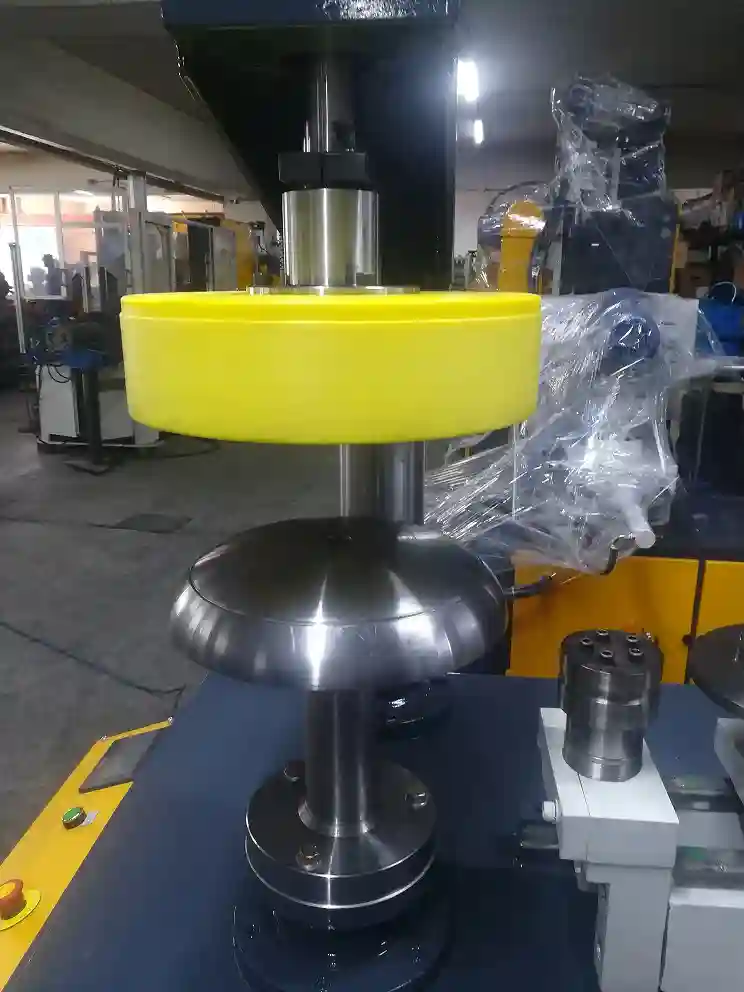
Sheet Metal Trimming Machine: A sheet metal forming machine is a type of manufacturing equipment used to shape or bend sheet metal into various forms or components. These machines are essential in industries like automotive, aerospace, construction, and home appliances. There are several types of sheet metal forming machines, each suited for different operations depending on the shape, thickness, and material of the sheet metal.
Common Types of Sheet Metal Forming Machines
- Press Brake (or Brake Press)
- Purpose: Bending sheet metal at various angles.
- Types: Mechanical, hydraulic, pneumatic, and servo-electric.
- Applications: Making boxes, enclosures, brackets, and other bent shapes.
- Power Press
- Purpose: Punching, blanking, deep drawing, or forming operations.
- Types: Mechanical or hydraulic.
- Applications: Mass production of components like washers, deep-drawn cups, etc.
- Roll Forming Machine
- Purpose: Continuous bending of long strips of sheet metal.
- Process: Metal passes through a series of rollers that progressively shape it.
- Applications: Roofing sheets, metal siding, gutters, etc.
- Hydraulic Press
- Purpose: Deep drawing, punching, and forming.
- Advantages: Can handle thicker metals and provide higher force.
- Spinning Machine
- Purpose: Rotational forming to create round parts.
- Applications: Producing symmetrical objects like gas cylinders, cones, and hemispheres.
- Stretch Forming Machine
- Purpose: Stretching and bending sheet metal simultaneously.
- Applications: Aircraft skins, curved panels, and complex contours.
- Stamping Press
- Purpose: High-speed punching, blanking, embossing, and coining.
- Used With: Dies and tools specifically shaped for the desired output.
- Shearing Machine
- Purpose: Cutting sheet metal to size before forming.
- Types: Guillotine shear, bench shear, power shear.
Key Considerations When Choosing a Machine
- Material type and thickness
- Size and complexity of the part
- Production volume
- Required accuracy and repeatability
- Budget and space
A sheet metal forming machine is a manufacturing tool used to shape flat sheets of metal into specific forms or components without removing any material. These machines are essential in industries like automotive, aerospace, construction, and consumer electronics, where precise metal parts are required in high volume and consistent quality. The forming process relies on applying force to deform the sheet metal plastically, changing its shape without breaking or cutting it.
One of the most common types is the press brake, which bends sheet metal into desired angles using a punch and die setup. It is widely used for forming parts like brackets, panels, and chassis. Another widely used machine is the power press, capable of performing tasks like blanking, punching, and deep drawing. It’s particularly effective in high-speed production environments due to its ability to shape metal in a single stroke.
Roll forming machines are designed for continuous bending of long strips of metal by passing them through a series of rollers. These machines are ideal for producing uniform profiles such as roofing sheets, door frames, and channels. Hydraulic presses, with their ability to exert very high pressure, are used for deep drawing and forming operations on thicker sheets. They offer more control and are suitable for complex shapes.
Spinning machines are used when a symmetrical, round component is needed. A flat sheet or disc is rotated and pressed over a mold using a roller tool to form items like gas cylinders, cones, and domes. For large panels or parts with smooth contours, stretch forming machines apply tension and bend the sheet over a form, commonly used in aircraft manufacturing.
Stamping presses operate at high speeds and are suitable for tasks such as embossing, coining, and forming with high precision. They are typically used with custom-designed dies and punches for mass production. Before forming begins, shearing machines are often used to cut sheet metal to the required size. These can be manual or powered and are designed to provide clean, straight cuts.
Selecting the right sheet metal forming machine depends on factors such as material type and thickness, complexity of the part, production volume, and the level of precision required. Each machine type has its own advantages, and in many manufacturing setups, multiple machines are used together to complete the entire forming process efficiently.
In addition to machine types, the success of sheet metal forming also depends heavily on tooling and material behavior. The punch and die configuration in a press brake or stamping press must be precisely matched to the metal’s properties and desired shape. Factors like springback, which is the metal’s tendency to return to its original shape after bending, must be compensated for during the tool design phase. Advanced software tools are now often used for simulating forming operations to predict issues like tearing, wrinkling, or insufficient forming.
Materials used in sheet metal forming range from aluminum and stainless steel to copper and titanium, depending on the application. Each metal has different ductility and tensile strength, which influences how easily it can be formed and the amount of force required. For instance, aluminum is lightweight and relatively easy to form, making it a preferred choice in the automotive and aerospace sectors. On the other hand, high-strength steel is harder to form but offers superior durability and is often used in structural applications.
Automation is also playing an increasingly important role in modern sheet metal forming. CNC (Computer Numerical Control) systems allow for precise, repeatable movements of machine components, reducing manual labor and increasing accuracy. Robotic arms can feed sheets into machines, transfer parts between stations, or perform post-forming tasks like welding or assembly. This not only boosts productivity but also minimizes errors and improves safety in production environments.
Safety is a major consideration in sheet metal forming operations, as the machines exert significant force and involve sharp edges and high-speed components. Safety features like light curtains, emergency stops, two-hand controls, and enclosed work areas are standard on modern machines. Operator training is equally important to ensure proper handling, maintenance, and emergency response.
In recent years, the industry has also seen growth in flexible forming technologies like hydroforming and incremental sheet forming. Hydroforming uses a high-pressure fluid to shape sheet metal against a die, allowing for complex and seamless shapes with high strength-to-weight ratios. Incremental forming, which uses a small tool to gradually shape a sheet through localized deformation, is suitable for prototyping and small-batch production due to its flexibility and low tooling cost.
Overall, sheet metal forming machines are a cornerstone of industrial manufacturing. With advancements in material science, digital control, and forming techniques, they continue to evolve—enabling faster production, better precision, and the ability to form increasingly complex geometries. Whether for mass production or custom components, these machines are essential for transforming raw sheet metal into the parts and products we rely on every day.
As industries demand more complex, lightweight, and durable components, the evolution of sheet metal forming machines is becoming increasingly centered around precision engineering, digital integration, and sustainability.
One of the most impactful advancements is the integration of smart manufacturing technologies, such as IoT (Internet of Things) sensors and real-time data analytics. Modern machines can monitor forming force, stroke cycles, temperature, and even vibration during operation. This data is fed into monitoring systems that can detect anomalies early, predict tool wear, and optimize maintenance schedules—minimizing downtime and extending machine life.
Laser-assisted forming is another emerging technique where localized heating with lasers reduces the force needed to form high-strength materials. This is especially useful in automotive applications, where ultra-high-strength steels are used for crash-resistant vehicle structures. By softening the metal in a controlled way, manufacturers can form more intricate shapes without cracking the material.
