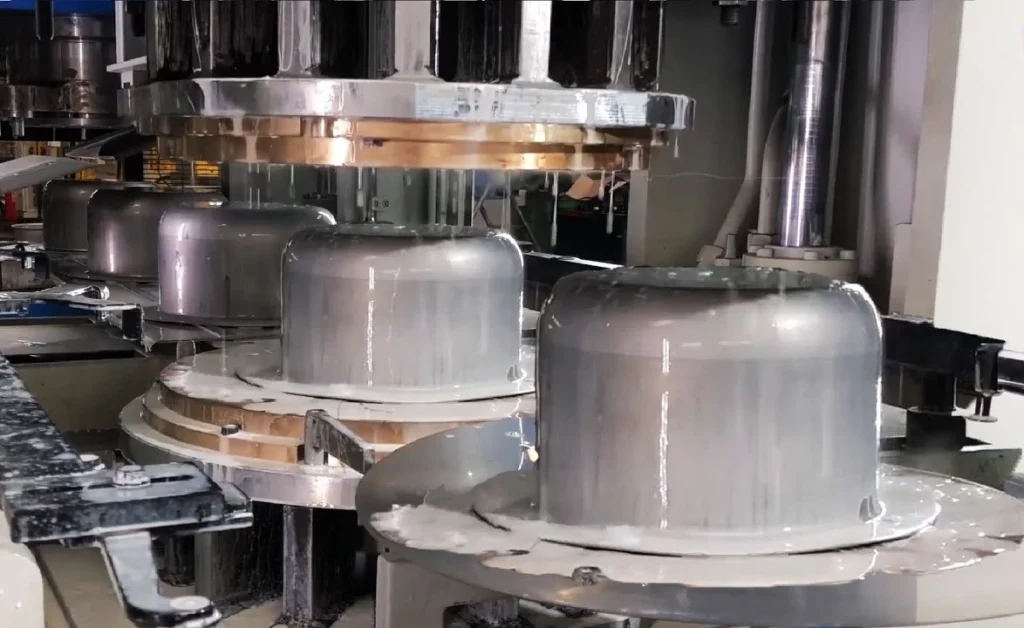
Deep Drawing Calculations and main principles of deep drawing with a hydraulic deep drawing press. Free consultation. Price from the manufacturer.
Deep Drawing Calculations
Deep drawing is a metal forming process used to form sheet metal into a desired shape. The process involves drawing a flat sheet of metal into a die cavity, using a punch. The sheet is then stretched and formed into the shape of the die cavity.
There are several calculations involved in the deep drawing process to ensure the final product meets the desired specifications. Some of the key calculations include:
- Blank size calculation: The size of the blank needed for deep drawing is calculated based on the desired diameter of the finished product, the depth of the draw, and the thickness of the material.
- Drawing force calculation: The force required to draw the material into the die cavity is calculated based on the size and shape of the die, the material properties, and the desired depth of the draw.
- Strain calculation: The strain on the material during the deep drawing process is calculated based on the initial and final dimensions of the material and the geometry of the die.
- Frictional force calculation: The force required to overcome the friction between the material and the die during the deep drawing process is calculated based on the coefficient of friction between the two materials.
- Punch force calculation: The force required to push the punch through the material during the deep drawing process is calculated based on the material properties and the geometry of the punch.
Overall, these calculations help ensure that the deep drawing process is carried out efficiently and effectively, resulting in a final product that meets the desired specifications.
Blank size calculation
Blank size calculation is an important aspect of deep drawing calculations, as it determines the amount of material required for the production of a specific part. The blank size is calculated based on the dimensions of the finished part, the material properties, and the desired thickness of the blank.
The basic formula for calculating the blank size is:
Blank Diameter (BD) = Finished Diameter (FD) + (2 x Depth of Draw (DOD))
where the depth of draw is the difference between the height of the blank before and after forming.
The blank size calculation also takes into account the material’s yield strength and its elongation at break, which are used to calculate the amount of deformation that can be achieved without cracking or tearing the material.
Other factors that can affect the blank size calculation include the type of tooling used, the press capacity, and the lubrication used during the drawing process.
