other components into tractors, plows, and harvesters. The precision of hydraulic presses ensures that the parts are securely and accurately installed, enhancing the reliability of agricultural machinery.
6.6. Recycling Industry
Hydraulic presses play a critical role in the recycling industry, particularly in the processing of scrap materials and waste management. They are used to crush, compact, or bale materials to prepare them for recycling or disposal.
6.6.1. Metal Recycling
In metal recycling, hydraulic presses are used to compress scrap metal into compact blocks or bales for easier transportation and processing. For example:
- Car body recycling: Hydraulic presses crush old car bodies into manageable sizes for melting down and recycling.
- Scrap metal baling: Loose metal scrap, such as aluminum cans, steel, and copper, is baled using a hydraulic press, reducing its volume and making it easier to transport and store before it is reprocessed.
6.6.2. Waste Management
Hydraulic presses are also used in waste management facilities to compact household and industrial waste into dense, manageable bales. This process reduces the space needed for waste storage and simplifies transportation to recycling or disposal facilities. The ability to compress materials like plastic, cardboard, and paper ensures more efficient waste handling and disposal.
6.7. Home Workshops and DIY
Hydraulic presses are not limited to large industrial applications. Smaller hydraulic presses are commonly used in home workshops and for do-it-yourself (DIY) projects. These compact presses offer hobbyists and small businesses a versatile tool for various applications.
6.7.1. Metalworking
In home workshops, hydraulic presses are frequently used for:
- Bending and shaping metal: Small hydraulic presses are used to bend metal bars, rods, or sheets into desired shapes for custom projects, such as making furniture, gates, or decorative items.
- Removing or installing bearings: Home mechanics and hobbyists use hydraulic presses to remove old bearings from machines or vehicle parts and press in new ones, ensuring proper fit and function.
6.7.2. Woodworking and Crafting
Hydraulic presses are sometimes used in woodworking and crafting, particularly in tasks that require compression or molding. For example:
- Veneer pressing: Hydraulic presses are used to compress thin sheets of wood veneer onto furniture surfaces, creating a smooth, uniform bond.
- Custom molds: In crafting, hydraulic presses can be used to create custom molds for making unique items like jewelry, art pieces, or decorative panels.
6.8. Hydraulic Press in Research and Testing
Hydraulic presses are widely used in research and testing environments for materials testing, particularly in laboratories where the mechanical properties of materials are evaluated.
6.8.1. Compression Testing
In materials science, hydraulic presses are used for compression testing to determine the strength and durability of materials. For example, concrete, metals, plastics, and composites are tested to see how much force they can withstand before deforming or breaking. The controlled pressure applied by the hydraulic press allows researchers to study how materials behave under stress.
6.8.2. Product Testing and Prototyping
Hydraulic presses are also used to test the durability and performance of finished products or prototypes. This includes tasks like:
- Durability testing: Products like car parts, electronics, or industrial components are subjected to high pressures to determine their durability and resistance to wear.
- Prototyping: In the development phase of new products, hydraulic presses can be used to shape or mold prototype parts to ensure that they meet design specifications before mass production begins.
6.9. Other Specialized Applications
Hydraulic presses can also be found in many other specialized applications, ranging from medical device manufacturing to the production of consumer goods.
6.9.1. Medical Device Manufacturing
In the medical field, hydraulic presses are used to manufacture precision components for devices like pacemakers, prosthetics, and surgical tools. The high precision offered by hydraulic presses ensures that each component meets strict medical standards for quality and safety.
6.9.2. Jewelry and Watchmaking
Hydraulic presses are also used in the jewelry and watchmaking industries to create intricate designs and shapes. For example:
- Metal stamping: Hydraulic presses are used to stamp designs onto metal sheets for jewelry making.
- Shaping watch components: Precision hydraulic presses form parts like watch cases, bezels, and straps, ensuring a perfect fit and high-quality finish.
Outline for Components of a Hydraulic Press
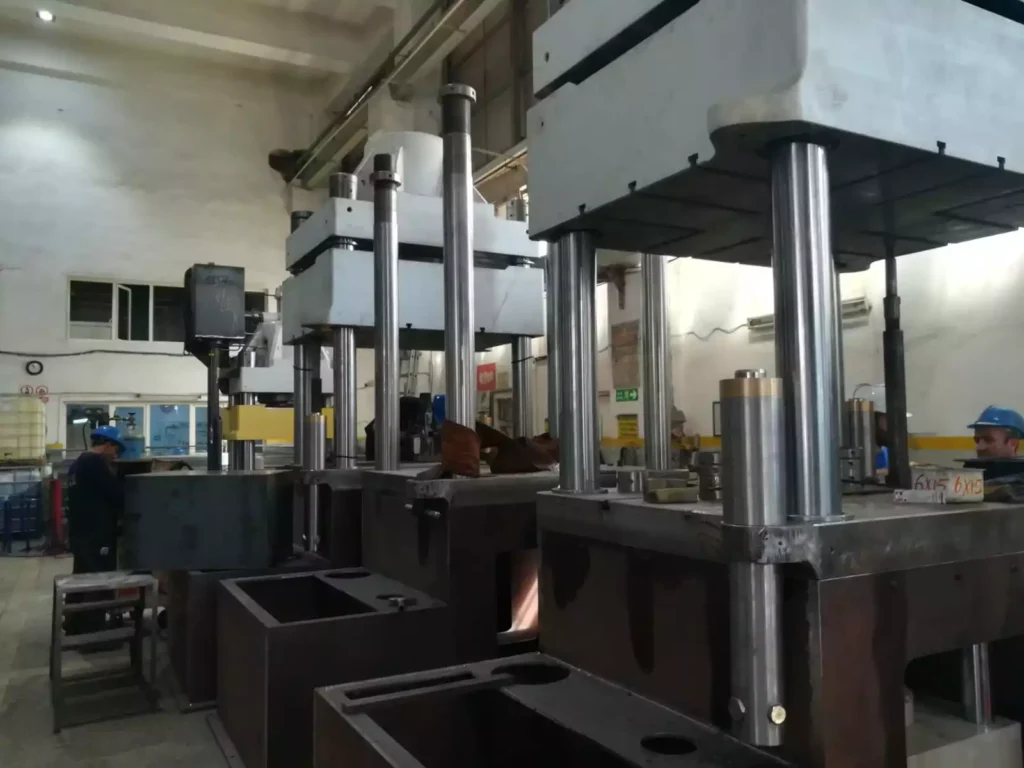
A hydraulic press is a mechanical machine that uses hydraulic pressure to compress, bend, shape, or cut materials. The core principle behind a hydraulic press is Pascal’s Law, which states that pressure applied to a confined fluid is transmitted equally in all directions. This principle allows hydraulic presses to amplify force, enabling the machine to perform heavy-duty tasks with minimal input force.
At the heart of a hydraulic press is its hydraulic system, which consists of several key components: the hydraulic cylinder, piston, hydraulic fluid, pump, valves, and reservoir. These components work together to generate pressure, transmit force, and control the operation of the press. The hydraulic system allows for smooth, consistent application of force, making the press highly efficient and reliable.
Hydraulic presses are used in a wide range of industries, from automotive manufacturing and metalworking to plastic molding and recycling. Understanding the components of a hydraulic press is essential for maintaining its performance, optimizing its efficiency, and preventing breakdowns. Each part of the hydraulic system plays a critical role in its overall operation, and even minor issues with one component can impact the entire machine.
The hydraulic press system is highly adaptable, with different types of presses and configurations designed for specific applications. From small bench presses used in workshops to massive four-column presses in automotive plants, hydraulic systems can be tailored to the task at hand, whether it involves forming metal parts, shaping plastic components, or compressing scrap materials for recycling.
The efficiency of hydraulic presses has also improved significantly over the years, thanks to innovations in hydraulic fluid, pump design, and control systems. Modern hydraulic presses now integrate smart sensors, programmable controls, and servo-hydraulic systems, allowing operators to control pressure, force, and speed with extreme precision.
Maintaining a hydraulic press involves regular monitoring of components like the hydraulic cylinder, pump, and fluid system. Regular maintenance ensures that the press operates safely and effectively, minimizing downtime and extending the life of the equipment.
In summary, the hydraulic press system is a finely tuned machine composed of various interdependent components. A well-maintained hydraulic system ensures that the press operates efficiently, delivering the force and precision required for industrial applications.
Hydraulic Cylinder
The hydraulic cylinder is one of the most crucial components of a hydraulic press, responsible for converting hydraulic pressure into linear motion. It is the part of the system that directly generates the force required to press, compress, or shape the material. The cylinder houses the piston and is filled with hydraulic fluid, which, when pressurized, pushes the piston forward to perform the desired task.
2.1. Function and Significance of the Hydraulic Cylinder
The primary role of the hydraulic cylinder is to create the force needed to perform the press’s work. When hydraulic fluid is pumped into the cylinder, the resulting pressure pushes against the piston, causing it to move in a linear motion. This movement is transferred to the material being worked on, whether it involves compressing metal, forming plastic, or bending steel.
Hydraulic cylinders are designed to handle immense pressures, often in the range of several hundred to several thousand PSI (pounds per square inch), depending on the size and capacity of the press. The strength of the cylinder, along with its design and material construction, determines the overall force output of the press.
2.2. Types of Hydraulic Cylinders
There are several types of hydraulic cylinders, each designed for specific applications and press designs.
2.2.1. Single-Acting Cylinders
A single-acting cylinder uses hydraulic pressure to move the piston in one direction only. The return stroke is typically powered by a spring or gravity. These cylinders are simpler in design and are often used in presses where the return stroke does not require significant force.
- Advantages:
- Simple and cost-effective design
- Requires less hydraulic fluid and a smaller pump
- Commonly used in manual presses or lighter-duty applications
2.2.1. Single-Acting Cylinders (Continued)
- Limitations (continued):
- The return stroke relies on external forces (such as springs or gravity), which may result in slower or less controlled movements.
- They are less suitable for applications requiring consistent force in both directions (pressing and releasing).
Single-acting cylinders are often found in smaller hydraulic presses, especially in tasks such as light pressing, assembling, or simple bending. They are typically used when the return stroke does not need to be fast or forceful, such as in certain assembly line tasks or in small workshops where simplicity and cost-effectiveness are prioritized.
2.2.2. Double-Acting Cylinders
In contrast to single-acting cylinders, double-acting cylinders are designed to exert force in both directions—both during the forward (pressing) and the return strokes. This is achieved by applying hydraulic pressure alternately to either side of the piston. Double-acting cylinders are more versatile and powerful, as they offer full control over the press’s movements.
- Advantages:
- Force can be applied in both directions, offering greater control over the entire operation.
- They are faster and more efficient than single-acting cylinders, as the return stroke can be powered hydraulically rather than relying on gravity or springs.
- Ideal for applications that require high precision and speed, such as metal forming, stamping, and forging.
- Limitations:
- More complex and expensive than single-acting cylinders.
- Requires a larger hydraulic pump and more hydraulic fluid to operate effectively.
Double-acting cylinders are commonly used in heavy-duty hydraulic presses, especially those found in large-scale industrial applications like automotive manufacturing and metalworking. Their ability to exert force in both directions makes them ideal for tasks requiring precision and speed, such as deep drawing, metal extrusion, and heavy-duty forging.
2.2.3. Telescopic Cylinders
A telescopic hydraulic cylinder, also known as a multi-stage cylinder, consists of several smaller cylinders nested within each other. These cylinders extend in stages, allowing for a longer stroke in a more compact design. Telescopic cylinders are often used in applications where space is limited, but a long stroke length is required, such as in lifting or pressing operations.
- Advantages:
- Capable of delivering a very long stroke in a compact form.
- Useful for applications where space is limited but the press needs to extend over a large distance.
- Commonly used in heavy machinery, dump trucks, and other lifting devices.
- Limitations:
- More complex design and construction lead to higher costs.
- Potential for more frequent maintenance due to the multiple stages of extension and retraction.
In hydraulic presses, telescopic cylinders are typically used when space is a concern, and a longer extension is needed to reach or compress materials. They are often found in construction equipment, large industrial presses, and certain custom-designed presses where long reach is essential.
2.3. Construction of Hydraulic Cylinders
The construction of a hydraulic cylinder is critical to its performance and longevity. The materials and manufacturing methods used must ensure that the cylinder can withstand high pressure, friction, and repeated use without failure.
2.3.1. Materials Used
Most hydraulic cylinders are made from high-strength steel or alloy materials. Steel is favored for its durability and ability to withstand the extreme pressures generated by hydraulic systems. Some components, such as the cylinder’s rod, are often plated with chrome or other anti-corrosive coatings to reduce friction and wear, and to protect the rod from corrosion.
- Steel: The primary material used in heavy-duty cylinders due to its high strength and resistance to deformation under pressure.
- Composite materials: In some specialized applications, lightweight composite materials may be used to reduce the weight of the cylinder while maintaining strength.
2.3.2. Seals and Rod Coatings
The seals used within hydraulic cylinders are critical to maintaining pressure and preventing fluid leaks. Common types of seals include O-rings, U-cups, and rod seals, all designed to prevent the escape of hydraulic fluid around the piston and rod.
- Seals: Typically made from materials like rubber, polyurethane, or PTFE (Teflon), seals are chosen based on their resistance to heat, wear, and hydraulic fluid. High-performance presses may use self-lubricating seals, which reduce the friction between moving parts and extend the lifespan of the cylinder.
- Rod coatings: To reduce wear and increase longevity, hydraulic cylinders often have chrome-plated rods. The chrome plating provides a hard, smooth surface that resists corrosion and minimizes friction between the rod and seals.
2.4. Cylinder Dynamics: Fluid Movement and Force Transmission
The movement of the hydraulic fluid within the cylinder is what enables the press to generate force. When the hydraulic pump pushes fluid into the cylinder, it creates pressure behind the piston, causing it to move forward. The size of the cylinder, the amount of hydraulic fluid pumped into it, and the surface area of the piston all affect how much force is generated.
- Force transmission: Pascal’s Law is central to the operation of a hydraulic press. The pressure applied to the fluid in the cylinder is transmitted equally in all directions, pushing the piston forward with amplified force. The size of the piston and cylinder determines the force multiplication.
- Cylinder size and pressure: Larger cylinders can exert more force because of the greater surface area over which the hydraulic pressure acts. However, the larger the cylinder, the more hydraulic fluid is required to move the piston, which means the press’s pump and reservoir must be appropriately sized.
2.5. Innovations in Cylinder Design for Longevity and Efficiency
Recent advancements in hydraulic cylinder design have focused on improving efficiency, durability, and energy savings. Some innovations include:
- Servo-hydraulic systems: These systems allow for more precise control over the movement of the piston, improving the accuracy of the press’s operation and reducing energy consumption. Servo-hydraulic systems adjust the pressure and flow of hydraulic fluid based on the task, resulting in less wasted energy.