Meanwhile, additive manufacturing (3D printing) is starting to complement traditional sheet metal processes, especially for the creation of forming dies and custom tooling. Complex, lightweight die structures can be produced more quickly and with optimized internal features, such as cooling channels, which improve performance and reduce lead times.
In terms of energy efficiency, newer hydraulic and electric press systems are designed to consume less power and operate more quietly compared to traditional machines. Servo-electric presses, for example, use precise electric actuators instead of hydraulics, enabling extremely accurate control over speed and position while reducing oil usage and environmental impact.
Another area gaining traction is modular machine design, where sheet metal forming machines can be quickly adapted to different tasks or materials by changing modules or tooling. This flexibility is crucial for industries like aerospace or medical devices, where frequent design changes and small batch sizes are common.
From a sustainability perspective, the industry is also focused on minimizing material waste. This is done through better nesting algorithms (for cutting operations), scrap recycling systems, and more efficient forming techniques that reduce the number of rejected parts. Closed-loop feedback systems between CAD/CAM software and the forming machine can adjust tool paths or bending angles in real-time to ensure the part remains within tolerance throughout production.
In educational and prototyping environments, desktop-scale sheet metal forming machines are becoming more accessible. These machines allow students, designers, and small manufacturers to experiment with forming techniques at a smaller scale, encouraging innovation and hands-on learning without large capital investment.
Looking ahead, the future of sheet metal forming will likely include greater use of AI-driven optimization, where machine learning algorithms analyze vast amounts of production data to improve process parameters continuously. Combined with robotics, machine vision, and collaborative interfaces, these intelligent systems will enable autonomous forming lines capable of adjusting to new product designs with minimal human intervention.
The sheet metal forming machine, once a purely mechanical workhorse, has now become a high-tech, adaptive part of the modern manufacturing ecosystem—bridging traditional metalworking skills with the digital demands of Industry 4.0.
Sheet Metal Drawing Machine
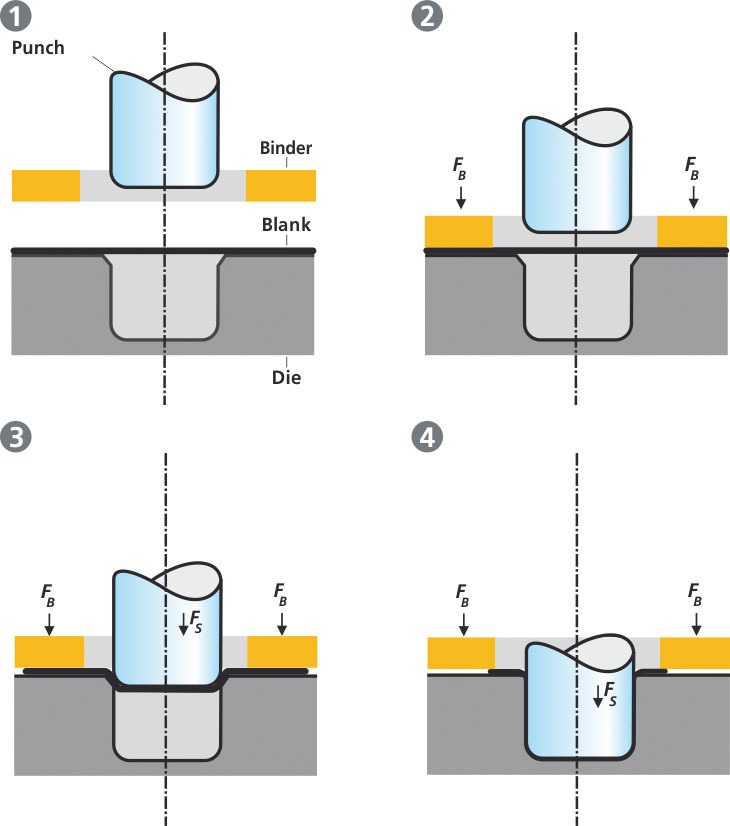
A Sheet Metal Drawing Machine is a specific type of forming machine used in the deep drawing process, where a flat sheet metal blank is radially drawn into a forming die by the mechanical action of a punch. It transforms a flat sheet into a hollow or cup-shaped component without wrinkling, tearing, or excessive thinning. This process is widely used to manufacture parts like kitchen sinks, gas cylinders, automotive body panels, and various containers.
How It Works
In a sheet metal drawing machine, the metal blank is placed over a die cavity, and a blank holder (also called a pressure pad) holds it in place to control material flow. A punch then pushes the metal into the die, stretching it into a new shape. The punch and die are usually precision-engineered to maintain tight tolerances and avoid surface defects.
Types of Sheet Metal Drawing Machines
- Hydraulic Drawing Press
- Offers precise force control, ideal for deep drawing high-strength or thick materials.
- Often used for complex or large parts.
- Smooth and adjustable speed and stroke.
- Mechanical Drawing Press
- Operates via crankshaft and flywheel mechanisms.
- Faster than hydraulic types, but less adaptable to complex geometries.
- Suitable for high-speed production of shallow drawn parts.
- Double Action Drawing Press
- Has two rams: one controls the blank holder, and the other moves the punch.
- Ensures better control over material flow during deep drawing.
- Common in automotive and appliance manufacturing.
- Pneumatic Drawing Press
- Uses compressed air to drive the punch.
- Simple and clean but limited in force capacity.
- More often used for light gauge metal and smaller parts.
Key Components
- Die: The cavity into which the sheet is drawn.
- Punch: The tool that presses the sheet into the die.
- Blank Holder: Holds the sheet and prevents wrinkling.
- Draw Beads: Control the metal flow into the die cavity.
Applications
- Automotive: Fuel tanks, body panels, wheel housings.
- Consumer goods: Kitchen sinks, cooking pots, metal containers.
- Aerospace: Engine housings, pressure vessels.
- Industrial: Electrical enclosures, filter housings.
Materials Typically Used
- Mild steel
- Stainless steel
- Aluminum
- Copper and brass
- Titanium (for aerospace and medical)
Advantages
- High dimensional accuracy
- Smooth surface finish
- Stronger parts due to work hardening
- Efficient material usage for hollow parts
Challenges
- Requires precise control to avoid tearing or wrinkling
- Tooling is expensive and needs regular maintenance
- Material selection is critical for successful drawing
A sheet metal drawing machine is designed to perform one of the most critical processes in metal forming—deep drawing. This operation transforms flat metal blanks into hollow, cup-like or box-like shapes using a combination of pressure, controlled force, and precision tooling. Unlike bending or rolling, drawing involves significant plastic deformation, where the material is pulled radially inward and forced into a die cavity by a punch. The machine must ensure that the material flows correctly without wrinkling at the flange or tearing near the punch radius, both of which are common defects if the process isn’t carefully controlled.
The machine typically operates in a sequence starting with the placement of a flat metal blank over a die opening. A blank holder then clamps down on the edges of the blank to control how the metal flows during the punch stroke. As the punch descends, it pushes the material into the die, forming it into the desired shape. The challenge here lies in maintaining a balance between holding force and punch force. If the blank holder force is too high, it may restrict the material flow and cause tearing; if too low, it may lead to wrinkling or uncontrolled flow. This delicate balance is why deep drawing machines often feature sophisticated hydraulic systems or servo-controlled actuators to finely tune these forces in real time.
The performance of a drawing machine also depends on the geometry of the punch and die, the lubrication conditions, and the properties of the sheet material. Smooth die surfaces and proper lubrication are critical because they reduce friction and prevent galling, which can damage both the part and the tooling. Additionally, the material’s ductility, thickness, and grain direction play a big role in determining how deep or complex a shape can be drawn in a single stroke. For more difficult parts or stronger metals, multi-step drawing processes may be used, involving redrawing, ironing, or annealing between steps to relieve stresses and allow further shaping.
In mass production environments, especially in automotive and appliance manufacturing, drawing machines are often automated and integrated into press lines with robotic handling systems. This automation not only improves speed and consistency but also reduces the physical strain on operators and minimizes human error. These systems can produce thousands of identical parts per shift with very tight tolerances and surface finish requirements. Sensors and real-time monitoring systems track each stroke, ensuring every part meets quality standards, and stopping the process if any anomaly is detected.
Modern sheet metal drawing machines are also increasingly equipped with digital controls and feedback systems that allow for adaptive forming. With the integration of Industry 4.0 technologies, these machines can learn from each production cycle, adjusting parameters like punch speed, dwell time, or holding pressure dynamically to optimize part quality. This is especially useful when forming high-performance alloys or working with recycled materials, where variability in sheet quality can affect outcomes.
In research and prototyping applications, smaller-scale or lab-grade drawing machines are used to test material formability, study lubrication effects, and develop new forming strategies. These experimental setups help manufacturers design better tooling and predict formability limits using techniques like finite element analysis, which can simulate the drawing process before any physical part is made.