Drawing force calculation:
Calculating the drawing force required for a deep drawing process involves several factors, including the material properties, the blank size and shape, the punch and die dimensions, and the friction between the material and the tooling.
One common equation used to calculate the drawing force is:
F = K * T * L * (S – L * R)
Where:
F = Drawing force (in pounds or newtons) K = Coefficient of drawing force (typically ranges from 0.5 to 0.6) T = Tensile strength of the material (in psi or MPa) L = Blank diameter or length (in inches or millimeters) S = Punch diameter or length (in inches or millimeters) R = Die radius (in inches or millimeters)
It’s important to note that this equation provides an estimate and may need to be adjusted based on specific process conditions and the desired level of accuracy. Additionally, other factors such as the material’s yield strength and work hardening behavior may also need to be considered. It’s recommended to consult with an experienced engineer or use specialized software to accurately calculate the required drawing force for a deep drawing process.
Strain calculation
In deep drawing, strain is the amount of deformation per unit length of the original material. It is an important parameter that determines the quality of the final product. The strain can be calculated using the following formula:
ε = ln (r/R)
Where:
- ε is the strain
- r is the radius of the punch
- R is the radius of the blank
The strain is directly proportional to the depth of the draw, which means that as the depth of the draw increases, the strain also increases. It is important to calculate the strain to ensure that the material does not exceed its elastic limit and cause defects in the final product.
Frictional force calculation
Calculating the frictional force is an essential step in determining the drawing force in deep drawing. The frictional force acts on the blank as it moves through the die and affects the drawing force required to form the blank into the desired shape. The frictional force depends on several factors, including the surface finish of the die, the material properties of the blank and die, and the lubrication conditions.
To calculate the frictional force, the coefficient of friction (µ) is required. The coefficient of friction is a dimensionless quantity that represents the ratio of the frictional force between two surfaces to the normal force acting perpendicular to those surfaces. The coefficient of friction can be determined experimentally or estimated based on empirical formulas.
Once the coefficient of friction is known, the frictional force can be calculated using the following equation:
Frictional force = µ x Normal force
where the normal force is the force acting perpendicular to the surface of the blank. The normal force is equal to the drawing force in deep drawing.
In summary, the frictional force can be calculated by determining the coefficient of friction between the blank and die surfaces and multiplying it by the normal force acting on the blank during the deep drawing process.
Punch force calculation
Punch force calculation in deep drawing involves determining the force required to punch the metal sheet into the desired shape. The punch force is determined based on various factors such as the size of the punch, the thickness of the sheet, the yield strength of the material, and the desired shape of the finished product.
The punch force can be calculated using the following formula:
Punch Force = (Shear Strength of Material x Perimeter of Blank) – (Blank Thickness x Drawing Force)
Here, the shear strength of the material is the maximum shear stress that the material can withstand before it starts to deform plastically. The perimeter of the blank is the length of the boundary of the blank, and the drawing force is the force required to pull the metal sheet through the die.
Once the punch force is calculated, it can be used to select an appropriate press machine for the deep drawing process. The press machine should be capable of providing the required punch force without exceeding its maximum capacity.
Hydraulic presses are powerful machines used to apply a significant amount of force to an object through hydraulic fluid pressure. They are essential in various industrial applications, providing the necessary force for processes such as metal forming, stamping, bending, and molding. The versatility and efficiency of hydraulic presses make them indispensable tools in manufacturing and production lines. This document will explore the different types of hydraulic presses, their application areas, components, operational principles, manufacturing process, and the challenges and advancements in the industry.
Types of Hydraulic Presses
Hydraulic presses come in various designs, each suited to specific applications and requirements. The primary types of hydraulic presses include C-frame presses, H-frame presses, four-column presses, straightening presses, arbor presses, laminating presses, and transfer presses.
C-frame presses, also known as gap-frame presses, have a C-shaped frame that provides three-sided access to the work area. This design is ideal for applications requiring easy loading and unloading of materials.
H-frame presses, or two-post presses, have a robust H-shaped frame that offers excellent stability and strength. They are commonly used for heavy-duty tasks such as metal forming and straightening.