- Lightweight materials: While most hydraulic cylinders are made from steel, some newer designs use composite materials to reduce the weight of the press without sacrificing strength. This is especially useful in mobile or lightweight presses where portability is a concern.
- Improved seal technology: Advances in sealing materials, such as the use of self-lubricating seals, have extended the lifespan of hydraulic cylinders by reducing wear and preventing leaks.
2.6. Common Hydraulic Cylinder Issues
Despite their robust design, hydraulic cylinders can experience problems, often related to wear and tear or improper maintenance.
2.6.1. Leaks
Leaks are one of the most common issues in hydraulic cylinders, often caused by worn or damaged seals. A leaking cylinder will lose pressure, reducing the press’s effectiveness and potentially causing the machine to malfunction. Regular maintenance and timely replacement of seals can prevent leaks.
2.6.2. Pressure Loss
Pressure loss can occur if there is damage to the cylinder or its seals, or if there is air trapped in the hydraulic system. Low pressure reduces the force generated by the press and can lead to uneven or incomplete pressing.
2.6.3. Wear and Tear
Over time, the piston rod, seals, and cylinder walls can become worn due to friction and repeated use. Wear and tear are particularly common in presses that operate under high pressures or in harsh environments. Regular inspection and maintenance are necessary to extend the life of the hydraulic cylinder.
3. Pistons
The piston in a hydraulic press is a critical component responsible for transferring the hydraulic pressure generated in the cylinder to the material being pressed. It is the moving part that directly interacts with the hydraulic fluid and converts this pressure into mechanical force.
3.1. Role of the Piston in a Hydraulic Press
The piston’s primary role is to convert the pressure from the hydraulic fluid into the linear motion required for pressing, forming, or compressing materials. When the hydraulic fluid enters the cylinder, it pushes against the piston, causing it to move. This motion is what allows the press to apply force to the material being worked on, whether it’s bending metal, compressing plastic, or cutting sheet metal.
3.2. Force Transmission: Amplification of Input Force
The piston in a hydraulic press works in tandem with the hydraulic cylinder to amplify the input force. Through Pascal’s Law, the small force applied to the hydraulic fluid at the pump is multiplied when transmitted to the larger piston surface area inside the cylinder. The larger the piston, the greater the force that can be applied to the material.
For example:
- In a hydraulic press with a small input piston and a large output piston, even a small amount of pressure at the input results in a much larger force being applied by the output piston. This is the key to the hydraulic press’s ability to generate high forces with minimal input effort.
3.3. Materials and Coatings: Ensuring Durability Under Pressure
The materials used in the construction of the piston are crucial to its durability, as it must withstand high pressures and repeated cycles of movement. Most pistons are made from high-strength steel, with certain coatings applied to reduce friction and prevent corrosion.
- Steel pistons: Steel is the most commonly used material for pistons due to its strength and durability. Steel pistons can handle the high pressures typically found in hydraulic presses without deforming or wearing down quickly.
- Coatings: To reduce friction and extend the life of the piston, it is often coated with materials such as chrome or nickel. These coatings provide a hard, smooth surface that resists wear and corrosion, allowing the piston to operate smoothly over time.
3.4. Interaction with Hydraulic Fluid: Sealing and Pressure Maintenance
The piston must maintain a perfect seal with the cylinder walls to ensure that hydraulic pressure is contained and transmitted effectively. To achieve this, piston seals are used. These seals prevent hydraulic fluid from leaking past the piston, which would reduce the press’s effectiveness and lead to pressure loss.
- Seals: Piston seals are typically made from rubber, PTFE (Teflon), or polyurethane, depending on the press’s operating conditions. These materials are chosen for their ability to withstand high pressures, temperatures, and exposure to hydraulic fluids.
A well-maintained seal ensures that the piston can move freely within the cylinder while maintaining constant pressure on the material being pressed.
3.5. Types of Pistons for Different Press Designs
Different types of pistons are used in hydraulic presses, depending on the press’s design and intended application. Some of the common piston designs include:
- Single-piece pistons: These are the most common type of piston used in hydraulic presses. They are made from a single piece of material, typically steel, and are designed for standard pressing tasks.
- Telescopic pistons: Used in presses where a longer stroke is needed, telescopic pistons consist of multiple segments that extend outward during operation, allowing the press to apply force over a longer distance.
- Custom pistons: In specialized presses, pistons may be custom-designed to meet the unique requirements of the application. These pistons may include additional features, such as integrated sensors or advanced coatings to handle extreme conditions.
3.6. Common Problems with Pistons and Solutions
Like hydraulic cylinders, pistons are subject to wear and tear, and issues can arise if they are not properly maintained. Common problems include:
3.6.1. Wear and Scoring
Over time, the piston can become worn or develop scoring (scratches or grooves) due to friction between the piston and the cylinder walls. This can reduce the piston’s effectiveness and lead to pressure loss. Regular inspection and replacement of worn pistons can prevent this issue.
3.6.2. Seal Failures
If the piston seals wear out or become damaged, hydraulic fluid can leak past the piston, reducing pressure and force. Replacing worn seals regularly and ensuring that the piston is properly lubricated can prevent seal failures.
3.6.3. Piston Deformation
In high-pressure applications, the piston can become deformed if it is not designed to handle the force generated by the press. Deformed pistons can lead to uneven pressure distribution and inefficient operation. Using pistons made from high-strength materials and ensuring that they are correctly sized for the application can prevent deformation.
Hydraulic Fluid
Hydraulic fluid is the lifeblood of any hydraulic press, responsible for transmitting the force generated by the pump to the cylinder and piston. It plays a critical role in ensuring the smooth operation of the press, lubricating moving parts, and dissipating heat generated during operation. The choice of hydraulic fluid has a significant impact on the performance, efficiency, and longevity of the hydraulic system.
4.1. Importance of Hydraulic Fluid in Force Transmission
The primary function of hydraulic fluid is to transmit force within the press. When the hydraulic pump pressurizes the fluid, the pressure is transmitted through the fluid to the cylinder and piston. Hydraulic fluid is incompressible, meaning that any pressure applied to it is transmitted equally throughout the system, ensuring efficient force transmission.
- Force transmission: Pascal’s Law is the guiding principle behind the use of hydraulic fluid. The pressure applied to the fluid at the pump is transmitted to the piston, where it is converted into mechanical force. This allows the hydraulic press to exert significant force with relatively low input effort.
- Fluid dynamics: The movement of hydraulic fluid within the system must be smooth and consistent to avoid pressure spikes or drops. Proper fluid management, including filtering and temperature control, is essential for maintaining consistent pressure throughout the hydraulic system.
4.2. Types of Hydraulic Fluids
The type of hydraulic fluid used in a press depends on the specific requirements of the application, including operating temperature, pressure, and environmental considerations. The most common types of hydraulic fluids are:
4.2.1. Mineral Oils
Mineral oils are the most commonly used hydraulic fluids in presses due to their affordability, availability, and overall effectiveness. Derived from petroleum, mineral oils offer good lubricating properties and are capable of withstanding the high pressures typically found in hydraulic systems.
- Advantages:
- Widely available and cost-effective.
- Good lubrication and anti-wear properties.
- Suitable for a wide range of hydraulic press applications.
- Disadvantages:
- Mineral oils can degrade at high temperatures.
- They are not environmentally friendly and can pose disposal challenges.
4.2.2. Water-Based Fluids
Water-based hydraulic fluids are typically used in applications where fire resistance is a concern. These fluids are less flammable than mineral oils and are often used in presses that operate in environments with high heat or where the risk of fire is present.
- Advantages:
- Non-flammable, making them ideal for high-heat environments.
- Provide good cooling properties due to their water content.
- Disadvantages:
- Water-based fluids offer less lubrication than mineral oils.
- They are prone to corrosion and must be carefully managed to prevent damage to the hydraulic system.
4.2.3. Synthetic Fluids
Synthetic hydraulic fluids are engineered to provide superior performance in extreme conditions, such as high temperatures or environments requiring high resistance to oxidation and wear. These fluids are often used in high-performance hydraulic presses that operate under demanding conditions.
- Advantages:
- Excellent thermal stability and resistance to oxidation.
- Long lifespan and reduced maintenance requirements.
- Suitable for high-pressure and high-temperature applications.
- Disadvantages:
- More expensive than mineral oils and water-based fluids.
- Disposal can be more complex due to their chemical composition.
4.2.4. Biodegradable Fluids
Biodegradable hydraulic fluids are gaining popularity due to their reduced environmental impact. These fluids are made from renewable sources, such as vegetable oils, and are designed to degrade naturally without harming the environment.
- Advantages:
- Environmentally friendly and biodegradable.
- Suitable for presses used in environmentally sensitive applications, such as agriculture or marine industries.
- Disadvantages:
- Biodegradable fluids may not perform as well at extreme temperatures or pressures.
- More expensive than traditional mineral oils.
4.3. Properties of Hydraulic Fluid
The properties of hydraulic fluid have a significant impact on the performance and efficiency of the hydraulic press. The most important properties include:
4.3.1. Viscosity
Viscosity refers to the fluid’s resistance to flow. Hydraulic fluids with the proper viscosity ensure smooth operation and efficient force transmission within the system.
- Low viscosity: Fluids with low viscosity flow more easily, but may not provide enough lubrication, leading to increased wear on moving parts.
- High viscosity: Fluids with high viscosity provide better lubrication but may flow too slowly, resulting in inefficient force transmission.
Selecting a hydraulic fluid with the appropriate viscosity for the operating conditions of the press is essential for maintaining performance and preventing wear.
4.3.2. Compressibility
Hydraulic fluids must be incompressible to effectively transmit force. Any compressibility in the fluid would result in energy loss and reduced efficiency. Fluids designed for hydraulic presses are formulated to minimize compressibility, ensuring that all applied pressure is transferred directly to the piston.
4.3.3. Thermal Stability
Hydraulic presses generate heat during operation, and the hydraulic fluid must be able to withstand high temperatures without breaking down. Thermal stability refers to the fluid’s ability to maintain its properties under heat. Fluids with high thermal stability resist oxidation and degradation, ensuring consistent performance even under heavy loads.
4.3.4. Lubricity
Hydraulic fluids must also act as a lubricant, reducing friction between moving parts within the hydraulic system. Proper lubrication reduces wear and extends the lifespan of components such as the cylinder, piston, and seals.
4.4. Fluid Contamination: Causes, Effects, and Prevention
Contamination of hydraulic fluid is one of the most common causes of hydraulic press failure. Contaminants such as dirt, metal particles, or water can enter the hydraulic system and reduce the effectiveness of the fluid, leading to damage and system failure.
4.4.1. Causes of Contamination
- External contamination: Dust, dirt, and debris can enter the hydraulic system through leaks, worn seals, or during fluid changes.
- Internal contamination: Wear and tear on the system’s components can generate metal particles or other debris, which then circulate within the fluid.
- Water ingress: Moisture can enter the system through condensation or leaks, leading to corrosion and reduced fluid performance.
4.4.2. Effects of Contamination
- Reduced fluid efficiency: Contaminants reduce the fluid’s ability to transmit pressure effectively, leading to inefficiencies in force transmission.
- Increased wear: Contaminated fluid can accelerate wear on the cylinder, piston, and seals, leading to premature failure of these components.
- Corrosion: Water contamination can cause corrosion within the hydraulic system, leading to leaks and system failure.
4.4.3. Preventing Fluid Contamination
- Filtration: Installing high-quality filters in the hydraulic system helps remove contaminants before they can cause damage. Regularly changing or cleaning filters is essential for preventing contamination.
- Seals and gaskets: Ensuring that seals and gaskets are in good condition prevents external contaminants from entering the system.
- Proper fluid handling: When adding or replacing hydraulic fluid, it’s important to ensure that the new fluid is clean and free of contaminants. Using proper containers and handling methods can prevent contamination during fluid changes.
4.5. Hydraulic Fluid Maintenance: Filtering, Cooling, and Fluid Replacement
Maintaining the hydraulic fluid is critical for the press’s long-term performance. This includes regular filtering, cooling, and fluid replacement.
4.5.1. Filtering
Regular filtering removes particles and contaminants from the fluid, preventing them from circulating through the system. Presses are typically equipped with inline filters that capture debris as the fluid moves through the system.
- Filter replacement: Filters should be inspected and replaced at regular intervals to ensure that they remain effective. A clogged or worn filter can reduce fluid flow and cause pressure loss.
4.5.2. Cooling
Hydraulic fluid can become hot during operation, leading to degradation and reduced performance. A cooling system, such as a heat exchanger or a reservoir with cooling fins, helps regulate the fluid’s temperature and prevents overheating.
- Fluid cooling systems: Some hydraulic presses are equipped with active cooling systems, such as liquid cooling or air-cooled heat exchangers, which help maintain optimal fluid temperatures during operation.
4.5.3. Fluid Replacement
Over time, hydraulic fluid degrades and loses its effectiveness. Regularly replacing the fluid ensures that the press continues to operate efficiently. Fluid replacement intervals depend on the type of fluid used, the operating conditions, and the press’s workload.
5. Press Frame
The press frame is the backbone of a hydraulic press, providing the structural support necessary to handle the immense forces generated during operation. The frame plays a crucial role in maintaining the alignment of the hydraulic cylinder, piston, and other components, ensuring that force is applied evenly to the material being worked on. In this section, we will explore the different types of press frames, their construction materials, and how frame design impacts the performance and durability of a hydraulic press.
5.1. Role of the Press Frame
The primary role of the press frame is to support the components of the hydraulic press and to withstand the forces generated during pressing operations. The frame must be rigid enough to prevent deformation or flexing under pressure, which could lead to misalignment of components and uneven force application. A strong, well-designed frame ensures that the press operates efficiently and safely, especially when handling high-force applications such as metal stamping, forging, or deep drawing.
Additionally, the frame must be designed to accommodate the specific tasks for which the press is used. For example, presses designed for metal forming may require larger, more robust frames to handle the increased stresses, while smaller presses for light assembly work can utilize more compact frames.
5.2. Types of Press Frames
There are several types of hydraulic press frames, each designed for specific applications. The choice of frame design depends on factors such as the size of the press, the force required, and the type of material being worked on.
5.2.1. H-Frame Hydraulic Press
The H-frame press is one of the most common types of hydraulic press frames, named for its resemblance to the letter “H.” This design features two vertical columns (the “legs” of the H) connected by horizontal beams (the “crossbar” of the H). The hydraulic cylinder and piston are mounted in the center of the crossbar, with the workpiece placed between the legs.
- Key features:
- Versatility: H-frame presses are used in a wide variety of applications, from metal forming and punching to straightening and bending.
- Strength: The frame provides excellent structural support, allowing the press to generate high forces without flexing or deforming.
- Open design: The open structure of the H-frame allows for easy access to the work area, making it easier to load and unload materials.
- Applications: H-frame presses are commonly used in heavy-duty applications such as automotive repair, metal fabrication, and industrial manufacturing.