As manufacturers continue to push for lighter, stronger, and more complex components—particularly in electric vehicles, aerospace, and medical devices—sheet metal drawing machines will remain a foundational technology. Their evolution will likely focus on even greater precision, faster cycle times, improved material compatibility, and deeper integration with smart manufacturing ecosystems.
In advanced manufacturing settings, the role of sheet metal drawing machines goes beyond simply forming shapes—it’s about achieving consistently high quality at high speed while adapting to ever-changing design requirements and materials. As product development cycles become shorter, drawing machines are expected to handle a wider range of geometries and materials without the need for extensive retooling. This flexibility is being enabled by modular tooling systems and programmable control interfaces that allow rapid setup changes and fine-tuning of forming parameters.
Tooling in a drawing machine, particularly the die and punch, must be designed with not only the final shape in mind but also the mechanical properties of the material during deformation. For example, sharper corners on a die might result in stress concentration and tearing if the material doesn’t have sufficient ductility. To prevent this, the edges are usually radiused, and in critical applications, simulations are performed before tooling is ever manufactured. Finite Element Method (FEM) simulations are now commonly used to predict material flow, thinning, stress distribution, and potential failure zones. These simulations save both time and money by identifying and correcting issues early in the design stage.
Another important aspect is lubrication. The drawing process involves significant contact between the tool and the sheet metal, and without proper lubrication, there’s a high risk of surface defects and tool wear. Lubricants must be carefully chosen based on the material type, the complexity of the draw, and environmental considerations. For example, deep drawing aluminum requires different lubricants than stainless steel due to differences in surface adhesion and friction characteristics. In high-speed automated lines, lubrication systems are integrated directly into the machine to apply the right amount at the right locations on each blank.
The evolution of materials also presents new challenges and opportunities for sheet metal drawing. With the rise of lightweight alloys like aluminum-magnesium blends, ultra-high-strength steels (UHSS), and even titanium in aerospace and medical fields, drawing machines must be capable of applying higher forces with greater control. These materials often require a combination of deep drawing and additional processes like ironing, where the wall thickness is reduced and the surface is smoothed, or reverse drawing, where the part is drawn in the opposite direction to refine geometry and strength.
In some advanced processes, sheet metal drawing is combined with hydroforming, where a pressurized fluid supports the material during forming, allowing for more complex and delicate shapes without cracking. Similarly, warm forming involves heating the sheet metal before drawing to improve ductility, especially for high-strength alloys. Drawing machines that incorporate such hybrid techniques expand the range of parts that can be manufactured, offering designers more freedom and enabling the creation of optimized lightweight structures.
The growing emphasis on sustainability in manufacturing also affects drawing operations. Manufacturers are looking for ways to reduce scrap, reuse lubricants, and lower energy consumption. Energy-efficient motors, regenerative braking in hydraulic systems, and advanced monitoring help meet environmental standards while maintaining productivity. Some manufacturers are also exploring forming recycled sheet metal, which introduces variability in the input material and requires highly responsive drawing machines to compensate in real time.
As these machines become more digitally connected, operators and engineers can access real-time dashboards showing cycle times, press loads, lubricant flow rates, part dimensions, and even predictive maintenance alerts. The machine effectively becomes part of a smart factory ecosystem where every stroke contributes data to improve the process continuously. AI and machine learning algorithms analyze this data to recommend settings, adjust machine behavior on the fly, or suggest when to replace tooling before defects occur.
Ultimately, the modern sheet metal drawing machine is not just a tool for shaping metal—it’s a high-precision, intelligent platform that supports innovation in design and efficiency in production. It sits at the intersection of mechanical engineering, material science, data analytics, and automation, enabling manufacturers to create stronger, lighter, and more complex components with incredible reliability.
Sheet Metal Bulging Machine
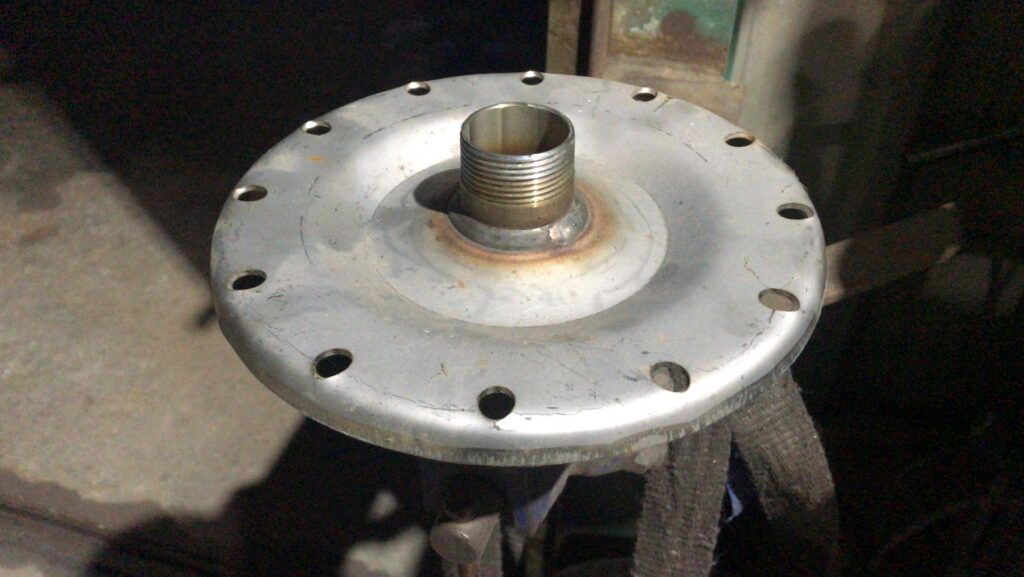
A Sheet Metal Bulging Machine is a specialized forming machine used to expand or “bulge” a portion of a hollow or tubular metal workpiece outward, creating a controlled protrusion or dome-like feature. This process is commonly applied in the manufacturing of components like pressure vessels, fire extinguishers, bellows, plumbing fittings, automotive exhaust parts, and containers requiring reinforced or functional geometric changes.
Unlike deep drawing, which pulls flat sheet metal into a cavity, bulging pushes part of a closed or partially closed metal surface outward—either by applying internal pressure (hydraulic, pneumatic, or explosive) or by mechanical pressing with shaped dies. The result is a localized increase in diameter or outward expansion of the material in a defined area without tearing or thinning beyond acceptable limits.
The typical working principle involves placing a tubular or closed-end cylindrical workpiece between two die halves. Then, internal pressure is applied, usually with a hydraulic medium or a rubber bladder, which forces the metal to expand outward into the die cavity. Some bulging machines use a punch-and-die mechanism where external force is applied radially or axially to bulge a specific section. The dies define the final external shape of the bulged region, and the process must be carefully controlled to avoid splitting, wrinkling, or material thinning.
Modern bulging machines come in several variations:
- Hydraulic Bulging Machines: Use fluid pressure to expand the metal. Precise and commonly used for medium to large-sized components.
- Rubber Bulging Machines: Employ a rubber pad or diaphragm to apply uniform pressure, often used for complex or delicate shapes.
- Mechanical Bulging Machines: Utilize cam-driven or servo-controlled punches to mechanically expand the material into a bulged shape.
- Explosive Bulging (used in R&D or special aerospace cases): Relies on a controlled detonation to create extreme internal pressure and form complex shapes—rare and highly specialized.
Bulging is especially effective for creating necks, flanges, ribs, and bosses on metal parts without welding or joining separate components. It improves structural rigidity and often reduces part count and weight in assembled products. The success of the bulging process depends on the material’s ductility, wall thickness, initial diameter, and pressure control. Thicker and more ductile materials like stainless steel, aluminum, copper, and mild steel perform well under bulging operations.
Tooling must be robust and designed to match the expected deformation profile. In automated systems, bulging machines can be integrated into production lines with feeding mechanisms, positioning sensors, and post-forming quality checks using vision systems or ultrasonic thickness gauges.
As with other metal forming technologies, bulging machines are increasingly equipped with digital control systems, load monitoring, and CNC/PLC programming to improve repeatability and minimize defect rates. By precisely controlling the expansion process, manufacturers can produce high-integrity parts with smooth surfaces and consistent mechanical properties.
A sheet metal bulging machine operates on the principle of internal or external force application to create a controlled expansion in a localized area of a metal workpiece, typically cylindrical or tubular in shape. The main objective is to form features such as bulges, necks, or domes without cutting or welding, preserving the material’s continuity and structural strength. This makes the bulging process ideal for industries that require pressure-tight, seamless components such as in fire extinguishers, air tanks, automotive exhaust parts, and HVAC systems.