Four-column presses, or four-post presses, have four vertical columns that provide superior support and uniform force distribution. These presses are suitable for large-scale applications requiring high precision and repeatability.
Straightening presses are specialized hydraulic presses used to straighten bent or distorted metal components. They are widely used in the automotive and construction industries.
Arbor presses are smaller, manually operated hydraulic presses used for light-duty tasks such as assembly, riveting, and broaching. They are commonly found in workshops and small manufacturing facilities.
Laminating presses are used to bond multiple layers of material together under heat and pressure. These presses are essential in industries such as electronics, where laminated components are common.
Transfer presses are automated hydraulic presses that move the workpiece through multiple stations for different operations. They are highly efficient and used in high-volume production environments.
Application Areas
Hydraulic presses are employed in various industries, thanks to their ability to deliver consistent and precise force. Key application areas include:
Metal forming and forging: Hydraulic presses are crucial in shaping and forming metal parts through processes such as stamping, bending, and deep drawing. They are essential in the production of automotive parts, machinery components, and structural elements.
Automotive industry: In the automotive sector, hydraulic presses are used for manufacturing various parts, including body panels, chassis components, and engine parts. They play a critical role in ensuring the structural integrity and performance of vehicles.
Aerospace industry: The aerospace industry relies on hydraulic presses for forming and shaping high-strength materials used in aircraft components. Precision and reliability are paramount in this industry, making hydraulic presses indispensable.
Plastic and rubber molding: Hydraulic presses are used in the molding of plastic and rubber components, including automotive parts, household goods, and medical devices. They ensure consistent product quality and precision.
Electrical and electronics industry: In the electronics sector, hydraulic presses are used for laminating circuit boards, forming connectors, and assembling electronic components. They provide the necessary force and precision for delicate operations.
Medical device manufacturing: Hydraulic presses are used in the production of medical devices, including surgical instruments, implants, and diagnostic equipment. They ensure the high precision and quality required in the medical field.
Packaging industry: Hydraulic presses are employed in the packaging industry for forming and shaping packaging materials, such as cardboard, plastic, and metal. They help produce packaging solutions that are strong, durable, and aesthetically pleasing.
Components of a Hydraulic Press
A hydraulic press comprises several key components that work together to generate and control the applied force. The main components include the frame, hydraulic cylinder, hydraulic pump, control valves, hydraulic fluid, pressure gauges and sensors, and die and tooling.
The frame is the main structure of the hydraulic press, providing stability and support for all other components. It is typically made of high-strength steel to withstand the significant forces generated during operation.
The hydraulic cylinder is the core component that generates the pressing force. It consists of a cylindrical chamber, a piston, and a piston rod. When hydraulic fluid is pumped into the cylinder, it moves the piston, which in turn applies force to the workpiece.
The hydraulic pump is responsible for generating the hydraulic fluid pressure needed to move the piston. It draws hydraulic fluid from a reservoir and delivers it to the cylinder under high pressure.
Control valves regulate the flow of hydraulic fluid to and from the cylinder, controlling the movement and force of the press. These valves can be manually operated or automated, depending on the press design.
Hydraulic fluid, typically oil, is the medium through which force is transmitted in the hydraulic system. It must have suitable properties, such as viscosity and lubricity, to ensure efficient operation and protect system components.
Pressure gauges and sensors monitor the hydraulic fluid pressure within the system. They provide real-time feedback to the operator or control system, ensuring safe and accurate press operation.
Die and tooling are the interchangeable components that come into direct contact with the workpiece. They are designed to shape, form, or cut the material as required by the specific application.
How Hydraulic Presses Work
Hydraulic presses operate based on Pascal’s principle, which states that pressure applied to a confined fluid is transmitted equally in all directions. This principle allows hydraulic presses to generate significant force with relatively small input pressure.
The operation of a hydraulic press begins with the hydraulic pump drawing fluid from the reservoir and delivering it to the cylinder. The control valves regulate the flow of fluid, directing it into the cylinder to move the piston. As the piston moves, it applies force to the workpiece placed between the die and tooling.
The hydraulic fluid plays a crucial role in this process, as it transmits the applied pressure and lubricates the system components. The pressure gauges and sensors continuously monitor the fluid pressure, providing feedback to ensure the press operates within safe limits.