5.2.2. C-Frame Hydraulic Press
The C-frame press, also known as a gap-frame press, is designed with an open front that resembles the letter “C.” This design provides easy access to the work area from three sides, allowing operators to load and unload materials quickly. C-frame presses are typically more compact than H-frame presses and are often used for smaller, lighter-duty tasks.
- Key features:
- Compact design: C-frame presses are ideal for applications where space is limited, as they take up less floor space than larger H-frame presses.
- Easy access: The open-front design allows operators to access the work area from multiple sides, making it easier to position materials and tools.
- Moderate force: While C-frame presses can handle moderate forces, they are generally not suitable for heavy-duty applications.
- Applications: C-frame presses are commonly used for tasks such as punching, stamping, and light assembly work. They are often found in workshops, small manufacturing facilities, and maintenance shops.
5.2.3. Four-Column Hydraulic Press
The four-column press features four vertical columns that provide structural support for the press. This design is often used in large, high-force applications where even pressure distribution and structural rigidity are critical. Four-column presses are typically used in industries such as automotive manufacturing, aerospace, and heavy machinery production.
- Key features:
- Even force distribution: The four-column design ensures that force is applied evenly across the entire workpiece, reducing the risk of material deformation.
- Large work area: Four-column presses can accommodate large workpieces, making them ideal for tasks such as metal forming, die cutting, and deep drawing.
- High force capacity: These presses are capable of generating extremely high forces, making them suitable for heavy-duty applications.
- Applications: Four-column presses are commonly used in large-scale industrial operations, such as metal forming, forging, and deep drawing in the automotive and aerospace industries.
5.2.4. Custom-Designed Frames
In some cases, hydraulic presses are designed with custom frames to meet the specific needs of the application. Custom-designed frames may incorporate elements of H-frame, C-frame, or four-column designs, but are tailored to the unique requirements of the press, such as handling irregularly shaped materials or operating in confined spaces.
- Key features:
- Tailored design: Custom frames are engineered to meet the specific demands of the task, ensuring optimal performance and efficiency.
- Specialized materials: Custom frames may be made from specialized materials or alloys to withstand extreme forces, temperatures, or environmental conditions.
- Applications: Custom hydraulic press frames are often used in specialized industries such as medical device manufacturing, electronics production, and advanced materials processing.
5.3. Frame Materials: Steel, Composites, and Lightweight Designs
The materials used in the construction of a press frame have a significant impact on its strength, durability, and performance. Most hydraulic press frames are made from high-strength steel, which offers excellent resistance to deformation under pressure. However, advances in materials science have led to the development of composite materials and lightweight designs that offer similar strength with reduced weight.
5.3.1. Steel Frames
Steel is the most commonly used material in hydraulic press frames due to its high tensile strength, durability, and ability to withstand heavy loads. Steel frames can be manufactured to handle both light and heavy-duty applications, making them versatile and reliable.
- Advantages:
- High strength and durability.
- Suitable for a wide range of applications, from light assembly to heavy metal forming.
- Can be easily fabricated into various frame designs (H-frame, C-frame, four-column).
- Disadvantages:
- Steel frames are heavy, which can make installation and transportation more difficult.
- Susceptible to corrosion if not properly treated or maintained.
5.3.2. Composite Materials and Lightweight Frames
In some specialized applications, composite materials are used to reduce the weight of the press frame without sacrificing strength. Composites such as carbon fiber or fiberglass-reinforced plastics offer high strength-to-weight ratios, making them ideal for presses that need to be portable or operate in weight-sensitive environments.
- Advantages:
- Lightweight, making the press easier to move and install.
- Resistant to corrosion and environmental degradation.
- Suitable for specialized applications where weight is a concern.
- Disadvantages:
- More expensive to manufacture than steel frames.
- May not offer the same level of durability as steel in heavy-duty applications.
5.4. Stress Distribution and Frame Performance
The design of the press frame plays a crucial role in how well it distributes the forces generated during pressing operations. Stress distribution refers to how evenly the force is spread across the frame and the workpiece. A well-designed frame ensures that the force is applied uniformly, reducing the risk of material deformation and improving the quality of the final product.
5.4.1. Preventing Frame Deformation
Press frames must be designed to resist deformation, which can occur if the frame is not strong enough to handle the forces generated by the hydraulic system. Deformation can lead to misalignment of the hydraulic cylinder and piston, reducing the efficiency of the press and potentially causing damage to the workpiece.
- Reinforcement: Frames can be reinforced with additional supports or crossbeams to increase their rigidity and prevent bending or flexing under pressure.
- Material selection: Using high-strength materials such as steel or composites can reduce the risk of frame deformation.
5.4.2. Impact on Press Performance
The rigidity and design of the press frame directly impact the performance of the hydraulic press. A strong, well-designed frame ensures that the press operates efficiently and consistently, allowing for precise control over force application. This is particularly important in industries such as automotive and aerospace, where even small deviations in force distribution can affect the quality and safety of the final product.
Power Source: Hydraulic Pump
The hydraulic pump is the heart of the hydraulic press, responsible for generating the hydraulic pressure that powers the press’s operations. The pump converts mechanical energy into hydraulic energy by moving hydraulic fluid from the reservoir into the system, creating the pressure necessary to move the piston and apply force to the material. In this section, we will explore the different types of hydraulic pumps, their efficiency, and recent innovations in pump design.
6.1. Function of the Hydraulic Pump
The primary function of the hydraulic pump is to create a flow of hydraulic fluid that is pressurized and directed into the hydraulic cylinder. The pump determines the amount of force that the press can generate, as the pressure in the system is directly related to the flow rate and the size of the hydraulic cylinder.
When the hydraulic pump is activated, it draws fluid from the reservoir and forces it into the cylinder. As the fluid enters the cylinder, it pushes the piston forward, creating the force needed to press, compress, or shape the material. The amount of pressure generated by the pump is a key factor in determining the overall force output of the hydraulic press.
6.2. Types of Hydraulic Pumps
There are several types of hydraulic pumps used in presses, each with its own advantages and limitations. The choice of pump depends on the specific requirements of the press, such as the desired pressure, flow rate, and energy efficiency.
6.2.1. Gear Pumps
Gear pumps are one of the most common types of hydraulic pumps used in smaller hydraulic presses. They are simple, reliable, and cost-effective, making them ideal for applications where moderate pressure and flow rates are required.
- Advantages:
- Simple design with few moving parts, reducing the risk of mechanical failure.
- Inexpensive and easy to maintain.
- Suitable for low to moderate pressure applications.
- Disadvantages:
- Less efficient than other types of pumps, especially at higher pressures.
- Limited pressure output, making them unsuitable for heavy-duty applications.
6.2.2. Vane Pumps
Vane pumps are known for their quiet operation and efficient performance. These pumps use rotating vanes to move fluid through the system, providing smooth, consistent flow. Vane pumps are often used in presses that require moderate pressure and flow rates, such as those found in plastic molding or assembly operations.
- Advantages:
- Quiet operation and smooth fluid flow.
- Efficient and reliable in moderate pressure applications.
- Longer lifespan due to reduced wear on components.
- Disadvantages:
- More expensive than gear pumps.
- Not suitable for high-pressure applications.
6.2.3. Piston Pumps
Piston pumps are the most powerful type of hydraulic pump, capable of generating extremely high pressures. These pumps use a series of pistons to move fluid through the system, providing precise control over pressure and flow rate. Piston pumps are commonly used in heavy-duty hydraulic presses, such as those found in metal forging, deep drawing, and large-scale manufacturing.
- Advantages:
- Capable of generating very high pressures, making them ideal for heavy-duty applications.
- Precise control over pressure and flow rate, allowing for accurate operation.
- Durable and long-lasting, even under extreme conditions.
- Disadvantages:
- More complex and expensive than gear or vane pumps.
- Requires regular maintenance to prevent wear and mechanical failure.
6.2.4. Fixed vs. Variable Displacement Pumps
Hydraulic pumps can be categorized as either fixed displacement or variable displacement based on how they control the flow of fluid.
- Fixed displacement pumps deliver a constant flow of hydraulic fluid at a set rate, regardless of the pressure in the system. These pumps are simpler and less expensive but are less energy-efficient, as they cannot adjust the flow rate to match the demands of the press.
- Variable displacement pumps can adjust the flow rate of hydraulic fluid based on the system’s needs. These pumps are more energy-efficient, as they only deliver the amount of fluid necessary to perform the task. Variable displacement pumps are commonly used in modern hydraulic presses, where energy efficiency and precision are important.
6.3. Pump Efficiency: Power-to-Pressure Ratio
The efficiency of a hydraulic pump is determined by its power-to-pressure ratio, which refers to how much mechanical energy is required to generate a given amount of hydraulic pressure. Efficient pumps can generate high pressures with minimal energy input, reducing operating costs and energy consumption.
- Energy-efficient pumps: Pumps with variable displacement designs are generally more energy-efficient than fixed displacement pumps, as they can adjust their output based on the press’s needs. This reduces the amount of energy wasted when the press is operating at lower pressures or during idle periods.
- Servo-hydraulic pumps: Some modern hydraulic presses use servo-hydraulic pumps, which combine hydraulic power with electrical control. These pumps use a servo motor to control the speed and flow of the hydraulic fluid, allowing for precise pressure control and reduced energy consumption. Servo-hydraulic systems are becoming more common in high-performance presses due to their energy efficiency and improved control.
6.4. Innovations in Pump Design: Energy-Efficient Models
Recent advancements in pump design have focused on improving energy efficiency and reducing the environmental impact of hydraulic presses. Some of the key innovations include:
- Servo-hydraulic systems: As mentioned earlier, servo-hydraulic systems offer precise control over the pump’s operation, allowing the press to adjust its pressure and flow rate in real-time based on the task at hand. This leads to significant energy savings and improved performance.
- Variable speed pumps: Pumps that can adjust their speed based on the press’s needs are becoming increasingly popular in industrial presses. By reducing the pump’s speed during low-demand periods, these systems reduce energy consumption and heat generation, improving the overall efficiency of the press.
- Compact pump designs: Advances in materials and manufacturing techniques have led to the development of compact hydraulic pumps that offer high pressure in a smaller footprint. These pumps are ideal for presses where space is limited, such as portable or bench-mounted presses.
6.5. Pump Maintenance and Common Issues
Hydraulic pumps are subject to wear and tear over time, and regular maintenance is essential for ensuring that the press continues to operate efficiently. Some of the most common issues with hydraulic pumps include:
6.5.1. Wear and Mechanical Failure
Over time, the internal components of the pump, such as gears, vanes, or pistons, can become worn due to friction and heat. This can lead to reduced efficiency, noise, and eventual mechanical failure. Regular inspection and maintenance of the pump’s moving parts are essential for preventing breakdowns.
- Preventive maintenance: Replacing worn components and ensuring that the hydraulic fluid is clean and free of contaminants can extend the lifespan of the pump and reduce the risk of mechanical failure.
6.5.2. Fluid Flow Issues
Pumps can experience problems with fluid flow due to blockages, leaks, or air bubbles in the system. These issues can reduce the pump’s efficiency and lead to pressure loss, reducing the overall performance of the press.
- Troubleshooting: Regularly checking the hydraulic system for leaks, blockages, and proper fluid levels can help identify and resolve flow issues before they impact the press’s operation.
6.5.3. Noise and Vibration
Excessive noise and vibration are often signs of issues with the hydraulic pump, such as worn bearings or misaligned components. If left unchecked, these problems can lead to mechanical failure and reduced performance.
- Inspection: Regularly inspecting the pump for signs of wear, alignment issues, or abnormal noise can help prevent more serious problems from developing.
7. Valves and Controls
Valves and controls are crucial elements of a hydraulic press, managing the flow and pressure of hydraulic fluid to ensure smooth, efficient, and safe operation. These components determine the direction of fluid movement, regulate pressure, and control the flow rate, allowing the hydraulic press to perform precise operations such as pressing, stamping, molding, or forging. This section will cover the types of valves used in hydraulic presses, their function, modern control systems, and common issues related to valves and control mechanisms.
7.1. Control Systems in a Hydraulic Press
The control system in a hydraulic press consists of a series of valves, switches, and sensors that regulate the movement of hydraulic fluid through the system. The operator can adjust these controls to manage the speed, pressure, and direction of the press’s movement. The main components of the control system include:
- Valves: These control the flow and pressure of the hydraulic fluid within the system.
- Sensors: These monitor the system’s pressure, position, and temperature, providing real-time feedback to the control system.
- Programmable Logic Controllers (PLCs): In modern presses, PLCs are used to automate and manage the operation of the press. They allow the operator to program specific tasks and control the press remotely.
Together, these components ensure that the press operates with precision, safety, and efficiency, allowing for repeatable and accurate results in industrial applications.
7.2. Types of Valves in Hydraulic Presses
Hydraulic systems use a variety of valves to control the direction, pressure, and flow rate of the fluid. The main types of valves include directional control valves, pressure relief valves, and flow control valves.
7.2.1. Directional Control Valves
Directional control valves are responsible for directing the flow of hydraulic fluid within the system. These valves determine whether the fluid is sent to the piston to extend or retract the press. Directional control valves can be manually operated or automated, depending on the press’s design.
- Types of directional control valves:
- Manual valves: Operated by hand, these are typically used in smaller, simpler presses.
- Solenoid valves: Electrically operated, solenoid valves are commonly used in modern hydraulic presses for more precise control of fluid direction.
- Pilot-operated valves: These valves use hydraulic or pneumatic signals to control the flow of fluid, allowing for faster and more efficient operation.
7.2.2. Pressure Relief Valves
Pressure relief valves play a critical safety role in hydraulic presses by preventing over-pressurization. These valves automatically release hydraulic fluid when the pressure in the system exceeds a predetermined level, protecting the press from damage and ensuring safe operation.
- Adjustable pressure relief valves: Operators can adjust the pressure threshold at which the valve opens, allowing for flexibility in different applications.
- Safety function: Pressure relief valves prevent damage to the hydraulic system by releasing excess pressure and returning fluid to the reservoir.
7.2.3. Flow Control Valves
Flow control valves regulate the speed at which hydraulic fluid flows through the system. By controlling the flow rate, these valves determine how fast the press moves, ensuring smooth operation and precise control over pressing tasks.
- Types of flow control valves:
- Throttle valves: These allow the operator to manually adjust the flow rate, providing precise control over the press’s speed.
- Pressure-compensated valves: These valves automatically adjust the flow rate based on the system’s pressure, ensuring consistent speed even under varying load conditions.
7.3. Modern Control Innovations
Advancements in hydraulic press technology have led to the development of more sophisticated control systems, including PLCs, CNC integration, and smart sensors. These innovations allow for greater automation, precision, and safety in hydraulic press operations.