The bulging process begins by securely positioning the metal tube or preformed part within the machine’s die cavity. The die cavity is shaped to define the final form of the bulged area. In most hydraulic or hydro-mechanical bulging machines, a fluid medium—either hydraulic oil or water—is introduced into the interior of the workpiece under very high pressure. As the internal pressure increases, the material is forced outward into the contours of the die, forming a precise and uniform bulge. Rubber-based bulging machines replace the fluid with an elastic membrane or pad that distributes pressure more evenly, which is particularly beneficial when dealing with irregular shapes or complex profiles.
Control of the bulging pressure is critical. Too much force can cause the material to thin excessively or rupture, while too little may result in incomplete forming or wrinkling. Advanced machines use real-time pressure feedback systems and programmable logic controllers (PLCs) to ensure that the pressure curve matches the specific requirements of the material and shape. The material’s yield strength, ductility, and wall thickness directly influence the maximum safe pressure that can be applied. To increase formability, sometimes the material is heated before or during the process, allowing more complex or deeper bulges to be achieved without failure.
In mechanical bulging, instead of internal pressure, force is applied externally through tools or punches that press against a segment of the metal from the outside, often aided by a mandrel inside the tube for support. This is particularly useful for forming beads or reinforcing rings in exhaust pipes or structural frames. These machines are often cam- or servo-driven and provide a fast, repeatable method of producing bulged shapes where high-volume, lower-complexity production is required.
One key advantage of bulging is that it enhances part functionality and aesthetics without the need for joints or weld seams, which can be weak points in pressure vessels or fatigue-sensitive parts. The process also lends itself well to material saving, since the bulged form is achieved through plastic deformation rather than assembly from multiple parts. Additionally, the elimination of joining processes reduces the chances of leaks, corrosion, and manufacturing errors.
Post-forming operations such as trimming, piercing, or calibration are often integrated with the bulging machine or handled in downstream processes. In automated production environments, robots or material handling systems may move the part through several stations—bulging, trimming, cleaning, and inspection—all within a single integrated cell. Non-destructive testing methods such as ultrasonic thickness gauging or eddy current inspection are used to verify that the bulged regions meet safety and dimensional standards, especially in applications involving pressure containment.
Modern bulging machines continue to evolve with the integration of CNC controls, servo-hydraulics, and simulation-driven design. Computer modeling of the bulging process using finite element analysis allows engineers to predict material behavior, identify potential weak points, and optimize die shapes before physical trials begin. This significantly reduces development time and tooling costs, especially when dealing with new materials or complex geometries.
As lightweighting becomes increasingly important in automotive, aerospace, and industrial design, bulging offers a viable method to reduce part weight while maintaining strength and stiffness. It enables designers to create innovative structural features that are both functional and aesthetically clean, and it aligns well with modern goals of material efficiency, automation, and product integrity.
The continued development of sheet metal bulging machines is deeply tied to advances in materials science, automation, and digital manufacturing. As new materials like high-strength steels, aluminum alloys, and even titanium become more common in industrial applications, bulging machines must adapt to handle their unique behaviors under pressure. High-strength steels, for instance, resist deformation and require carefully calculated pressure profiles to bulge effectively without cracking. Aluminum, while more ductile, is prone to thinning and wrinkling, making pressure modulation and die surface finish critically important.
To address these challenges, modern machines often include real-time thickness monitoring and adaptive pressure systems that respond to feedback from sensors during the forming process. These sensors can detect minute changes in material flow or pressure resistance and instantly adjust internal parameters—slowing down the stroke, increasing support pressure, or holding at a particular stage of deformation. This dynamic feedback loop enhances process reliability and significantly reduces scrap rates, which is crucial in industries like aerospace and medical devices where part precision is non-negotiable.
Digital twins are increasingly being used in the development and refinement of bulging processes. A digital twin is a virtual replica of the bulging machine and the forming process, constantly updated with data from sensors, control systems, and simulations. Engineers can use this digital model to simulate countless scenarios—trying different die shapes, material types, or pressure cycles—to optimize production before physically forming even a single part. This integration between physical and virtual systems marks a shift toward smarter, more predictive manufacturing.
Bulging machines are also evolving in terms of structural design and energy efficiency. Servo-hydraulic systems, which replace traditional hydraulic valves with servo-driven actuators, allow for much finer control over pressure and movement while using less energy and generating less heat. These systems also run quieter, require less maintenance, and offer more environmentally friendly operations, aligning with green manufacturing trends. In some cases, fully electric bulging machines are being developed for smaller applications, eliminating the need for hydraulic oil altogether.
Safety is another area where improvements are ongoing. Because bulging involves high internal pressures—sometimes reaching thousands of psi—it presents risks that must be carefully managed. Modern machines feature multi-layer containment systems, emergency release mechanisms, and interlock systems to prevent operation if the part or tooling is misaligned. Operators are protected by transparent shields, pressure-rated enclosures, and software safeguards that halt the process if any parameter moves out of the safe operating range.
From a production standpoint, bulging machines are increasingly modular, allowing quick changeovers between different product types. This is especially useful for manufacturers that produce multiple variants of a part with only slight differences in bulge size or position. Tooling systems can be swapped out quickly, and pre-programmed forming recipes can be loaded through the control interface to switch jobs in minutes rather than hours. This flexibility reduces downtime and supports lean manufacturing practices.
In educational and prototyping environments, scaled-down versions of bulging machines are used to teach metal forming fundamentals and explore new design concepts. These smaller machines allow students and engineers to experiment with different forming strategies, materials, and die geometries in a hands-on way. In research labs, they’re used to explore cutting-edge ideas like multi-step bulging, controlled wrinkling for textured surfaces, or integration with additive manufacturing for hybrid parts.
Ultimately, the sheet metal bulging machine represents a key capability in advanced manufacturing—one that enables designers and engineers to push the boundaries of what can be made from a single piece of metal. Whether it’s a simple flare on a pipe end or a complex, load-bearing dome on a pressure vessel, bulging adds both form and function without compromise. As the technology continues to evolve, its role will only grow in importance across industries that demand strength, reliability, and innovation from every part they produce.
Sheet Metal Deep Draw Stamping Machine
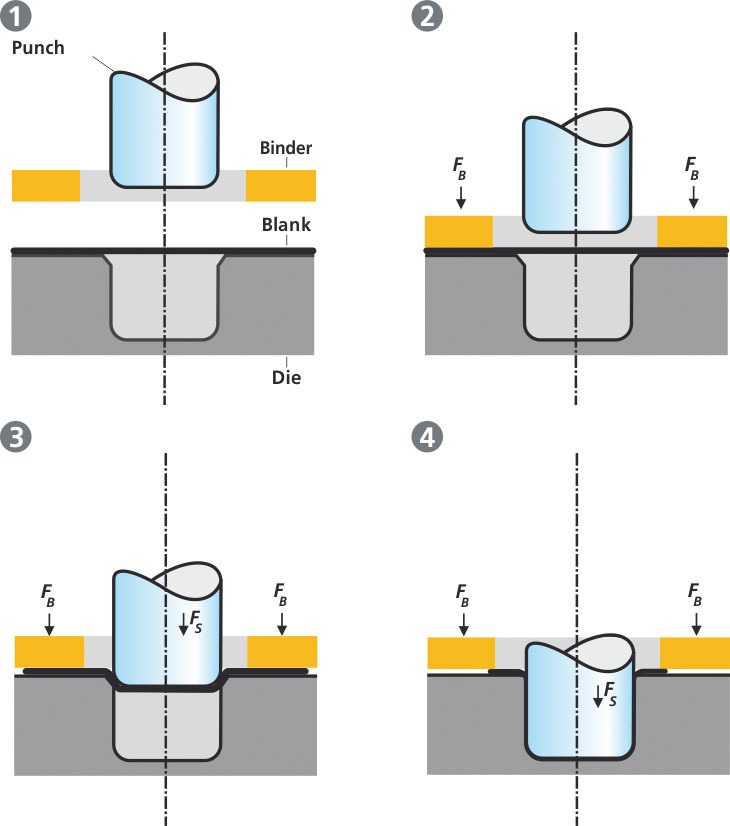
A Sheet Metal Deep Draw Stamping Machine is a specialized piece of equipment used in the process of deep drawing, which is a forming technique that transforms a flat metal sheet into a three-dimensional part. The process typically involves stretching the material into a die cavity using a punch to form shapes such as cups, cylinders, and other complex geometries. Deep drawing is essential in industries like automotive, aerospace, appliances, and consumer electronics, where parts require precise, complex shapes with minimal material waste.
In a deep draw stamping machine, the sheet metal is fed into a die cavity. The die cavity determines the final shape of the part, while a punch presses down on the metal, pushing it into the die. The challenge with deep drawing, compared to other metal forming processes like bending, is that the material must stretch without tearing or excessively thinning, and this requires carefully controlled pressure, speed, and material handling. Deep draw stamping machines are designed to apply the necessary force while controlling the material flow, ensuring that the final part meets dimensional accuracy and surface finish requirements.