The force generated by the hydraulic press can be precisely controlled by adjusting the hydraulic fluid pressure and the position of the control valves. This allows for accurate and repeatable operations, essential for high-quality manufacturing.
Manufacturing of Hydraulic Presses
The manufacturing of hydraulic presses involves several stages, from design and engineering to assembly and quality control. Each stage is critical to ensuring the press’s performance, reliability, and safety.
Design and engineering: The process begins with the design and engineering phase, where specifications for the press are developed based on the intended application. This includes selecting suitable materials, determining the required force and stroke, and designing the frame and hydraulic system.
Material selection: High-quality materials, such as high-strength steel for the frame and durable alloys for the hydraulic components, are selected to ensure the press’s longevity and performance.
Fabrication of components: The individual components of the hydraulic press, including the frame, cylinder, and pump, are fabricated using precision machining and manufacturing techniques. This ensures that each component meets the required tolerances and specifications.
Assembly process: The fabricated components are then assembled into the complete hydraulic press. This involves mounting the cylinder, pump, and control valves onto the frame, connecting the hydraulic lines, and installing the die and tooling.
Quality control and testing: Rigorous quality control measures are implemented throughout the manufacturing process to ensure the press meets all specifications and standards. This includes pressure testing the hydraulic system, verifying the accuracy of the control valves, and performing operational tests to ensure the press functions correctly.
Advancements and Innovations
The hydraulic press industry is continually evolving, driven by advancements in technology and increasing demands for efficiency and precision. Key innovations include automation and control systems, energy efficiency improvements, and smart hydraulic presses.
Automation and control systems: Modern hydraulic presses are often equipped with advanced control systems that automate the pressing process. This includes programmable logic controllers (PLCs), human-machine interfaces (HMIs), and sensors that monitor and adjust the press’s operation in real time. Automation improves efficiency, reduces the risk of human error, and enhances the consistency of the finished products.
Energy efficiency improvements: Manufacturers are focusing on developing hydraulic presses that consume less energy and have a smaller environmental footprint. This includes using variable displacement pumps, energy recovery systems, and optimizing the hydraulic system’s design to minimize energy losses.
Smart hydraulic presses: The integration of IoT (Internet of Things) technology into hydraulic presses has led to the development of smart presses. These presses can communicate with other machines and systems, providing real-time data on their status, performance, and maintenance needs. This connectivity allows for predictive maintenance, reducing downtime and extending the press’s lifespan.
Challenges in Hydraulic Press Manufacturing
The manufacturing of hydraulic presses presents several challenges, including precision and quality requirements, cost management, technological advancements, and environmental considerations.
Precision and quality requirements: Hydraulic presses must deliver consistent and precise force, which requires high levels of accuracy in the manufacturing process. Ensuring each component meets the required tolerances and specifications is critical to the press’s performance and reliability.
Cost management: The cost of materials, labor, and energy can significantly impact the overall cost of manufacturing hydraulic presses. Manufacturers must balance quality and cost to remain competitive in the market.
Technological advancements: Keeping up with technological advancements is essential for manufacturers to meet the evolving demands of the industry. This requires continuous investment in research and development to incorporate new technologies and improve existing designs.
Environmental considerations: Environmental regulations and sustainability concerns are increasingly important in hydraulic press manufacturing. Manufacturers must develop eco-friendly presses that consume less energy, use recyclable materials, and minimize their environmental impact.
Conclusion
Hydraulic presses are essential machines in various industries, providing the necessary force for processes such as metal forming, stamping, and molding. Understanding the different types of hydraulic presses, their components, and how they work is crucial for effective application and operation.
The manufacturing process of hydraulic presses involves careful design and engineering, material selection, precision fabrication, and rigorous quality control. Despite the challenges, advancements in technology and innovations are driving the industry forward, leading to more efficient, precise, and environmentally friendly hydraulic presses.
As industries continue to evolve, the hydraulic press industry must adapt and innovate to meet the demands of efficiency, precision, and sustainability. Through continuous research and development, manufacturers can enhance the performance and reliability of hydraulic presses, contributing to the success of various industrial applications.