7.3.1. Programmable Logic Controllers (PLCs)
PLCs are computerized control systems that allow operators to program and automate the operation of the hydraulic press. PLCs enable the press to perform complex tasks with minimal human intervention, improving efficiency and repeatability in industrial applications.
- Automation: PLCs allow operators to program specific pressing sequences, adjust pressure and speed settings, and monitor performance remotely.
- Improved accuracy: PLCs ensure that pressing tasks are performed consistently, reducing errors and improving product quality.
7.3.2. CNC Integration
Some hydraulic presses are equipped with CNC (Computer Numerical Control) systems, which provide even greater precision and control over pressing operations. CNC systems allow for highly accurate positioning and force control, making them ideal for tasks such as metal forming, stamping, and precision assembly.
- Precision: CNC systems provide real-time feedback and adjustments, ensuring that the press operates within exact tolerances.
- Flexibility: CNC-controlled presses can be programmed to handle a wide range of tasks, from simple pressing to complex multi-step operations.
7.3.3. Smart Sensors and Remote Monitoring
Modern hydraulic presses are increasingly equipped with smart sensors that provide real-time data on the system’s pressure, temperature, and position. These sensors allow operators to monitor the press’s performance remotely, enabling predictive maintenance and reducing downtime.
- Remote monitoring: Sensors send data to a centralized control system, allowing operators to monitor the press’s performance and detect potential issues before they cause breakdowns.
- Predictive maintenance: By analyzing data from smart sensors, operators can schedule maintenance based on the actual condition of the press, reducing the risk of unexpected failures and extending the lifespan of the machine.
7.4. Valve Issues and Maintenance
Valves are subject to wear and tear over time, and issues with valves can lead to poor press performance or system failure. Common problems with valves in hydraulic presses include leaks, blockages, and pressure loss.
7.4.1. Leaks
Leaks in directional control valves or pressure relief valves can cause a drop in hydraulic pressure, reducing the force generated by the press. Leaks are often caused by worn seals, damaged valve components, or contamination in the hydraulic fluid.
- Solution: Regular inspection and maintenance of valves can help identify and fix leaks before they lead to larger issues. Replacing worn seals and cleaning valve components are common preventive measures.
7.4.2. Blockages
Blockages in flow control valves can disrupt the smooth movement of hydraulic fluid through the system, causing the press to operate erratically. Blockages are typically caused by dirt, debris, or contamination in the hydraulic fluid.
- Solution: Installing high-quality filters in the hydraulic system can prevent contaminants from entering the valves. Regularly cleaning or replacing filters is essential to preventing blockages.
7.4.3. Pressure Loss
Pressure loss in the system can occur if pressure relief valves open prematurely or if directional control valves are not functioning properly. This can result in reduced pressing force and inconsistent operation.
- Solution: Calibrating the pressure relief valves and ensuring that all valves are functioning correctly can help maintain consistent pressure levels in the system.
Reservoir and Cooling System
The reservoir and cooling system of a hydraulic press play a crucial role in maintaining the performance and longevity of the machine. The reservoir stores the hydraulic fluid, while the cooling system regulates the fluid’s temperature, preventing overheating and ensuring efficient operation. This section will explore the design and function of the hydraulic reservoir and cooling system, as well as the importance of regular maintenance.
8.1. Role of the Reservoir
The hydraulic reservoir serves as the storage tank for the hydraulic fluid used in the press. Its primary functions include:
- Storing hydraulic fluid: The reservoir holds the hydraulic fluid when it is not being circulated through the system, ensuring that there is always a sufficient supply of fluid for the press to operate.
- Cooling: As hydraulic fluid passes through the system, it absorbs heat generated by the press’s components. The reservoir allows the fluid to cool before it is recirculated back into the system.
- Contamination management: The reservoir also serves as a settling tank where contaminants such as dirt, metal particles, and air bubbles can be removed from the fluid before it re-enters the hydraulic system.
The size and design of the reservoir depend on the size of the press and the amount of fluid required for its operation. Larger presses require larger reservoirs to ensure an adequate supply of fluid and efficient cooling.
8.2. Reservoir Design Considerations
The design of the hydraulic reservoir is critical to the performance of the press. Key design considerations include:
- Size and capacity: The reservoir must be large enough to store sufficient hydraulic fluid to keep the press operating smoothly. A well-sized reservoir also allows enough time for the fluid to cool before it is recirculated.
- Ventilation: Reservoirs are typically designed with vented lids or breathers to allow air to escape as the fluid level changes. Proper ventilation prevents pressure build-up and reduces the risk of contamination entering the system.
- Filtration: Many reservoirs are equipped with filters or strainers to remove contaminants from the fluid. Filtering the fluid before it is recirculated helps prevent damage to the hydraulic system and improves overall performance.
8.3. Cooling Systems
Hydraulic presses generate heat during operation, primarily due to the friction between moving parts and the compression of hydraulic fluid. If the fluid becomes too hot, it can degrade, reducing its effectiveness and causing damage to the hydraulic system. The cooling system is essential for maintaining optimal fluid temperature and ensuring the longevity of the press.
8.3.1. Types of Cooling Systems
There are several types of cooling systems used in hydraulic presses, each designed to manage the heat generated during operation.
- Air-Cooled Systems: In air-cooled systems, the hydraulic fluid is circulated through a radiator or cooling fins, where it is cooled by air flow. This type of cooling system is typically used in smaller presses or applications where heat generation is minimal.
- Advantages: Simple and cost-effective design. No need for additional coolant.
- Disadvantages: Less effective in high-temperature environments or for heavy-duty presses.
- Liquid-Cooled Systems: Liquid cooling systems use water or another coolant to absorb heat from the hydraulic fluid. The coolant circulates through a heat exchanger, where it transfers the heat away from the fluid. Liquid-cooled systems are more efficient than air-cooled systems and are typically used in larger or high-performance presses.
- Advantages: More effective at managing high heat loads. Suitable for heavy-duty and high-speed presses.
- Disadvantages: Requires more complex maintenance, including regular coolant checks and potential leaks.
8.3.2. Heat Exchangers
In many hydraulic presses, heat exchangers are used to transfer heat from the hydraulic fluid to the surrounding air or coolant. These devices are critical in maintaining the optimal operating temperature of the fluid, especially in large or high-speed presses where heat generation is significant.
- Plate heat exchangers: These devices use a series of metal plates to transfer heat between the hydraulic fluid and the coolant. Plate heat exchangers are compact and efficient, making them ideal for modern hydraulic systems.
- Shell-and-tube heat exchangers: These are larger, more industrial heat exchangers used in heavy-duty presses. They consist of a series of tubes through which the hydraulic fluid and coolant flow, transferring heat between them.
8.4. Reservoir and Cooling System Maintenance
Proper maintenance of the hydraulic reservoir and cooling system is essential for ensuring the longevity and performance of the press. Key maintenance tasks include:
8.4.1. Fluid Level Checks
The hydraulic fluid level in the reservoir should be checked regularly to ensure that there is always sufficient fluid to operate the press. Low fluid levels can lead to overheating, cavitation, and reduced system performance.
- Topping off fluid: If the fluid level is low, the reservoir should be topped off with the appropriate hydraulic fluid to prevent damage to the system.
8.4.2. Cleaning and Filtering
The reservoir and cooling system should be cleaned regularly to remove dirt, debris, and contaminants that can degrade the performance of the press. Filters and strainers should be checked and replaced as needed to ensure that the fluid remains clean and free of debris.
- Changing filters: Filters should be replaced at regular intervals, as recommended by the manufacturer. Dirty or clogged filters can reduce fluid flow and increase pressure, leading to system inefficiencies.
8.4.3. Coolant Checks
In liquid-cooled systems, the coolant should be checked regularly for proper levels and potential contamination. Leaks in the cooling system can lead to overheating, reducing the performance and lifespan of the press.
- Leak prevention: Inspecting hoses, connections, and heat exchangers for signs of leaks or damage is critical to maintaining the effectiveness of the cooling system.
8.5. Impact of Overheating and Fluid Degradation
Overheating can have a detrimental effect on the hydraulic fluid, causing it to degrade and lose its effectiveness. Thermal breakdown of the fluid can lead to reduced lubrication, increased wear on components, and eventual system failure. Regular maintenance of the reservoir and cooling system is essential to preventing overheating and ensuring that the press operates efficiently and reliably.
9. Seals and Gaskets (600 words)
Seals and gaskets play an essential role in maintaining the pressure and fluid integrity of a hydraulic press. These components ensure that the hydraulic fluid is contained within the system, preventing leaks and maintaining consistent pressure. Without effective seals and gaskets, the hydraulic system would suffer from pressure loss, contamination, and inefficient operation, which could lead to damage to the press and costly downtime.
9.1. Importance of Seals in Maintaining Fluid Pressure
The primary function of seals in a hydraulic press is to prevent hydraulic fluid from leaking out of the system. Hydraulic systems operate under extremely high pressure, and even a small leak can lead to significant pressure loss and reduced efficiency. Seals are used in various parts of the press, including the hydraulic cylinder, piston, and valves, to ensure that hydraulic fluid is contained and pressure is maintained.
Seals also play a secondary role in lubricating the moving parts of the press. Many seals are designed to retain a thin film of hydraulic fluid between the moving parts, reducing friction and wear. This ensures that components such as the piston and cylinder walls move smoothly and efficiently without excessive heat or damage.
9.2. Types of Seals Used in Hydraulic Presses
There are several types of seals used in hydraulic presses, each designed for specific functions and operating conditions. The most common types of seals include O-rings, U-cups, rod seals, and piston seals.
9.2.1. O-Rings
O-rings are the most common type of seal used in hydraulic systems. They are typically made from rubber or synthetic materials like Nitrile or Viton and are designed to sit in grooves between two surfaces, creating a tight seal to prevent fluid leakage.
- Applications: O-rings are commonly used in static applications, such as sealing the joints between two non-moving components.
- Advantages: They are inexpensive, easy to replace, and suitable for a wide range of operating pressures and temperatures.
9.2.2. U-Cups
U-cups are used in dynamic applications, such as sealing the piston or rod in a hydraulic cylinder. Their “U”-shaped design allows them to flex under pressure, providing a tight seal even when the piston or rod is in motion.
- Applications: U-cups are often found in the hydraulic cylinder and rod assemblies.
- Advantages: Their flexible design ensures a strong seal while allowing for the movement of components.
9.2.3. Rod Seals
Rod seals are used to seal the area where the piston rod exits the hydraulic cylinder. They prevent hydraulic fluid from leaking out of the cylinder and also keep contaminants, such as dirt or dust, from entering the system.
- Applications: Found in the rod assembly of hydraulic cylinders.
- Advantages: Rod seals ensure long-term performance by preventing leakage while protecting the cylinder from external contamination.
9.2.4. Piston Seals
Piston seals are used to seal the gap between the piston and the cylinder walls, ensuring that hydraulic fluid does not pass from one side of the piston to the other. Piston seals are critical in maintaining pressure within the cylinder and ensuring that the piston moves smoothly and efficiently.
- Applications: Installed on the piston head within hydraulic cylinders.
- Advantages: Provide a tight seal and reduce friction, improving the efficiency of the hydraulic press.
9.3. Seal Materials: Rubber, Polyurethane, and PTFE
Seals are typically made from materials that offer high durability, resistance to wear, and the ability to withstand the high pressures and temperatures found in hydraulic systems. The most common materials used in hydraulic seals include:
- Rubber: Rubber seals are widely used due to their flexibility, resilience, and affordability. However, rubber can degrade over time when exposed to extreme heat or certain types of hydraulic fluid.
- Polyurethane: Polyurethane seals are known for their excellent abrasion resistance and durability, making them ideal for applications where seals are subjected to significant wear.
- PTFE (Teflon): PTFE seals offer high resistance to heat and chemical degradation, making them ideal for use in hydraulic systems that operate at high temperatures or with aggressive fluids.
9.4. Common Seal Failures: Causes and Prevention
While seals are designed to last, they can wear out or fail over time, leading to leaks and reduced system performance. Common causes of seal failure include:
9.4.1. Wear and Tearing
Seals are subject to wear and tear due to friction between moving parts. Over time, this can cause seals to crack, degrade, or lose their flexibility, leading to leaks. Using high-quality materials and maintaining proper lubrication can help extend the life of seals.
9.4.2. Contamination
Contaminants such as dirt, dust, or metal particles can damage seals by causing them to wear out faster. Installing proper filters and regularly maintaining the hydraulic system can prevent contamination and reduce seal wear.
9.4.3. Improper Installation
Seals that are improperly installed can become pinched, twisted, or damaged during operation. Ensuring that seals are installed correctly and in the right position can prevent these issues.
9.5. Innovations in Seal Technology
Recent advances in seal technology have led to the development of self-lubricating seals and longer-lasting materials, which can help reduce maintenance costs and extend the lifespan of hydraulic presses.
- Self-lubricating seals: These seals are made from materials that naturally reduce friction between the moving parts, reducing wear and extending the life of the seal.
- Improved materials: Seals made from advanced materials such as PTFE or reinforced polyurethane offer better resistance to heat, wear, and chemical degradation, improving their durability in demanding applications.
Sensors, Gauges, and Monitoring Systems
The integration of sensors, gauges, and monitoring systems into hydraulic presses has revolutionized how these machines operate, providing real-time data on the press’s performance and condition. These components enhance the safety, accuracy, and efficiency of the press by monitoring key parameters such as pressure, temperature, and piston position. In this section, we will explore the role of sensors, gauges, and monitoring systems in hydraulic presses and how they improve the operation and maintenance of the machine.
10.1. Monitoring Systems in Hydraulic Presses
Monitoring systems in hydraulic presses track the system’s performance and provide real-time feedback to operators and control systems. These systems help detect potential issues before they lead to system failure, enabling predictive maintenance and reducing downtime. Monitoring systems also improve the precision of pressing operations by providing accurate data on force, pressure, and piston position.
10.2. Types of Sensors in Hydraulic Presses
Sensors are used throughout the hydraulic press to measure different parameters. The most common types of sensors include pressure sensors, temperature sensors, and position sensors.
10.2.1. Pressure Sensors
Pressure sensors measure the hydraulic pressure within the system, providing real-time data on the force being applied during pressing operations. Pressure sensors ensure that the press is operating within safe limits and help maintain consistent force application.
- Application: Pressure sensors are typically placed in the hydraulic cylinder or near the pump to monitor the fluid pressure.
10.2.2. Temperature Sensors
Temperature sensors monitor the temperature of the hydraulic fluid and other components to prevent overheating. If the fluid temperature rises too high, it can degrade the fluid and reduce the efficiency of the system.
- Application: Temperature sensors are often installed in the hydraulic reservoir or near heat-generating components such as the pump or cylinder.
10.2.3. Position Sensors
Position sensors track the movement and position of the piston within the hydraulic cylinder. These sensors help ensure that the press applies the correct amount of force over the desired distance, improving the accuracy and repeatability of pressing operations.