The core components of a deep draw stamping machine include the punch, die, blank holder, and sometimes, draw beads. The punch applies downward force on the sheet metal, while the die creates the cavity into which the metal is drawn. The blank holder holds the sheet in place to prevent wrinkling as the punch descends. Draw beads are used to control the material flow into the die and prevent wrinkles in the material during the forming process.
One of the key considerations when designing a deep draw stamping machine is ensuring that the material is evenly distributed and flows properly. If the material is drawn too quickly, it could tear; if it’s drawn too slowly, it may wrinkle. To prevent this, machines are equipped with features like variable stroke control and adjustable blank holding pressure. Additionally, the machine may incorporate lubrication systems to reduce friction between the punch, die, and sheet metal, which helps to prevent wear and tear on the tooling and ensures a smoother finish on the part.
The deep draw stamping process is typically done in stages. For more complex parts, multiple drawing stages might be required. The part is drawn incrementally, with each stage reducing the thickness of the material while increasing its depth. After the final drawing stage, the part may be subjected to further operations like trimming, punching, or flanging to complete its final shape.
These machines can be mechanical presses, which operate via a crank mechanism, or hydraulic presses, which use hydraulic fluid to generate the necessary force. Hydraulic presses are often favored for deep drawing due to their ability to provide precise control over the force, which is essential for creating deep, complex shapes. Mechanical presses, on the other hand, are faster and are typically used for high-volume production of simpler parts.
In modern deep draw stamping machines, automation plays a significant role. Automatic feeders and part handling systems can load sheets, remove finished parts, and handle materials with minimal human intervention, which increases throughput and reduces labor costs. For highly automated operations, robots or conveyors are used to transport parts to the next stage of production, ensuring continuous and efficient processing.
Furthermore, advanced sensors and monitoring systems are now integrated into many machines to ensure process consistency and quality. These systems monitor parameters like pressure, temperature, and material thickness throughout the stamping process. By collecting real-time data, manufacturers can immediately detect deviations in part quality, allowing them to make adjustments to the machine settings on the fly, minimizing the risk of defects and reducing scrap.
Deep draw stamping is used for a wide variety of applications. In automotive manufacturing, it’s used to create parts like body panels, fuel tanks, and wheel rims. In the appliance industry, deep draw stamping is used to create items such as washing machine tubs and sinks. Medical device manufacturers also rely on deep drawing to create components like surgical trays and housings for electronic equipment.
The process itself is highly energy-efficient compared to some other forming techniques, especially when combined with modern hydraulic and automation technologies. It’s also highly scalable, meaning manufacturers can produce large quantities of parts quickly and with minimal waste, which is ideal for industries requiring high-volume production.
To summarize, sheet metal deep draw stamping machines represent a critical technology for producing complex, high-quality metal parts with a smooth, precise finish. By carefully controlling material flow, pressure, and tooling, these machines can create intricate shapes from a single sheet of metal, reducing the need for additional fabrication steps like welding or joining. As demand for lightweight, durable, and complex parts continues to grow in industries ranging from automotive to electronics, deep draw stamping remains a key manufacturing process.
Sheet metal deep draw stamping machines are essential in shaping a wide range of products with complex geometries. These machines excel in creating deep, seamless parts by drawing a flat metal sheet into a cavity using a punch. Unlike other stamping techniques, deep drawing significantly stretches the material, transforming it into hollow or cup-like shapes. This process is crucial in industries like automotive, aerospace, and consumer electronics, where precision, part strength, and material efficiency are critical.
The challenge of deep drawing lies in managing material flow and preventing issues like tearing, thinning, or wrinkling during the forming process. Proper management of force, speed, and material positioning is essential to achieve high-quality parts. This is why deep draw stamping machines often have features that allow for adjustable blank holding pressure, variable stroke control, and precise lubrication systems. These components work in tandem to control the material’s behavior and ensure the part’s integrity, reducing scrap and defects.
Deep drawing machines may be equipped with different types of presses depending on the application. Hydraulic presses are commonly used for deep drawing because they offer precise control over the applied force, which is essential for creating parts with greater depth and complexity. Mechanical presses, on the other hand, are often used for higher-volume production of simpler shapes due to their speed and efficiency. The choice between hydraulic and mechanical presses depends on the complexity of the parts being produced and the required production speed.
To enhance efficiency and reduce downtime, modern deep draw stamping machines are increasingly automated. Automated systems for feeding material, handling finished parts, and positioning components improve throughput and minimize the need for manual labor. These systems often include robotic arms or conveyors to streamline operations and maintain a continuous production flow. This automation not only boosts productivity but also reduces human error, ensuring more consistent part quality.
In addition to the basic components of punch, die, and blank holder, advanced deep draw machines incorporate sensors and real-time monitoring systems to enhance process control. These sensors track key parameters, such as pressure, temperature, and material thickness, throughout the stamping process. By analyzing this data, manufacturers can detect issues early, allowing for timely adjustments to ensure consistent part quality and minimize waste.
The deep draw stamping process is often used in the production of parts with specific requirements such as strength, shape, and material properties. In automotive manufacturing, for example, deep draw stamping is used to create components like body panels, fuel tanks, and other structural elements. The ability to form complex shapes from a single sheet of metal reduces the need for additional processes like welding or assembly, which contributes to lower production costs and higher part strength.
The method also offers significant advantages in terms of material efficiency. Since the process uses a single metal sheet to create a finished part, the material waste is typically minimal, making it more environmentally friendly than other manufacturing processes. For manufacturers focused on reducing costs and improving sustainability, deep drawing offers an attractive solution for producing high-strength, lightweight parts without excessive scrap.
Another notable advantage is the scalability of the deep draw stamping process. Once the tooling is set up and the machine is calibrated, it can produce large quantities of identical parts quickly. This is especially useful in industries that require high-volume production, such as automotive or appliance manufacturing. The efficiency of the deep draw stamping machine ensures that manufacturers can meet high demand while maintaining consistent quality.
Moreover, innovations in machine design continue to improve the capabilities of deep draw stamping machines. Advances in die design, material technology, and automation are making it possible to create even more complex parts with greater precision. Additionally, the integration of smart technologies like artificial intelligence and machine learning into deep draw stamping machines allows for predictive maintenance and real-time optimization of the forming process.
As industries demand lighter, stronger, and more intricately shaped parts, the deep draw stamping machine continues to be a key technology. By adapting to the evolving needs of modern manufacturing, these machines help produce components that meet the highest standards for performance and durability. Whether used for automotive parts, aerospace components, or household appliances, deep draw stamping is a versatile and efficient technique that remains indispensable in high-precision metal forming.
As manufacturing industries continue to demand higher levels of efficiency and precision, deep draw stamping machines are evolving to meet these needs. Innovations in materials, tooling, and machine controls are enhancing the capabilities of deep drawing processes, allowing manufacturers to push the limits of what can be produced from a single sheet of metal.
One significant area of development is the use of advanced materials that push the boundaries of deep drawing capabilities. Manufacturers are increasingly working with high-strength alloys, lightweight metals like aluminum and titanium, and even new composite materials that present unique challenges in forming. These materials often require more delicate handling to ensure they do not fail during the deep drawing process, and this has led to more sophisticated machine controls that can adjust parameters such as pressure, speed, and stroke length on the fly. The ability to form high-strength, lightweight materials efficiently makes deep draw stamping increasingly attractive in sectors like automotive manufacturing, where reducing weight while maintaining strength is crucial.
In addition to material advancements, tooling technology is also improving. Modern deep draw stamping dies are designed with greater precision and longevity. Die materials have become stronger and more durable, reducing wear and tear over time, which can otherwise lead to defects or inconsistent quality. Advanced die coatings and surface treatments further increase the lifespan of tooling, making it more cost-effective and allowing for higher production volumes before the need for maintenance or replacement.
One particularly promising area in deep draw stamping technology is the integration of advanced simulation and digital twins into the design and operation of machines. Digital twins create a virtual model of the machine and the forming process, allowing manufacturers to simulate the entire deep drawing operation before it is executed in real life. This allows for early detection of potential problems, such as wrinkles, thinning, or material failure, and enables engineers to make adjustments to tooling or material choices before physical trials. By leveraging these technologies, manufacturers can optimize tooling designs, material choices, and process parameters to ensure the highest quality and efficiency in production.
Automation and digital control systems have also greatly enhanced deep draw stamping machines’ capabilities. Industry 4.0 technologies are making deep draw presses smarter, with the ability to collect data in real-time, analyze it, and adjust machine settings autonomously. These “smart” presses can monitor a variety of factors, such as punch load, die wear, and material properties, adjusting the process to optimize part quality and prevent defects. The use of predictive maintenance is another key benefit of these digital systems, where machine components are monitored for signs of wear or failure, and maintenance can be performed proactively to avoid downtime and reduce repair costs.
Energy efficiency is another consideration as the demand for sustainable manufacturing increases. Hydraulic deep draw stamping machines are being designed with more energy-efficient systems, and innovations in hydraulic systems, such as regenerative energy recovery, are being incorporated to recover and reuse energy during the press cycle. Some presses are moving toward fully electric solutions, which offer even greater energy savings and more precise control, while also reducing the carbon footprint of the manufacturing process.