- Application: Position sensors are typically placed on the hydraulic cylinder to monitor the piston’s movement.
10.3. Pressure Gauges: Analog vs. Digital
Pressure gauges are used to visually monitor the hydraulic pressure within the system. Traditional analog gauges use mechanical components to display the pressure, while modern digital gauges provide more precise, real-time data.
- Analog gauges: Simple, reliable, and easy to read, analog gauges are commonly used in smaller or older presses.
- Digital gauges: Digital gauges provide more accurate readings and can be integrated with monitoring systems to provide real-time data for automated control.
10.4. Role of Monitoring Systems in Improving Performance and Safety
The integration of sensors and monitoring systems improves the safety, performance, and efficiency of hydraulic presses in several ways:
- Real-time feedback: Monitoring systems provide real-time data on key performance metrics, allowing operators to make adjustments on the fly and ensure consistent press operation.
- Predictive maintenance: By tracking parameters such as pressure, temperature, and component wear, monitoring systems can help operators schedule maintenance before issues lead to costly breakdowns.
- Improved accuracy: Position sensors and digital pressure gauges allow for more precise control over pressing operations, ensuring that the press applies the correct force and completes tasks with high accuracy.
10.5. Predictive Maintenance Using Real-Time Data
One of the most significant benefits of modern monitoring systems is their ability to facilitate predictive maintenance. By analyzing real-time data from sensors, operators can identify potential issues, such as fluid leaks, overheating, or pressure loss, before they lead to system failure.
- Predictive maintenance: This approach reduces the risk of unexpected downtime and extends the life of the press by addressing issues early. Monitoring data can also be used to schedule routine maintenance tasks based on the actual wear and condition of components, rather than following a fixed maintenance schedule.
Introduction to Hydraulic Presses
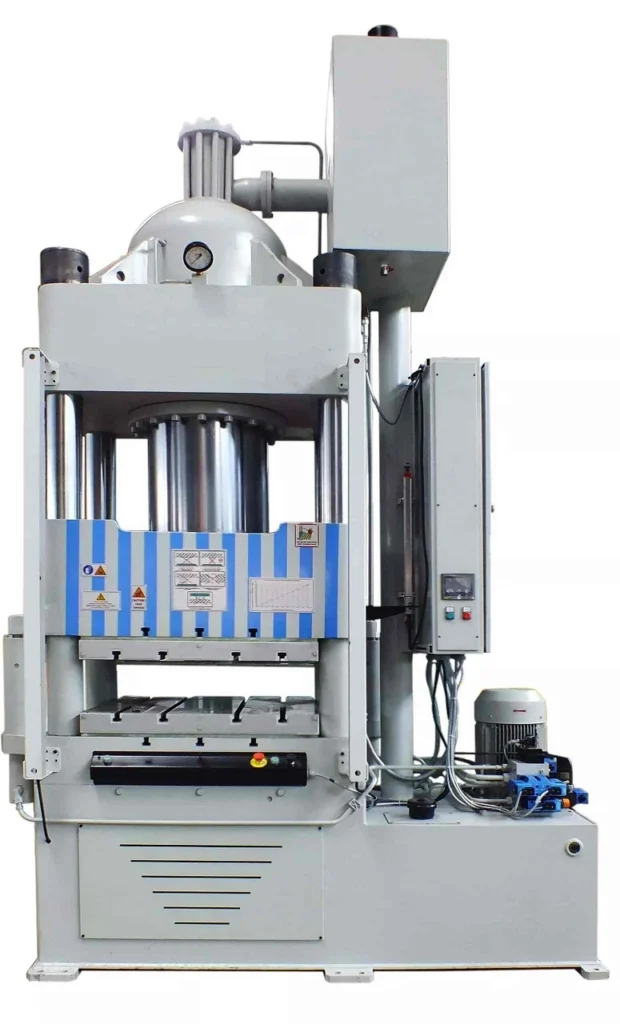
A hydraulic press is a mechanical device that uses hydraulic force to compress, shape, or mold materials into specific forms. It is widely regarded as one of the most important machines in industrial manufacturing due to its ability to apply immense force with precision and control. This force is generated by the hydraulic system, which operates based on Pascal’s Law, allowing a small input force to be multiplied into a much larger output force. Hydraulic presses are used in a variety of industries, including automotive manufacturing, aerospace, metalworking, and plastic molding, where they perform tasks such as metal forming, stamping, deep drawing, and forging.
The working principle of a hydraulic press revolves around the movement of hydraulic fluid under pressure, which is directed into a cylinder to push a piston. This piston, in turn, applies a compressive force on the material placed beneath it. By manipulating the size of the cylinder and piston, hydraulic presses can generate forces ranging from a few tons to thousands of tons, making them highly versatile machines.
Hydraulic presses are favored for their precision and control, enabling manufacturers to produce consistent, high-quality parts. They are also more compact compared to mechanical presses, and their force can be applied more evenly, which is particularly important in tasks requiring smooth, controlled deformation of materials.
This principle of multiplying force has applications far beyond presses. Hydraulic systems are used in a wide range of machinery and tools, including hydraulic lifts, jacks, and braking systems in vehicles. The versatility and efficiency of hydraulic systems make them a cornerstone of modern engineering and manufacturing.
Despite their widespread use and importance, the operation of hydraulic presses remains based on a simple concept—containing and controlling fluid pressure to achieve a desired mechanical outcome. The ability to harness the power of fluid dynamics enables hydraulic presses to perform complex and demanding tasks with minimal input force.
In the following sections, we will explore in detail the scientific principles that underpin hydraulic press operation, the components that make up these systems, and the various types of hydraulic presses used across industries. Additionally, we will delve into the energy efficiency, control mechanisms, and future innovations that continue to make hydraulic presses indispensable in the modern industrial landscape.
Fundamental Science Behind Hydraulic Presses
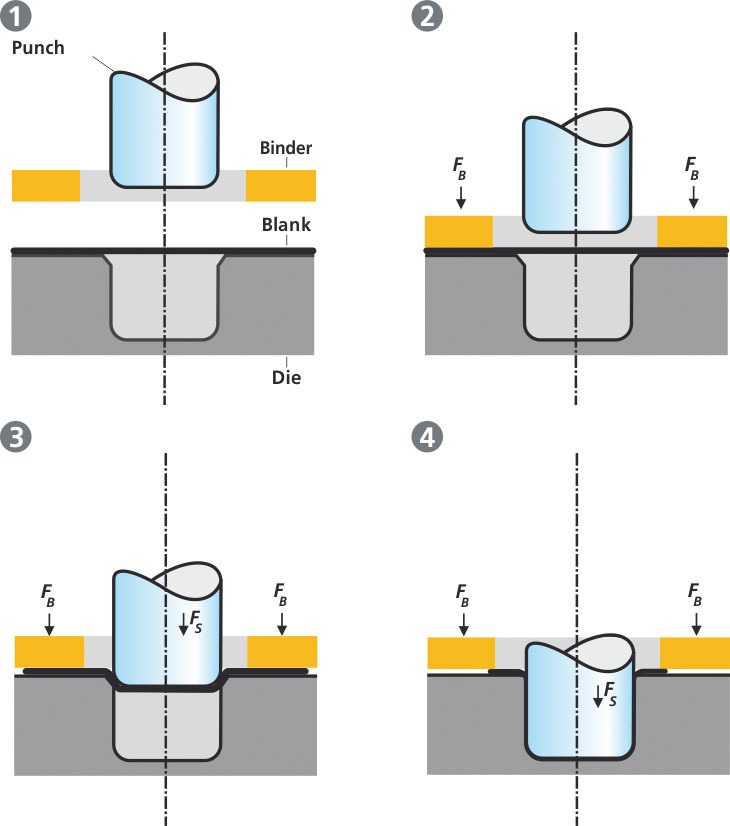
The working principle of a hydraulic press is based on the foundational concept of Pascal’s Law, which governs the behavior of fluids in confined spaces. Understanding the physics behind hydraulic systems is essential to grasp how hydraulic presses can generate such significant force with relatively little input.
2.1. Pascal’s Law and Its Application in Hydraulic Systems
Pascal’s Law, named after the French mathematician Blaise Pascal, states that when pressure is applied to a confined fluid, it is transmitted equally in all directions. This means that any change in pressure in one part of a confined fluid is reflected throughout the entire fluid. In a hydraulic press, this principle is used to multiply force and transfer it efficiently across a system.
The mathematical expression of Pascal’s Law is:P=FAP = \frac{F}{A}P=AF
Where:
- P is pressure,
- F is force, and
- A is the area over which the force is applied.
In a hydraulic press, this equation explains how a small input force can be transformed into a much larger output force. By increasing the area of the output piston while keeping the pressure constant, the force exerted by the press can be amplified. This is the fundamental principle behind force multiplication in hydraulic systems.
2.2. Force Multiplication in Hydraulic Systems
To understand how Pascal’s Law applies in a hydraulic press, consider a simple system with two connected cylinders of different sizes. A small input force is applied to the smaller piston (called the master cylinder), creating pressure in the hydraulic fluid. Because pressure is transmitted equally in all directions, this pressure is also applied to the larger piston (called the slave cylinder). Since the area of the larger piston is greater, the force it exerts is proportionally larger, even though the pressure in the system remains constant.
For example, if a 100 Newton force is applied to a small piston with an area of 1 square centimeter, the resulting pressure is 100 N/cm². If this pressure is applied to a larger piston with an area of 10 cm², the force exerted by the larger piston will be 100 N/cm² × 10 cm² = 1,000 Newtons. This ability to multiply force makes hydraulic systems incredibly powerful and efficient.
This concept is the core of how hydraulic presses operate: a small force applied at the input is transformed into a much larger force at the output, allowing for tasks such as metal forming, forging, stamping, and crushing to be carried out with great efficiency.
2.3. How Pressure is Distributed Through a Confined Fluid
In a hydraulic press, the hydraulic fluid plays a critical role in force transmission. Hydraulic fluids are generally incompressible, meaning they do not significantly change in volume when subjected to pressure. This property allows the fluid to transmit force consistently and efficiently.
When pressure is applied to the fluid, the fluid distributes that pressure equally in all directions within the confined space of the hydraulic cylinder. This even distribution is key to ensuring that the press applies uniform pressure across the material being worked on. Whether the press is used to form metal sheets, mold plastics, or perform deep drawing operations, the hydraulic fluid ensures that the force is applied consistently across the entire surface area of the workpiece.
In practical terms, this means that the hydraulic press can handle complex shapes and materials that require smooth, even deformation, such as in metal forming or precision assembly tasks.
2.4. Real-World Examples of Pascal’s Law Beyond Hydraulic Presses
While hydraulic presses are perhaps the most well-known application of Pascal’s Law, this principle is utilized in many other areas of engineering and machinery. Some real-world examples include:
- Hydraulic car jacks: Used to lift heavy vehicles with minimal input force. A small pump applies pressure to the hydraulic fluid, lifting the vehicle with the help of a larger piston.
- Hydraulic braking systems: Found in cars, trucks, and airplanes, hydraulic brakes use fluid pressure to multiply the force applied by the driver’s foot on the brake pedal, applying enough force to stop the vehicle.
- Hydraulic lifts: Commonly used in construction, warehouses, and factories, hydraulic lifts use Pascal’s Law to raise and lower heavy loads with great precision.
In each of these examples, the ability to multiply force using hydraulic systems allows for efficient operation of equipment, reducing the need for manual labor and increasing productivity.
2.5. Hydraulic Force vs. Mechanical Force
Hydraulic presses differ from mechanical presses in how they generate and apply force. While both types of presses are used to deform, shape, or compress materials, the mechanisms they use are quite different.
- Hydraulic presses use hydraulic fluid to apply pressure, allowing for smoother and more controlled force application. The force in a hydraulic press is applied uniformly, which is ideal for tasks that require precision, such as metal forming and plastic molding.
- Mechanical presses, on the other hand, rely on mechanical linkages and flywheels to generate force. While mechanical presses can be faster and are suitable for repetitive tasks like stamping, they are less versatile when it comes to handling complex shapes or materials.
The key advantage of hydraulic presses is their ability to apply constant pressure over a long stroke length. This makes them ideal for tasks like deep drawing, metal extrusion, and compression molding, where materials need to be shaped gradually and with high precision.
Components of a Hydraulic Press and Their Roles in the Working Principle
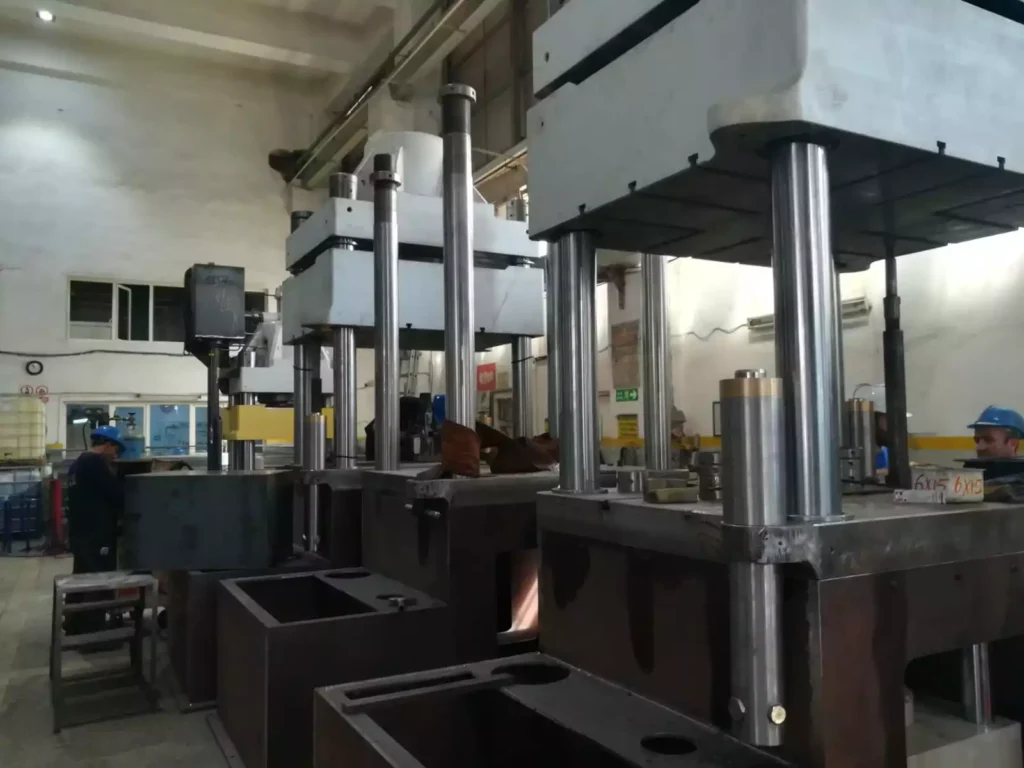
A hydraulic press consists of several key components, each of which plays an essential role in converting hydraulic energy into mechanical force. Understanding how these components function and interact with each other is crucial to comprehending the overall working principle of hydraulic presses. In this section, we will explore the most important components of a hydraulic press and their specific contributions to the system’s operation.