In terms of product quality, deep draw stamping machines are now capable of producing parts with extremely tight tolerances and excellent surface finishes. This is especially critical in industries such as aerospace and medical device manufacturing, where part precision is paramount for safety and functionality. Surface finish improvements are achieved through better lubrication, improved die designs, and optimized machine settings that reduce friction between the metal and tooling. This leads to smoother parts with fewer defects and reduces the need for secondary finishing processes.
The future of deep draw stamping is also intertwined with the evolution of hybrid manufacturing processes. For example, combining deep drawing with additive manufacturing (3D printing) could lead to more complex parts that integrate different materials or feature internal structures that are difficult or impossible to achieve with traditional manufacturing methods. This hybrid approach allows manufacturers to take advantage of the strengths of both processes—precision deep drawing for shaping and 3D printing for adding intricate details or supporting structures.
In some industries, multi-material deep drawing has emerged as a technique where different types of metal or composite materials are formed together in a single part. For example, combining a steel exterior with an aluminum core, or using a composite material for weight reduction, is made possible with specialized deep drawing techniques. This could offer even further potential for lightweighting, energy efficiency, and cost reduction, especially in automotive and aerospace sectors where multi-material design is increasingly popular.
Finally, the growing focus on sustainability is driving the development of closed-loop production systems for sheet metal forming. By designing deep draw stamping processes that produce minimal waste and ensure that scrap metal can be fully recycled into the production cycle, manufacturers are contributing to more sustainable industrial practices. In fact, deep drawing is often chosen for its ability to minimize material waste compared to other forming processes like casting or welding, as the process starts with a single sheet and typically results in fewer offcuts.
In summary, sheet metal deep draw stamping machines are evolving at the intersection of material science, digital technology, and automation. These machines are becoming more efficient, precise, and capable of handling an increasing range of materials and complexities. As industries continue to demand stronger, lighter, and more intricate parts, deep draw stamping machines will remain a cornerstone of modern manufacturing, driving innovation and meeting the needs of high-performance applications across multiple sectors.
Sheet Metal Shear Spinning Machine
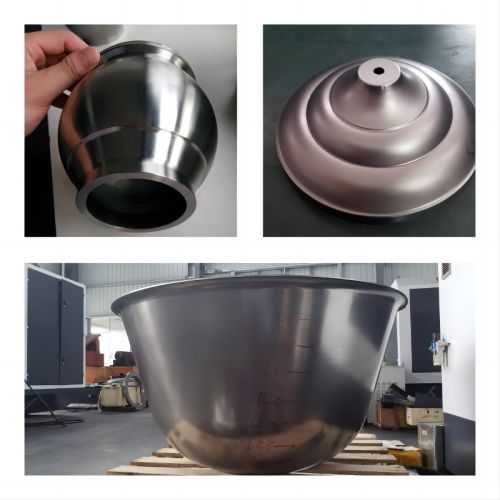
A Sheet Metal Shear Spinning Machine is a specialized machine used for the process of shear spinning, a metal forming technique where a flat sheet of metal is transformed into a hollow, thin-walled part, such as a cone, cylinder, or other rotationally symmetric shapes. This process combines the principles of spinning and shear deformation to shape the metal into the desired form while maintaining material integrity and minimizing waste.
Shear spinning differs from traditional spinning in that it involves a combination of both radial and tangential forces. The metal sheet is clamped on a spinning mandrel, which is then rotated at high speeds. A forming tool, typically a roller or a set of rollers, applies pressure to the metal sheet while it is being rotated. As the sheet is deformed, it stretches and flows over the mandrel, taking on the shape of the die or tooling. The process is often used for creating thin-walled parts, especially in aerospace, automotive, and other industries that require lightweight and high-strength components.
The core components of a shear spinning machine include:
- Spinning Mandrel: The central rotating part of the machine onto which the sheet metal is placed. The mandrel can be a fixed or adjustable diameter, depending on the shape of the final part.
- Forming Tool: A tool (usually a set of rollers or a spinning tool) that applies pressure to the metal sheet as it rotates. The tool shapes the metal by stretching and compressing it to form the desired part.
- Tooling and Dies: Custom-designed dies or forms help control the final shape and dimensions of the part. The tooling design is crucial for achieving the required geometry and surface finish.
- Rotational Speed Control: The rotational speed of the mandrel is carefully controlled to ensure that the material flows evenly and does not become overstretched, which could lead to thinning or cracking.
- Blank Holder: In some shear spinning machines, a blank holder is used to prevent the metal from shifting or wrinkling as it is formed, ensuring that the metal remains in place during the spinning process.
The process begins with a flat sheet of metal, called the “blank,” which is clamped to the mandrel. As the mandrel rotates at high speed, the forming tool is gradually brought into contact with the edges of the sheet. The tool applies radial force, causing the metal to stretch and conform to the shape of the mandrel. The machine may use several stages of deformation, with the tool moving progressively down the length of the mandrel to gradually form the part.
Shear spinning is ideal for producing parts that are both lightweight and strong, such as aerospace components like aircraft fuselage sections, turbine casings, and heat exchangers. The process is also used for parts in automotive industries, such as wheels, housings, and other structural components. One of the main advantages of shear spinning is its ability to produce parts with a uniform thickness, even in complex shapes. The process also allows for tight control over the part’s geometry and surface finish, often eliminating the need for further machining or finishing operations.
Another key benefit of shear spinning is its material efficiency. Because the process stretches and shapes the metal sheet without cutting or removing material, it minimizes waste, making it a cost-effective method for producing parts, especially in high-volume production. Additionally, shear spinning is a relatively quick process compared to other methods like casting or forging, making it ideal for producing large quantities of parts in a short amount of time.
The process also allows for flexibility in material selection. While commonly used with metals like aluminum, copper, steel, and titanium, shear spinning can also be adapted for use with some plastic materials or composite sheets, depending on the part requirements.
One challenge in shear spinning is managing the material flow during the deformation process. If the process is not carefully controlled, there is a risk of uneven thinning or excessive wrinkling, particularly in the areas of the part that experience the greatest deformation. To mitigate this, many shear spinning machines are equipped with sophisticated control systems that monitor and adjust the speed, force, and path of the forming tool in real-time to ensure uniform material distribution and to prevent defects. In high-precision applications, the entire process may be monitored using computer numerical control (CNC) systems, which allow for more intricate, detailed, and repeatable part production.
Additionally, shear spinning machines are often designed to be flexible, allowing for quick changeovers between different part sizes and geometries. Adjustable mandrels and tooling can be swapped out easily, and the machine’s settings can be modified to accommodate different materials and thicknesses. This makes shear spinning machines suitable for both small batch production and large-scale manufacturing.
The shear spinning machine’s role in industries that require both precision and strength is becoming more critical as design requirements become more complex. Aerospace manufacturers, for instance, rely on shear spinning to produce lightweight, high-strength parts for aircraft. In automotive production, shear spinning is increasingly being used to create structural components that need to withstand high stress while reducing weight to improve fuel efficiency.
In conclusion, a sheet metal shear spinning machine is an advanced piece of manufacturing equipment that allows for the production of high-quality, lightweight, and precise metal parts. Its ability to form thin-walled parts from a single sheet of material while maintaining strength and minimizing waste makes it indispensable in industries like aerospace, automotive, and more. With continuous advancements in technology, shear spinning is expected to play an even greater role in meeting the demand for complex, high-performance parts.
Shear spinning machines continue to advance, especially as industries demand even higher precision, material efficiency, and flexibility. The growing use of automation and digital control systems is making these machines more adaptable and capable of handling a wider range of materials and part geometries. One significant trend is the integration of Industry 4.0 technologies, which allows manufacturers to optimize production through real-time data collection, predictive analytics, and automated adjustments. This can greatly improve the speed and accuracy of the process, reducing scrap and ensuring consistent part quality.
In addition, advanced materials like high-strength alloys, composites, and lightweight metals like titanium and aluminum are increasingly being used in shear spinning. These materials require careful management during the forming process to avoid defects like cracking or excessive thinning. With newer machines that incorporate adaptive control systems and sophisticated sensors, the machine can detect material behavior during the process and adjust the parameters dynamically to ensure a flawless result.
The ability of shear spinning machines to handle multiple materials and complex shapes means they are highly versatile. Many modern machines allow for quick tool changes and adjustments to accommodate different material thicknesses and part configurations. This makes them ideal for industries that require flexibility, such as automotive and aerospace, where designs frequently change and parts must be produced in both small batches and large volumes.
The tooling used in shear spinning machines has also evolved. Newer tooling systems are designed for better wear resistance, reducing maintenance downtime and increasing production efficiency. The design of tooling and dies has become more sophisticated, allowing for greater precision in the final part shape and surface finish. In some cases, tooling systems are designed to reduce or eliminate the need for secondary operations, such as trimming or finishing, by improving the accuracy of the part right off the machine.