3.1. Hydraulic Cylinder
The hydraulic cylinder is the heart of the hydraulic press. It is responsible for converting hydraulic pressure into linear mechanical force, which is then used to deform or shape the material. The cylinder houses the piston, and the pressurized hydraulic fluid is pumped into the cylinder, creating pressure behind the piston. This pressure causes the piston to move, applying force to the material beneath the press.
Types of Hydraulic Cylinders
Hydraulic cylinders come in different designs depending on the application of the press:
- Single-acting cylinders: These cylinders have hydraulic fluid applied to only one side of the piston, causing the piston to move in one direction. The return stroke is powered by external forces, such as springs or gravity.
- Double-acting cylinders: In these cylinders, hydraulic fluid is applied to both sides of the piston, allowing it to move in both directions. This design offers more control over the movement of the piston and is commonly used in modern hydraulic presses that require precision and speed.
Function of the Cylinder in the Hydraulic Press
The force generated by the hydraulic press is proportional to the surface area of the cylinder and the pressure applied to the hydraulic fluid. This makes the cylinder’s design critical to determining how much force the press can exert. Larger cylinders can generate more force due to the increased area over which the hydraulic fluid applies pressure.
In a typical operation cycle, the press’s hydraulic pump forces fluid into the cylinder, pushing the piston downward. The material placed beneath the press is deformed or compressed as the piston moves. Once the task is completed, the hydraulic fluid is either released (in single-acting cylinders) or pumped back (in double-acting cylinders) to retract the piston.
3.2. Piston
The piston is another vital component of a hydraulic press, as it is responsible for transferring the hydraulic pressure into mechanical force. The piston moves within the hydraulic cylinder as pressurized fluid is pumped in, causing it to push down on the material being pressed. The force exerted by the piston depends on its size, the pressure of the hydraulic fluid, and the area over which the pressure is applied.
Piston’s Role in Force Transmission
In hydraulic systems, the piston acts as the intermediary between hydraulic pressure and mechanical action. The movement of the piston is what ultimately causes the deformation, compression, or shaping of the material. The larger the surface area of the piston, the greater the force it can exert. For example, a larger piston will apply a higher force to the material even with the same amount of hydraulic pressure.
Pistons are typically made from high-strength materials like steel or cast iron, ensuring they can withstand the immense pressures generated by the hydraulic system. To ensure smooth operation, pistons are often coated with chrome or other wear-resistant materials to reduce friction and prevent corrosion.
Precision and Control
Modern hydraulic presses rely on highly precise pistons that can handle extremely high loads while maintaining smooth, controlled movement. In presses used for precision tasks, such as in the automotive or aerospace industries, the piston’s movement must be carefully controlled to ensure that the material is shaped or pressed accurately. The responsiveness of the piston allows operators to achieve fine control over the force and speed of the press, ensuring high-quality results.
3.3. Hydraulic Fluid
Hydraulic fluid plays a critical role in the operation of a hydraulic press by serving as the medium through which force is transmitted. When hydraulic fluid is pressurized, it transmits this pressure to the piston, causing the piston to move. The properties of hydraulic fluid, such as viscosity, thermal stability, and compressibility, directly impact the performance and efficiency of the press.
Types of Hydraulic Fluids
Several types of hydraulic fluids are used in hydraulic presses, each with different properties suited to specific operating conditions:
- Mineral-based hydraulic fluids: These are the most common and cost-effective fluids used in general-purpose presses. They offer good lubrication and heat dissipation properties but may not perform well in extreme temperatures.
- Water-based hydraulic fluids: These fluids are used in applications where fire resistance is important. They are less flammable than mineral oils, making them suitable for presses used in environments with a higher risk of fire.
- Synthetic hydraulic fluids: These are high-performance fluids designed for extreme conditions, such as high temperatures or heavy loads. They offer superior stability and performance but are more expensive than other types of hydraulic fluids.
Role of Hydraulic Fluid in Energy Transmission
The hydraulic fluid is incompressible, which allows it to transmit force efficiently. When pressurized by the hydraulic pump, the fluid moves through the system and into the cylinder, where it applies pressure to the piston. This pressure causes the piston to move, exerting force on the material. The ability of hydraulic fluid to transmit force without significant loss is what makes hydraulic systems so efficient.
In addition to transmitting force, hydraulic fluid also acts as a lubricant, reducing friction between the moving parts of the press, such as the piston and cylinder walls. It also helps dissipate heat generated during operation, preventing the system from overheating and maintaining consistent performance.
3.4. Hydraulic Pump
The hydraulic pump is responsible for pressurizing the hydraulic fluid and driving the movement of the piston. It converts mechanical energy (from a motor or engine) into hydraulic energy by moving the fluid through the system. The pump is a critical component in determining the overall performance and speed of the hydraulic press, as it directly affects how quickly pressure builds up in the system.
Types of Hydraulic Pumps
Hydraulic presses use several types of pumps, depending on the press’s size, application, and desired performance:
- Gear pumps: These are simple, cost-effective pumps that are commonly used in smaller presses or low-pressure applications. They are less efficient than other types of pumps but are reliable and easy to maintain.
- Vane pumps: Known for their quiet operation and smooth fluid flow, vane pumps are used in presses that require moderate pressure and efficiency.
- Piston pumps: These are the most powerful and efficient hydraulic pumps, capable of generating extremely high pressures. Piston pumps are typically used in heavy-duty industrial presses that require precise control and high force.
Pump’s Role in Generating Pressure
The hydraulic pump draws fluid from the reservoir and forces it into the hydraulic system, creating pressure. This pressure is what drives the movement of the piston, allowing the press to apply force to the material. The pump’s efficiency and capacity determine how quickly pressure builds up in the system and how much force the press can generate.
Modern hydraulic pumps are often designed to adjust their output based on the press’s operating conditions. For example, variable displacement pumps can change the amount of fluid they move depending on the press’s needs, improving energy efficiency and reducing waste.
3.5. Valves and Controls
Valves and control systems in a hydraulic press manage the flow of hydraulic fluid and ensure that pressure is applied safely and efficiently. Valves are used to control the direction, speed, and pressure of the fluid as it moves through the system.
Types of Valves
- Directional control valves: These valves control the direction of the hydraulic fluid, determining whether the piston moves up or down. They are essential for controlling the operation of the press.
- Pressure relief valves: These valves protect the system from over-pressurization by releasing excess fluid back into the reservoir when the pressure exceeds a safe limit.
- Flow control valves: These valves regulate the speed of the press by controlling the flow rate of hydraulic fluid into the cylinder. This allows operators to adjust the press’s speed for different tasks.
Control Systems and Automation
In modern hydraulic presses, valves are often controlled electronically, using Programmable Logic Controllers (PLCs) or Computer Numerical Control (CNC) systems. These control systems allow operators to automate the press’s operation, adjusting parameters such as pressure, speed, and stroke length with high precision.
Automation improves the efficiency and accuracy of hydraulic presses, making them suitable for tasks that require repetitive or precise operations. Smart control systems also enable real-time monitoring of the press’s performance, helping operators identify potential issues before they cause downtime or damage.
3.6. Reservoir and Cooling System
The reservoir stores the hydraulic fluid when it is not in use and provides a place for the fluid to cool before it is recirculated back into the system. The reservoir plays an important role in maintaining the efficiency and longevity of the hydraulic press by ensuring that the fluid remains clean and at an optimal temperature.
Reservoir’s Role in Fluid Management
The hydraulic fluid in the system heats up during operation due to friction and pressure. The reservoir allows the fluid to cool before it is pumped back into the system, preventing overheating and maintaining consistent pressure. In addition, the reservoir often contains filters that remove impurities from the fluid, preventing contamination that could damage the hydraulic components.
Cooling Systems
Some hydraulic presses are equipped with cooling systems, such as heat exchangers or radiators, to further control the temperature of the hydraulic fluid. Keeping the fluid at the correct temperature is essential for maintaining the press’s performance and preventing premature wear on components.
Pascal’s Law in Detail
Pascal’s Law is the fundamental scientific principle behind the operation of hydraulic presses. It explains how hydraulic systems can efficiently multiply force, making hydraulic presses capable of performing heavy-duty tasks such as forming, molding, and cutting with minimal input force. In this section, we will delve deeper into the mathematical foundation of Pascal’s Law, explore the concept of force multiplication in hydraulic systems, and discuss how pressure is transmitted through hydraulic fluid. Understanding Pascal’s Law is crucial to appreciating the inner workings and efficiency of hydraulic presses.
4.1. Mathematics of Pascal’s Law
Pascal’s Law, formulated by the French mathematician Blaise Pascal in the 17th century, states that when pressure is applied to a confined fluid, it is transmitted equally in all directions within the fluid. This simple yet powerful concept is the key to understanding how hydraulic systems work.
The equation for Pascal’s Law can be expressed as:P=FAP = \frac{F}{A}P=AF
Where:
- P is the pressure in the system (measured in Pascals, Pa),
- F is the force applied (measured in Newtons, N), and
- A is the area over which the force is applied (measured in square meters, m²).
This equation shows that pressure in a hydraulic system is a function of the force applied to the fluid and the area over which that force is distributed. By rearranging the formula to solve for force, we get:F=P×AF = P \times AF=P×A
This tells us that the force generated by the hydraulic system is directly proportional to the pressure applied and the surface area of the piston or cylinder. This relationship allows hydraulic presses to generate enormous forces with relatively small input pressures, making them highly efficient and powerful machines.
4.2. Pressure and Force Distribution
In a hydraulic press, Pascal’s Law ensures that the pressure applied to the hydraulic fluid is transmitted uniformly throughout the system. This means that the force generated by the press is distributed evenly across the entire surface area of the piston, resulting in uniform force application to the material being worked on.
For example, consider a hydraulic system with two pistons: a smaller input piston and a larger output piston. When pressure is applied to the input piston, the hydraulic fluid transmits that pressure to the larger output piston. Since pressure is constant throughout the fluid, the larger piston generates a much greater force because it has a larger surface area over which the pressure is applied.
If the input piston has an area of 1 square centimeter and the output piston has an area of 10 square centimeters, the force exerted by the output piston will be 10 times greater than the force applied to the input piston. This is known as force multiplication, and it is the reason why hydraulic presses can generate such large forces with minimal input effort.
The concept of force multiplication can be further illustrated using the example of a car jack. When you pump the handle of a hydraulic jack, you are applying a small force to a small piston. The hydraulic fluid then transmits this pressure to a larger piston, which exerts enough force to lift a car. The principle at work is the same as in a hydraulic press: pressure is distributed evenly, and the force is multiplied based on the difference in piston areas.
4.3. Formula for Force Multiplication
To better understand the concept of force multiplication, let’s break down the calculation:
Consider a hydraulic press with an input piston that has an area of 2 cm² and an output piston with an area of 50 cm². If you apply a force of 100 Newtons to the input piston, we can calculate the pressure applied to the hydraulic fluid using the following formula:P=FA=100 N2 cm2=50 N/cm2P = \frac{F}{A} = \frac{100 \, \text{N}}{2 \, \text{cm}^2} = 50 \, \text{N/cm}^2P=AF=2cm2100N=50N/cm2
This pressure is transmitted equally throughout the hydraulic fluid, so the same pressure (50 N/cm²) is applied to the output piston. To calculate the force exerted by the output piston, we multiply the pressure by the area of the output piston:Foutput=P×Aoutput=50 N/cm2×50 cm2=2500 NF_{\text{output}} = P \times A_{\text{output}} = 50 \, \text{N/cm}^2 \times 50 \, \text{cm}^2 = 2500 \, \text{N}Foutput=P×Aoutput=50N/cm2×50cm2=2500N
In this example, a small input force of 100 N is multiplied into a much larger output force of 2500 N. This illustrates how hydraulic presses can generate extremely high forces by applying relatively small forces at the input.
The ratio of the areas of the pistons is what allows the system to multiply force. In this case, the ratio of the areas is 50 cm² (output piston) to 2 cm² (input piston), or 25:1. This means that for every 1 unit of force applied to the input piston, 25 units of force are generated at the output piston.
4.4. Pressure Applied to Different Pistons
In hydraulic systems, pressure is the same throughout the fluid, regardless of the size of the pistons. However, the force generated at each piston varies depending on the surface area of the piston. This is what allows hydraulic presses to achieve force multiplication.
Let’s take another example where the hydraulic press has two pistons—an input piston with an area of 5 cm² and an output piston with an area of 25 cm². If a force of 200 N is applied to the input piston, the pressure in the system will be:P=FinputAinput=200 N5 cm2=40 N/cm2P = \frac{F_{\text{input}}}{A_{\text{input}}} = \frac{200 \, \text{N}}{5 \, \text{cm}^2} = 40 \, \text{N/cm}^2P=AinputFinput=5cm2200N=40N/cm2
This pressure is transmitted to the output piston, and the force generated by the output piston will be:Foutput=P×Aoutput=40 N/cm2×25 cm2=1000 NF_{\text{output}} = P \times A_{\text{output}} = 40 \, \text{N/cm}^2 \times 25 \, \text{cm}^2 = 1000 \, \text{N}Foutput=P×Aoutput=40N/cm2×25cm2=1000N
As seen here, the output force is five times greater than the input force because the area of the output piston is five times larger than the area of the input piston. This proportional relationship between piston area and output force is the foundation of the hydraulic press’s ability to generate large amounts of force.
4.5. Hydraulic Press Efficiency
One of the key benefits of hydraulic presses is their efficiency in converting input force into output force. Hydraulic systems are typically more efficient than mechanical systems because they transmit force directly through the incompressible fluid. This allows hydraulic presses to maintain consistent pressure and smooth force application over long distances, making them ideal for tasks that require precision and uniformity.
However, the overall efficiency of a hydraulic press depends on several factors, including:
- Hydraulic fluid properties: The viscosity and compressibility of the hydraulic fluid can affect how efficiently pressure is transmitted. High-quality hydraulic fluids with low compressibility are essential for maintaining efficient operation.
- System leaks: Leaks in the hydraulic system can result in pressure loss, reducing the efficiency of the press. Proper sealing and regular maintenance are necessary to prevent fluid leaks.
- Friction and heat: Friction between moving parts can generate heat, which reduces the overall efficiency of the system. Proper lubrication and cooling systems are needed to minimize friction and prevent overheating.
Hydraulic presses are particularly efficient when performing tasks such as deep drawing, forging, and stamping, where smooth and consistent force application is required over extended periods. The ability of hydraulic systems to maintain constant pressure ensures that the material is shaped uniformly, resulting in high-quality products with minimal defects.