The role of sustainability in manufacturing is also shaping the future of shear spinning. As companies focus more on reducing their environmental impact, processes that minimize material waste and energy consumption are highly valued. Shear spinning, by its nature, is material-efficient because it uses a single sheet of metal and stretches it to form the part, rather than cutting away excess material as in traditional machining. This makes it a preferred choice for producing complex parts without excessive scrap, which is especially important in industries that deal with expensive or rare materials.
Furthermore, composite materials are starting to be explored in shear spinning for applications where metal alone may not suffice. In these cases, the machine can be adapted to handle not just traditional metals but also some types of plastics and composites, opening up new opportunities for the production of lightweight yet strong parts in industries like electronics, medical devices, and renewable energy sectors.
As the demand for lightweight and high-performance components grows, especially in industries like aerospace and automotive, shear spinning continues to be a valuable technology. It allows for the production of parts that are both strong and lightweight, meeting the increasing requirements for fuel efficiency, structural integrity, and performance. By offering a fast, cost-effective way to produce parts with uniform thickness and minimal waste, shear spinning remains an integral process in modern manufacturing, particularly in the production of parts with rotational symmetry.
Looking ahead, the future of shear spinning will likely see more integration with additive manufacturing (3D printing) for hybrid processes, which could enable even more complex and customized part designs. For example, parts could be partially produced using additive techniques, with areas of the part that require specific material properties or geometries then completed using shear spinning. This hybrid approach could bring together the strengths of both technologies, enabling the creation of parts with even more intricate features, optimized material distribution, and reduced weight.
In conclusion, sheet metal shear spinning machines are becoming increasingly sophisticated, offering greater versatility, precision, and efficiency. As technology continues to advance, these machines will play an even more significant role in producing high-quality, lightweight, and durable parts across a wide range of industries, from aerospace to automotive and beyond. The integration of automation, digital control, and adaptive technologies will make shear spinning more efficient and adaptable to the ever-changing demands of modern manufacturing.
As the manufacturing landscape evolves, the capabilities of sheet metal shear spinning machines are expanding, particularly with the integration of artificial intelligence (AI) and machine learning. These technologies allow machines to not only monitor the forming process in real time but also to predict potential failures or deviations in material behavior before they occur. For example, AI algorithms can analyze historical data from the machine to identify patterns and predict when maintenance might be required, helping to minimize downtime and reduce operational costs. This level of predictive maintenance ensures that parts are produced with high precision and quality, while also extending the lifespan of the machine itself.
The data-driven manufacturing trend is also gaining traction, with shear spinning machines becoming more connected to larger smart factories. By linking to central systems that collect and analyze data from multiple machines across a facility, manufacturers can better optimize their processes for efficiency and consistency. This connected approach helps manufacturers identify areas of improvement, streamline production workflows, and achieve higher levels of automation, which is especially beneficial in high-volume production environments.
The demand for customization in parts is another area where shear spinning machines are increasingly being used. As industries like aerospace and automotive require more bespoke components to meet specific performance standards, shear spinning offers the flexibility to produce complex parts with tight tolerances and unique geometries. The ability to make rapid adjustments to tooling and machine settings allows for quick reconfiguration and production of one-off or small-batch parts without significant downtime or cost.
Additionally, there is an ongoing push toward process integration, where shear spinning is combined with other forming techniques such as hydroforming or deep drawing. These integrated processes enable the creation of even more intricate parts that require multiple forming stages. For instance, a part may be partially formed using shear spinning to create a thin-walled section, then further processed with hydroforming to achieve more complex internal geometries or increased strength. The combination of processes provides a high level of flexibility and enables manufacturers to meet the increasing demands for lightweight, high-strength parts with advanced material properties.
Another emerging trend is the use of sustainable materials in shear spinning. As industries focus on reducing their environmental impact, there is a growing interest in using renewable and eco-friendly materials in production. This can include recycled metals or biocomposites, which can be formed using shear spinning machines. While these materials may require special handling or adjustments to process parameters, the potential benefits in terms of sustainability and cost reduction make them a promising choice for the future of manufacturing.
The versatility of shear spinning machines also makes them suitable for applications outside traditional metalworking industries. For example, medical device manufacturing has begun to leverage shear spinning for creating complex parts like implants, surgical instruments, and prosthetics. The ability to form thin, precisely shaped parts is essential in the medical field, where part strength, durability, and biocompatibility are critical. As medical technologies advance, shear spinning may play a greater role in producing the next generation of cutting-edge medical devices.
Customization and precision are also crucial in electronics manufacturing, where parts must meet specific performance requirements in compact, lightweight, and thermally efficient designs. Shear spinning enables the creation of thin-walled casings or housings for electronic components, ensuring that they are both lightweight and strong while minimizing material waste. This process, combined with other advanced techniques like laser welding and additive manufacturing, allows for the production of parts with complex internal geometries that would be difficult or impossible to achieve with traditional methods.
Furthermore, shear spinning continues to make strides in improving energy efficiency in manufacturing processes. As energy costs rise and sustainability becomes an even greater priority for manufacturers, the ability to produce parts with minimal material waste and lower energy consumption is becoming increasingly important. The inherent efficiency of shear spinning, which uses a single sheet of material and minimizes scrap, aligns well with these goals. Moreover, the ongoing development of more energy-efficient machines—whether through advancements in drive systems, better lubrication techniques, or optimized cycle times—will contribute to further reductions in energy usage.
As global competition in industries like automotive and aerospace intensifies, manufacturers are seeking every opportunity to reduce costs while improving product quality. Shear spinning offers an ideal solution by producing parts with minimal waste, reducing the need for extensive post-processing, and allowing for rapid prototyping and low-volume production. The ability to easily adjust machine settings and tooling configurations also makes shear spinning an attractive option for manufacturers who need to quickly adapt to changing market demands or customer specifications.
The integration of robotics into shear spinning processes is another area of growth. Robotics can be used for material handling, tool changes, and even inspection tasks, ensuring that parts are formed and processed with minimal human intervention. This improves not only the speed of production but also the consistency and precision of the parts being produced. Automated quality control systems, often integrated with vision systems or AI-based monitoring, further ensure that parts meet strict tolerance and surface finish requirements.
In the long term, additive manufacturing techniques like 3D printing may play an increasingly significant role in combination with shear spinning. For example, 3D printing could be used to create intricate internal structures or features that are difficult to achieve with traditional forming methods. The combination of additive and shear spinning could lead to more innovative and efficient manufacturing techniques, offering designers and engineers greater flexibility in part design and production.
Ultimately, the future of sheet metal shear spinning machines is tied to the ongoing demands for innovation, sustainability, and efficiency in manufacturing. As industries evolve, shear spinning will continue to be a key technology in producing high-performance parts, particularly in industries where weight reduction, material strength, and precision are critical. The integration of digital control, automation, and advanced materials will only further enhance the capabilities of shear spinning, positioning it as an even more integral part of the modern manufacturing landscape.
Sheet Metal Transfer Die Forming Machine
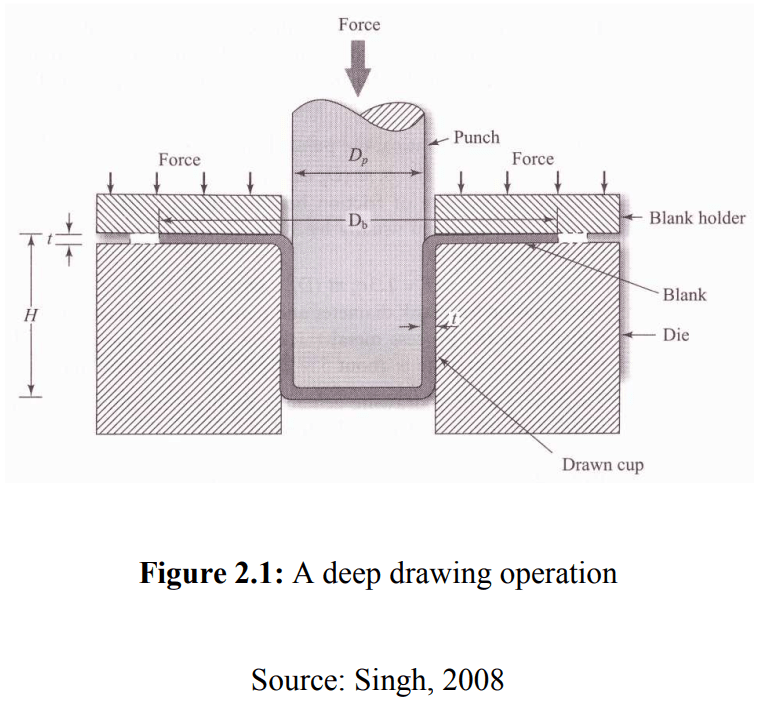
A Sheet Metal Transfer Die Forming Machine is a type of metal forming equipment used to shape and form metal sheets into parts with complex geometries. It is often used in high-volume manufacturing processes, especially in industries like automotive, aerospace, and electronics, where precision and consistency are crucial. The machine is designed to carry out the entire forming process using a transfer die system, where the workpiece is transferred between multiple stations in the die set to perform various stages of forming, blanking, punching, bending, and drawing.