4.6. Real-World Applications of Pascal’s Law in Hydraulic Presses
The principles of Pascal’s Law are applied in various industries where hydraulic presses are used to perform tasks that require large amounts of force. Some common applications of hydraulic presses include:
- Automotive manufacturing: Hydraulic presses are used to shape metal parts such as car body panels, engine components, and chassis frames.
- Aerospace: Hydraulic presses are essential for manufacturing high-strength metal parts used in aircraft, such as wing components, landing gear, and turbine blades.
- Metalworking: Hydraulic presses are used in metal forming, stamping, forging, and extrusion processes to shape and mold metals into complex shapes.
- Plastic molding: Hydraulic presses are used in the plastic molding industry to shape plastic materials into various products, such as automotive interiors, packaging, and consumer goods.
- Recycling: Hydraulic presses are used to compact scrap metal, plastics, and other materials into dense bales for easy transportation and recycling.
Working Process of a Hydraulic Press
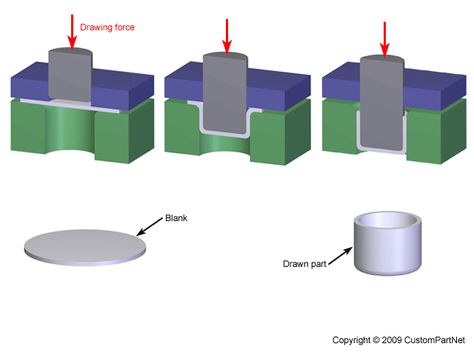
The working process of a hydraulic press is a well-orchestrated mechanical and fluid dynamic cycle. It involves the application of pressure through a hydraulic system, causing a piston to move, which in turn applies force to the material being worked on. The entire process revolves around the efficient transmission of force through hydraulic fluid, ensuring that large forces can be applied to the material with precision and control. This section will provide a step-by-step explanation of how a hydraulic press operates, from the input of hydraulic pressure to the final force application on the material.
5.1. Basic Operation Cycle of a Hydraulic Press
The operation of a hydraulic press involves several key steps, each of which plays a vital role in shaping or compressing the material being processed. The entire cycle can be divided into three primary stages: fluid pressurization, piston movement, and material deformation.
Step 1: Pressurizing the Hydraulic Fluid
The first step in the operation of a hydraulic press is pressurizing the hydraulic fluid, which is stored in a reservoir. The hydraulic pump is responsible for drawing the fluid from the reservoir and pressurizing it. This pump can be powered by an electric motor or a pneumatic system, depending on the design of the press.
As the hydraulic pump operates, it forces the hydraulic fluid into the hydraulic cylinder. This fluid, being incompressible, transmits the pressure evenly throughout the cylinder. The pressure applied to the fluid is what creates the force needed to move the piston.
- Hydraulic fluid pressurization: The pump applies mechanical energy to the fluid, increasing its pressure. The pressure level depends on the type of press and the amount of force required for the task. For example, in high-force applications such as forging or deep drawing, the pressure might reach thousands of PSI (pounds per square inch).
Step 2: Piston Movement
Once the hydraulic fluid is pressurized, the next step is the movement of the piston within the hydraulic cylinder. The pressurized fluid pushes against the surface area of the piston, causing it to move downward (in the case of a vertical press) or in another direction, depending on the press design.
As the piston moves, it transfers the hydraulic force to the material placed on the press’s bed or anvil. The force is applied uniformly across the material, enabling tasks such as compression, bending, cutting, or molding.
- Control over piston movement: The movement of the piston is controlled by directional control valves, which determine whether the fluid enters one side of the piston or the other. For presses that require precision, the flow control valves can also regulate the speed at which the piston moves, ensuring smooth and controlled operation.
- Force generation: The force applied by the piston is proportional to the pressure in the hydraulic system and the surface area of the piston. Larger pistons with greater surface areas generate more force, making hydraulic presses highly efficient for heavy-duty applications like metal stamping or forging.
Step 3: Material Deformation
The final stage in the operation of a hydraulic press is the deformation of the material being processed. As the piston applies force to the material, the material is compressed, bent, or shaped according to the specific requirements of the task.
- Material behavior under pressure: Different materials respond to pressure in various ways. Metals, for example, deform plastically when subjected to enough force, allowing them to be shaped into desired forms without breaking. Plastics, on the other hand, may require less force but must be handled carefully to avoid cracking or warping.
- Even force distribution: One of the advantages of hydraulic presses is their ability to apply force evenly across the surface of the material. This even distribution of force ensures that the material is deformed consistently, reducing the risk of defects such as uneven thickness, cracks, or warping.
Once the task is complete, the hydraulic system reduces the pressure, and the piston retracts, either by releasing the hydraulic fluid or by using a return spring (in single-acting cylinders) or applying pressure to the opposite side of the piston (in double-acting cylinders). This completes the operational cycle.
5.2. Input Force to Output Force: A Detailed Breakdown
One of the key principles behind the working process of a hydraulic press is the multiplication of force. Hydraulic presses are designed to take a small input force and amplify it into a much larger output force, thanks to the mechanics of Pascal’s Law and the difference in surface areas between the input and output pistons.
Let’s break down this process in more detail:
- Small input force: In a hydraulic press, the operator or an automated system applies a relatively small input force to the hydraulic fluid, either by operating the hydraulic pump or using a manual lever (in the case of manual presses).
- Pressure generation: This input force creates pressure within the hydraulic fluid, which is then transmitted through the system. Since the fluid is incompressible, the pressure is distributed evenly throughout the hydraulic system.
- Force amplification: The force applied by the piston to the material is determined by the surface area of the piston and the pressure in the hydraulic system. Because the piston typically has a much larger surface area than the input mechanism, the force exerted by the piston is amplified many times over.
For example, if a hydraulic press has an input piston with an area of 2 square centimeters and an output piston with an area of 50 square centimeters, the output force will be 25 times greater than the input force. This ability to multiply force is what makes hydraulic presses so effective in tasks that require immense force, such as forging, stamping, or metal forming.
5.3. Stroke Length and Speed Control
The stroke length refers to the distance the piston travels during the operation of the hydraulic press. Stroke length is an important parameter in press operation because it determines how far the material is compressed, bent, or shaped. In many applications, precise control over stroke length is necessary to ensure that the material is processed correctly.
- Adjusting stroke length: Hydraulic presses are typically equipped with controls that allow operators to adjust the stroke length. For example, when pressing large or thick materials, the stroke length may need to be longer to ensure full compression. In contrast, for smaller, more delicate tasks, a shorter stroke length is often sufficient.
- Speed control: The speed at which the piston moves is another critical factor in the operation of a hydraulic press. The speed is controlled by regulating the flow rate of the hydraulic fluid through the system. Flow control valves are used to adjust the fluid flow, allowing operators to slow down or speed up the piston’s movement based on the requirements of the task.
In applications that require precision forming, such as in the automotive or aerospace industries, the ability to control the speed of the press is essential. Slower speeds allow for greater precision and help avoid defects, while faster speeds increase productivity when handling simpler tasks.
5.4. Reverse Stroke: The Return Process
In addition to the pressing stroke, hydraulic presses also have a reverse stroke, where the piston is retracted after the pressing operation is complete. The reverse stroke is essential for resetting the press so that the next operation can be performed.
Single-Acting Cylinders
In hydraulic presses with single-acting cylinders, the piston is powered by hydraulic pressure in only one direction—typically the downward stroke. After the pressing operation is completed, the piston is returned to its starting position using either a return spring or gravity. These systems are simpler but may not offer as much control over the reverse stroke.
- Return spring: A spring attached to the piston helps pull it back to the starting position once the hydraulic pressure is released.
- Gravity return: In vertical presses, the weight of the piston itself can help it return to the starting position.
Double-Acting Cylinders
In double-acting cylinders, hydraulic pressure is applied to both sides of the piston, allowing for controlled movement in both directions. This means the piston can be powered both during the pressing stroke and the reverse stroke. Double-acting cylinders are more versatile and allow for greater control over the reverse stroke, making them ideal for precision tasks.
- Hydraulic return: Pressure is applied to the opposite side of the piston to move it back to its starting position after the operation. This offers more control and ensures that the piston retracts at a consistent speed.
5.5. Hydraulic Press Cycle Time
The cycle time of a hydraulic press refers to the amount of time it takes to complete one full operation, including the pressing stroke, the reverse stroke, and the resetting of the press. Several factors affect the cycle time, including:
- Hydraulic pump capacity: The pump’s ability to generate pressure quickly can reduce the cycle time. High-capacity pumps allow for faster pressurization of the hydraulic fluid, resulting in quicker piston movement.
- Piston speed: The speed at which the piston moves during both the pressing and reverse strokes also affects the cycle time. Flow control valves help regulate this speed to achieve the desired balance between precision and productivity.
- Material properties: The material being pressed can impact cycle time. Harder materials, such as metals, may require more time for compression, while softer materials like plastics can be processed more quickly.
- Stroke length: Longer strokes require more time to complete, extending the overall cycle time. Shorter strokes are faster but may not be suitable for all applications.
In high-volume production environments, minimizing cycle time is crucial to maintaining efficiency and productivity. Modern hydraulic presses are designed with automated controls and efficient hydraulic systems to optimize cycle time while maintaining high levels of precision and quality.
Types of Hydraulic Presses and Their Variations in Working Principles
Hydraulic presses come in a wide variety of designs, each tailored to specific applications and industries. While the basic principle of operation—applying hydraulic pressure to a piston to generate force—remains the same, the differences in frame construction, force output, and functionality make each type of hydraulic press suited for particular tasks. In this section, we will explore several common types of hydraulic presses and the variations in their working principles.
6.1. Manual Hydraulic Presses
Manual hydraulic presses are operated by hand, typically using a lever or hand pump to generate hydraulic pressure. These presses are simple in design and do not require external power sources like electricity or pneumatic systems. Manual presses are most commonly used for small-scale tasks where precision and high force are needed but speed is not a priority.
Working Principle of Manual Hydraulic Presses
- Hand-powered pump: In a manual press, the operator applies force to a lever or hand pump, which drives the hydraulic fluid into the cylinder. This creates pressure in the hydraulic system and moves the piston to apply force to the material.
- Force application: Despite being manually powered, these presses can generate significant force, thanks to Pascal’s Law. The operator can apply a relatively small amount of force, which is multiplied through the hydraulic system, allowing for tasks like pressing bearings, small metal forming, or assembly work.
- Control: Manual presses allow for precise control over the amount of force applied, as the operator can stop or adjust the lever or pump as needed. However, the process can be slow compared to automated systems.
Applications
- Workshops and laboratories: Manual hydraulic presses are often found in small workshops and laboratories where precision and control are more important than speed. They are used for tasks such as press fitting, bearing insertion, jewelry making, and small metal forming.
- Low-volume production: These presses are ideal for low-volume or one-off production runs, as they are cost-effective and easy to operate.
Advantages
- Portability: Manual hydraulic presses are often compact and portable, making them easy to move and set up in different locations.
- Low cost: They are more affordable than powered hydraulic presses, making them ideal for smaller operations or hobbyists.
- Precision: The operator has full control over the amount of force applied, allowing for precise and careful operations.
Limitations
- Limited force: While manual presses can generate significant force, they are limited in comparison to powered hydraulic presses. They are not suitable for tasks requiring very high pressure or force.
- Slower operation: Manual presses are slower than powered presses, as the operator must manually pump the hydraulic fluid into the cylinder.
6.2. Powered Hydraulic Presses
Powered hydraulic presses use an external power source, such as an electric motor or pneumatic system, to drive the hydraulic pump. This allows for faster and more consistent operation compared to manual presses, making them ideal for industrial applications that require high force and rapid cycle times.
Electric Hydraulic Presses
Electric hydraulic presses are driven by electric motors that power the hydraulic pump, generating fluid pressure automatically. These presses are commonly used in large-scale manufacturing environments where high force and precision are required.
Working Principle of Electric Hydraulic Presses
- Electric motor: The electric motor drives the hydraulic pump, which pressurizes the hydraulic fluid. The pressurized fluid is then directed into the cylinder, moving the piston and applying force to the material.
- Automated control: Electric presses often feature programmable controls, allowing operators to set specific parameters such as pressure, stroke length, and speed. This automation improves consistency and reduces operator error.
- Continuous operation: Unlike manual presses, electric hydraulic presses can operate continuously, making them suitable for high-volume production environments.
Applications
- Metal forming and stamping: Electric hydraulic presses are widely used in the metalworking industry, where they perform tasks such as metal stamping, bending, and punching.
- Plastic molding: These presses are also used in the plastic molding industry, where they shape plastic materials into various components.
- Automotive manufacturing: Electric hydraulic presses are essential in automotive production, where they are used to press car body panels, engine components, and structural elements.
Advantages
- High force output: Electric presses can generate much higher force than manual presses, making them suitable for heavy-duty applications.
- Precision and automation: Programmable controls allow for precise force application, ensuring consistency and reducing the need for manual intervention.
- Faster cycle times: Electric presses operate more quickly than manual presses, allowing for higher productivity in industrial settings.
Limitations
- Higher cost: Electric hydraulic presses are more expensive than manual presses, both in terms of initial investment and ongoing energy costs.
- Maintenance: These presses require more maintenance due to their complex electrical and hydraulic systems.
Pneumatic Hydraulic Presses
Pneumatic hydraulic presses are driven by compressed air, which is used to power the hydraulic pump. These presses are often used in light industrial applications where lower force is required, but speed and efficiency are still important.
Working Principle of Pneumatic Hydraulic Presses
- Compressed air: The press is powered by an air compressor, which provides the energy needed to drive the hydraulic pump. The compressed air creates pressure in the hydraulic fluid, which moves the piston and applies force to the material.
- Faster operation: Pneumatic presses are known for their quick operation, making them suitable for tasks that require rapid cycle times.
Applications
- Light assembly work: Pneumatic hydraulic presses are often used for assembly line tasks such as pressing bearings, fasteners, and small components into place.
- Low to moderate force applications: These presses are ideal for tasks that require moderate force, such as plastic forming, light metal forming, and punching.
Advantages
- Energy efficiency: Pneumatic presses are more energy-efficient for light-duty tasks, as they consume less power than electric presses.
- Fast operation: These presses offer rapid cycle times, making them ideal for repetitive tasks in assembly lines.
- Environmentally friendly: Pneumatic systems are often considered environmentally friendly, as they use compressed air instead of electricity.
Limitations
- Lower force output: Pneumatic presses cannot generate the same level of force as electric hydraulic presses, limiting their use in heavy-duty applications.
- Air consumption: They require a constant supply of compressed air, which may not be practical in all environments.
6.3. H-Frame Hydraulic Presses
H-frame hydraulic presses are named for their distinctive frame shape, which resembles the letter “H.” This frame design provides excellent stability and force distribution, making H-frame presses one of the most versatile and widely used types of hydraulic presses in manufacturing and repair applications.
Working Principle of H-Frame Hydraulic Presses
- H-frame structure: The press consists of two vertical columns (the legs of the “H”) connected by horizontal crossbars. The hydraulic cylinder and piston are mounted in the center of the crossbar, allowing the piston to move vertically and apply force to the material placed between the columns.
- High force capacity: H-frame presses are capable of generating large amounts of force, making them suitable for tasks such as metal forming, stamping, bending, and forging. The frame design ensures that the force is evenly distributed across the material, preventing deformation or misalignment.
- Manual or powered operation: H-frame presses can be operated manually, with a hand pump, or powered by an electric or pneumatic system, depending on the size and force requirements.
Applications
- Metalworking and fabrication: H-frame hydraulic presses are commonly used in the metalworking industry for tasks such as bending, straightening, stamping, and forming metal components.
- Automotive repair: These presses are often found in automotive repair shops, where they are used for tasks such as pressing bearings, straightening chassis components, and forming metal parts.
- General manufacturing: H-frame presses are versatile machines used in a wide range of manufacturing industries, including construction, aerospace, and machinery production.
Advantages
- Versatility: H-frame presses can be used for a wide range of tasks, from small-scale repairs to large-scale industrial manufacturing.
- High stability: The H-frame design provides excellent structural stability, ensuring that the force is applied evenly and accurately.
- Customizability: H-frame presses can be customized with different hydraulic systems, bed sizes, and accessories to meet specific production needs.
Limitations
- Large footprint: H-frame presses require a significant amount of floor space, making them less suitable for small workshops or facilities with limited space.
- Heavier: These presses tend to be heavier and more difficult to move compared to other types of hydraulic presses.
6.4. C-Frame Hydraulic Presses
C-frame hydraulic presses are designed with an open-front structure that resembles the letter “C.” This design provides easy access to the work area, making C-frame presses ideal for tasks that require quick loading and unloading of materials. C-frame presses are commonly used for smaller-scale applications where precision and speed are important.
Working Principle of C-Frame Hydraulic Presses
- Open-front design: The C-frame design allows for easy access to the pressing area from three sides, making it easier to position and remove materials. The hydraulic cylinder is mounted at the top of the frame, with the piston moving downward to apply force to the material placed on the bed.
- Single-point force application: In C-frame presses, the force is applied at a single point directly beneath the piston. While this provides precise control, it can also result in uneven force distribution if the material is not properly aligned.
Applications
- Precision metalworking: C-frame presses are often used in precision metalworking tasks such as punching, stamping, and forming small metal components.
- Assembly line work: These presses are commonly found in assembly lines for tasks such as pressing fasteners or components into place, particularly in industries like electronics and automotive.
- Small part production: C-frame presses are ideal for producing small parts or components that require high precision and frequent handling.
Advantages
- Compact and space-efficient: C-frame presses have a smaller footprint than H-frame presses, making them ideal for smaller workshops or production lines with limited space.
- Easy access: The open-front design allows for quick and easy loading and unloading of materials, improving productivity in high-volume applications.
- Precision: These presses offer excellent precision, making them ideal for tasks that require careful control of force and alignment.
Limitations
- Lower force capacity: C-frame presses typically generate less force than H-frame presses, limiting their use in heavy-duty applications.
- Frame deformation risk: The open-front design can result in frame deformation under extreme loads, so these presses are best suited for lighter tasks.
Fluid Dynamics in Hydraulic Presses
Fluid dynamics is a critical aspect of hydraulic press operation, governing how hydraulic fluid behaves within the system and ensuring the efficient transmission of force from the hydraulic pump to the piston. The properties of hydraulic fluid—such as viscosity, compressibility, and temperature tolerance—play a significant role in the performance of the hydraulic press. Understanding the principles of fluid dynamics in hydraulic systems helps in optimizing press efficiency, minimizing losses, and maintaining consistent pressure during operation.
In this section, we will explore how hydraulic fluid behaves under pressure, the role of flow rate and pressure regulation, the importance of cooling systems, and the effects of fluid contamination on press performance.
7.1. Understanding Fluid Behavior in Hydraulic Systems
Hydraulic presses rely on hydraulic fluid to transmit force from the pump to the piston. The fluid must be incompressible and able to withstand high pressures without degrading or causing system failures. The key properties of hydraulic fluid, which directly impact the operation of the press, are viscosity, compressibility, and thermal stability.
Viscosity
Viscosity refers to the resistance of a fluid to flow. In hydraulic systems, the viscosity of the hydraulic fluid is crucial because it determines how easily the fluid can move through the system and how effectively it can transmit force.
- Low viscosity fluids: Fluids with low viscosity flow easily, which can reduce friction and allow for faster operation of the press. However, if the viscosity is too low, the fluid may not provide sufficient lubrication for the moving parts of the hydraulic system, leading to increased wear and tear.
- High viscosity fluids: Fluids with higher viscosity provide better lubrication, which helps protect the components of the hydraulic press from friction and wear. However, high-viscosity fluids flow more slowly, which can reduce the overall speed of the press and cause energy losses due to internal friction in the fluid.
Maintaining the optimal viscosity for the hydraulic fluid is essential for ensuring that the press operates efficiently. The viscosity of the fluid can change with temperature, so hydraulic systems often include temperature control features to keep the fluid within its optimal viscosity range.
Compressibility
Compressibility is the degree to which a fluid can be compressed when subjected to pressure. In hydraulic presses, the ideal hydraulic fluid is incompressible, meaning that it does not significantly change in volume when pressure is applied. Incompressibility allows the fluid to transmit pressure efficiently and consistently across the system.
- Incompressible fluids: When the hydraulic fluid is incompressible, the pressure generated by the pump is transmitted directly to the piston, allowing for smooth and precise operation. Most hydraulic fluids are designed to be nearly incompressible, which is why they are effective in generating and transmitting large forces.
- Compressible fluids: If the hydraulic fluid were compressible, it would absorb some of the pressure applied by the pump, leading to losses in force transmission. This would result in inefficiencies in the press’s operation and potential inconsistencies in the application of force to the material.
Thermal Stability
Hydraulic fluid absorbs heat generated by the operation of the press, particularly due to the friction between moving parts and the compression of fluid under pressure. Thermal stability refers to the fluid’s ability to maintain its properties, such as viscosity and pressure tolerance, even at elevated temperatures.
- Thermally stable fluids: Hydraulic fluids with high thermal stability can withstand high operating temperatures without degrading. This ensures that the fluid continues to transmit force effectively, even during prolonged use or under heavy loads.
- Degradation at high temperatures: If the hydraulic fluid is not thermally stable, it may break down at high temperatures, losing its viscosity and becoming less effective at transmitting pressure. This can lead to reduced performance, increased wear on components, and eventual system failure.
For presses that operate at high speeds or under heavy loads, it is important to use hydraulic fluids that can tolerate high temperatures without losing their essential properties.
7.2. Flow Rate and Pressure Regulation
The flow rate and pressure of hydraulic fluid within the system are two of the most important factors that determine the performance of a hydraulic press. The flow rate affects the speed at which the piston moves, while the pressure determines how much force is applied to the material. Proper regulation of both flow and pressure is critical to ensuring that the press operates efficiently and effectively.
Flow Control in Hydraulic Presses
The flow rate of hydraulic fluid refers to how quickly the fluid moves through the system. Flow rate is typically measured in liters per minute (LPM) or gallons per minute (GPM), and it directly influences the speed of the piston.
- High flow rate: A higher flow rate allows the hydraulic press to complete its stroke more quickly, increasing the speed of operation. This is particularly useful in high-volume production environments where short cycle times are essential.
- Low flow rate: Lower flow rates result in slower piston movement, which can be beneficial in applications that require precise control or gradual deformation of the material, such as deep drawing or precision molding.
Flow control is managed by flow control valves, which adjust the rate at which hydraulic fluid is supplied to the cylinder. These valves allow operators to regulate the speed of the press, ensuring that the flow rate is appropriate for the task at hand. For example, tasks that require high precision may benefit from slower piston movement, while tasks that prioritize speed over precision may require higher flow rates.
Pressure Regulation in Hydraulic Presses
The pressure in a hydraulic system is what generates the force that moves the piston and applies pressure to the material. Pressure is measured in pounds per square inch (PSI) or bars, and it determines the amount of force that the hydraulic press can exert.
- High pressure: Higher pressures result in greater force being applied by the piston, allowing the press to handle heavy-duty tasks such as forging, metal forming, and cutting.
- Low pressure: Lower pressures may be used for tasks that require less force, such as assembly work, light metal forming, or plastic molding.
Pressure regulation is achieved using pressure control valves, including pressure relief valves and pressure-reducing valves. These valves ensure that the hydraulic system maintains a safe and consistent pressure level, preventing over-pressurization that could damage the press or the material being worked on.
- Pressure relief valves: These valves open when the pressure in the system exceeds a certain limit, allowing excess fluid to return to the reservoir. This prevents the system from becoming over-pressurized, which could lead to component failure or safety hazards.
- Pressure-reducing valves: These valves reduce the pressure to a specific level required for certain tasks. For example, when working with delicate materials that cannot withstand high pressure, a pressure-reducing valve can lower the force applied by the press to avoid damaging the material.
7.3. Heat Generation and Fluid Cooling
Hydraulic systems generate heat as a result of fluid compression, friction between moving parts, and resistance within the hydraulic lines. If the hydraulic fluid becomes too hot, its viscosity may decrease, leading to reduced efficiency and potential damage to the hydraulic components. Cooling systems are therefore an essential part of most hydraulic presses, ensuring that the fluid remains at an optimal temperature during operation.
Heat Generation in Hydraulic Systems
Several factors contribute to heat generation in hydraulic presses:
- Fluid compression: When hydraulic fluid is pressurized, it generates heat. This is particularly true in high-force applications where the fluid is subjected to extreme pressures.
- Friction: As the hydraulic fluid moves through the system, it encounters friction between the various components, including valves, hoses, and cylinders. This friction generates heat, particularly in systems that operate at high speeds.
- Energy losses: Some of the energy applied to the hydraulic fluid is lost in the form of heat due to internal resistance within the fluid. This is why hydraulic systems are often equipped with cooling systems to dissipate the heat and maintain fluid performance.
Cooling Systems for Hydraulic Presses
To prevent the hydraulic fluid from overheating, most hydraulic presses are equipped with cooling systems. These systems help regulate the temperature of the hydraulic fluid, ensuring that it remains within the optimal range for efficient operation. Common types of cooling systems include:
- Air-cooled systems: In air-cooled systems, the hydraulic fluid is passed through a heat exchanger or cooling fins, where it is cooled by ambient air. These systems are simple and cost-effective but may not be sufficient for heavy-duty presses that generate a lot of heat.
- Liquid-cooled systems: Liquid cooling systems use water or another coolant to absorb heat from the hydraulic fluid. The coolant is circulated through a heat exchanger, where it transfers the heat away from the fluid. Liquid-cooled systems are more effective at managing high heat loads and are commonly used in large or high-performance presses.
- Reservoir cooling: The hydraulic reservoir itself can act as a cooling mechanism by allowing the hydraulic fluid to cool before being recirculated through the system. Larger reservoirs provide more surface area for heat dissipation, improving cooling efficiency.
Maintaining the proper temperature of the hydraulic fluid is critical to preventing performance degradation and prolonging the life of the press. Overheating can cause the fluid to break down, leading to reduced lubrication, increased wear, and potential system failure.
7.4. Fluid Contamination and Its Effect on Performance
One of the most common causes of hydraulic press failure is fluid contamination. Contaminants such as dirt, metal particles, and water can enter the hydraulic system and degrade the performance of the hydraulic fluid. This can lead to inefficiencies in force transmission, increased wear on components, and eventual system failure.
Causes of Fluid Contamination
Contamination of hydraulic fluid can occur for several reasons:
- External contamination: Dirt, dust, and other debris can enter the hydraulic system through leaks, damaged seals, or during fluid changes. External contamination is especially common in presses that operate in harsh or dirty environments, such as factories or construction sites.
- Internal contamination: Over time, the components of the hydraulic system—such as seals, hoses, and cylinders—can wear down, generating metal particles or other debris. These contaminants then circulate within the fluid, causing further damage to the system.
- Water ingress: Water can enter the hydraulic system through condensation, leaks, or improper fluid handling. Water contamination can lead to corrosion of hydraulic components and reduce the effectiveness of the hydraulic fluid.
Effects of Contamination on Hydraulic Press Performance
Fluid contamination can have several negative effects on the performance of a hydraulic press:
- Reduced efficiency: Contaminants can reduce the efficiency of the hydraulic fluid by obstructing fluid flow, leading to pressure losses and reduced force output.
- Increased wear: Contaminants such as metal particles or dirt can increase the wear on hydraulic components, leading to premature failure of seals, cylinders, and valves.
- Corrosion: Water contamination can cause corrosion of the press’s metal components, leading to leaks, reduced pressure, and potential system failure.
- Cavitation: If air bubbles enter the hydraulic system, they can cause cavitation, where the air pockets collapse under pressure, damaging the components and reducing system efficiency.
Preventing Fluid Contamination
Proper fluid management is essential to preventing contamination and maintaining the performance of the hydraulic press. Some common preventive measures include:
- Using high-quality filters: Installing filters in the hydraulic system helps remove contaminants from the fluid before they can cause damage. Regularly inspecting and replacing filters is crucial to preventing contamination.
- Proper fluid handling: When adding or replacing hydraulic fluid, it is important to ensure that the new fluid is clean and free of contaminants. Using clean containers and handling the fluid in a controlled environment can prevent contamination.
- Seal maintenance: Ensuring that seals and gaskets are in good condition can prevent external contaminants from entering the system. Regular inspection and replacement of seals are essential for maintaining system integrity.
EMS Metalworking Machinery
We design, manufacture and assembly metalworking machinery such as:
- Hydraulic transfer press
- Glass mosaic press
- Hydraulic deep drawing press
- Casting press
- Hydraulic cold forming press
- Hydroforming press
- Composite press
- Silicone rubber moulding press
- Brake pad press
- Melamine press
- SMC & BMC Press
- Labrotaroy press
- Edge cutting trimming machine
- Edge curling machine
- Trimming beading machine
- Trimming joggling machine
- Cookware production line
- Pipe bending machine
- Profile bending machine
- Bandsaw for metal
- Cylindrical welding machine
- Horizontal pres and cookware
- Kitchenware, hotelware
- Bakeware and cuttlery production machinery
as a complete line as well as an individual machine such as:
- Edge cutting trimming beading machines
- Polishing and grinding machines for pot and pans
- Hydraulic drawing presses
- Circle blanking machines
- Riveting machine
- Hole punching machines
- Press feeding machine
You can check our machinery at work at: EMS Metalworking Machinery – YouTube
Applications:
- Beading and ribbing
- Flanging
- Trimming
- Curling
- Lock-seaming
- Ribbing
- Flange-punching