The transfer die forming process involves several distinct stations or steps that are integrated into one machine, allowing for the production of multi-step parts in a continuous, automated fashion. Here’s a breakdown of how the process works and the key features of a transfer die forming machine:
- Blanking and Feeding: The process starts with the feeding of a flat metal sheet, or blank, into the machine. The blank is positioned in the first station, where it is punched or cut to shape. The machine uses a feeder mechanism to transfer the blank through the various stations of the die, which is necessary for performing subsequent operations like bending, drawing, or punching.
- Multiple Stations: The machine is equipped with a series of die stations that perform different forming operations on the sheet metal. These stations are typically arranged in a sequence, each one contributing a specific function such as blanking, stamping, bending, piercing, drawing, or trimming. The workpiece is transferred from one station to the next using a system of transfer fingers or conveyors.
- Transfer Mechanism: The key feature of a transfer die forming machine is its transfer mechanism, which moves the sheet metal from one station to another. This mechanism can include mechanical, pneumatic, or hydraulic actuators that ensure accurate positioning of the workpiece at each station. The transfer system also allows for precise control over the timing and positioning of the blank, which is critical for producing high-quality parts with tight tolerances.
- Tooling and Dies: The dies used in transfer die forming machines are specifically designed to perform multiple functions in a single operation. These tools are often made from high-strength steel and are designed to withstand the pressures of metal forming. Custom tooling is often used to ensure the part is formed to the required shape, size, and finish, with minimal waste or distortion.
- Pressing and Forming: The press used in the transfer die forming process provides the force required to shape the material. It applies pressure to the metal sheet through the dies, causing it to conform to the desired shape. Depending on the part design and material, different types of presses, such as hydraulic presses or mechanical presses, can be used to provide the necessary force.
- Automation and Precision: Transfer die forming machines are often fully automated, with computer numerical control (CNC) systems controlling each station’s operations. Automation ensures high production rates, minimal human intervention, and greater consistency in part quality. The precision of the machine also reduces the risk of defects and increases part accuracy, which is especially important in industries that require tight tolerances, such as automotive and aerospace.
- Part Transfer and Handling: Once the part has been formed through the various stages of the die, it is transferred to the next phase of production. This could involve a final trimming step, assembly, or quality inspection. Depending on the part complexity, a robotic system or conveyor belts might be used to transfer the finished parts to the next stage of production.
The main advantage of sheet metal transfer die forming machines is their ability to produce complex parts in a continuous, automated process with high efficiency. This system is especially useful in high-volume production runs, where it can quickly produce large quantities of parts without sacrificing quality or precision. The high automation also reduces labor costs and ensures consistent output.
Some of the key benefits of this technology include:
- High Productivity: Due to its automated nature, a transfer die forming machine can handle large volumes of parts with minimal downtime, making it ideal for industries that require mass production.
- Complex Part Geometries: The use of multiple die stations allows for the production of intricate and multi-step parts that would be difficult or impossible to create using traditional stamping methods.
- Minimal Waste: The precision of the machine and the ability to transfer the blank between stations reduces material waste, making the process more efficient and cost-effective.
- Consistency and Precision: The automation and precision of the machine ensure that each part is formed with consistent quality, reducing variation and ensuring that parts meet the required specifications.
- Cost Efficiency for Large Batches: While the initial investment in a transfer die forming machine may be high, the machine’s ability to handle large batches at high speeds makes it cost-effective over time for high-volume production runs.
Transfer die forming is commonly used in industries such as:
- Automotive Industry: For producing parts like body panels, brackets, and structural components.
- Aerospace Industry: For creating parts like panels, brackets, and complex enclosures.
- Electronics: For manufacturing housings, brackets, and other intricate components.
- Consumer Goods: For producing various parts in appliances, hardware, and other products.
However, one of the challenges of transfer die forming is the initial setup cost. The creation of custom tooling and dies can be expensive, and the machine itself requires a significant capital investment. Despite these costs, the ability to produce parts with high precision and at high speeds makes the investment worthwhile for many manufacturers, especially in industries where production volumes are high.
Another consideration is the complexity of the part design. If the part has highly intricate geometries or requires multiple stages of forming, the die set may need to be specially designed, which can increase lead time and costs. Additionally, any changes to part design may require modifications to the tooling or die set, which can also impact production schedules.
Overall, a sheet metal transfer die forming machine is a highly efficient, versatile, and precise tool used in manufacturing to produce complex metal parts at high volumes. With its automated processes, minimal waste, and ability to handle intricate designs, it is an essential piece of equipment in many industries where precision, speed, and cost-effectiveness are paramount.
Sheet metal transfer die forming machines are becoming increasingly important in industries where precision and high-volume production are critical. The automated nature of these machines allows for rapid production of parts, reducing labor costs while ensuring that parts are formed with tight tolerances and minimal defects. The use of advanced control systems, such as CNC (Computer Numerical Control), has further enhanced the machine’s ability to perform complex operations with minimal manual intervention, allowing for greater consistency across large production runs.
The machine’s ability to transfer workpieces between multiple die stations allows for the creation of parts that would be impossible to produce in a single stamping operation. This is particularly useful when dealing with complex parts that require multiple forming steps, such as bending, punching, and drawing. With each stage of the forming process happening in a different station, the part can gradually take shape without the need for multiple passes through separate machines.
Transfer die forming machines are also known for their ability to handle a wide range of materials, from standard metals like steel, aluminum, and copper to advanced alloys and composite materials. This versatility is important in industries like automotive and aerospace, where the materials used in components often need to meet specific strength, weight, and performance criteria. The machines can be adjusted to accommodate different material thicknesses, ensuring that even delicate or high-strength materials are formed without damage or distortion.
One of the main challenges when working with a transfer die forming machine is ensuring that the transfer mechanism is reliable and precise. If the sheet metal is not properly aligned as it moves through the various stages of the die, it can lead to defects such as warping, dimensional inaccuracies, or part misalignment. As a result, it is crucial to maintain and calibrate the transfer system regularly to ensure it remains accurate and efficient. The integration of robotic systems or automated handling mechanisms further ensures that parts are consistently positioned as they move through each station.
Despite the initial cost of tooling and die creation, the long-term benefits of using transfer die forming machines become apparent when considering the high-speed production rates and minimal material waste. The precision with which these machines operate reduces scrap and rework, which helps keep costs low and production timelines tight. In industries where large volumes of parts are required, such as automotive manufacturing, the ability to produce high-quality parts quickly and efficiently can give manufacturers a significant competitive advantage.
As industries continue to demand lighter, stronger, and more complex components, transfer die forming machines are expected to evolve with advancements in technology. For instance, the integration of additive manufacturing (3D printing) with transfer die forming could provide new opportunities for producing even more intricate parts that would otherwise be challenging to produce through traditional forming methods. Additionally, as the push for sustainability grows, manufacturers may explore the use of recycled materials or more energy-efficient processes in transfer die forming, making the process not only cost-effective but also environmentally friendly.
With continuous advancements in automation, materials science, and machine control, sheet metal transfer die forming machines will continue to be a key technology in industries that rely on high-precision, high-volume manufacturing. As these machines become even more capable and adaptable, they will play an essential role in producing the next generation of components for a wide range of applications, from automotive to aerospace and beyond.
As technology continues to advance, sheet metal transfer die forming machines are becoming more sophisticated, integrating features like artificial intelligence (AI) and machine learning to further enhance their capabilities. AI can help improve the predictive maintenance of the machines, reducing downtime and optimizing production schedules. Machine learning algorithms can analyze patterns from past production runs to predict future behavior, such as wear on tooling or changes in material properties. This predictive approach allows manufacturers to proactively address issues before they affect production quality or timelines, ultimately saving time and money.
Moreover, IoT (Internet of Things) integration is allowing for even greater control and monitoring. With IoT-enabled machines, manufacturers can track real-time data from the production process, such as temperature, pressure, and speed. This real-time information can be used to make adjustments on the fly, ensuring that the part quality remains consistent throughout the production cycle. The ability to adjust machine parameters based on real-time data helps ensure that parts are produced with optimal material usage and without defects, enhancing overall productivity.
Energy efficiency is also becoming a priority in the design of modern transfer die forming machines. Manufacturers are increasingly focusing on ways to reduce energy consumption throughout the forming process, which is particularly important in high-volume production environments. Advances in energy recovery systems and more efficient drive motors are making machines more energy-efficient, which not only lowers operational costs but also aligns with the growing emphasis on sustainability in manufacturing. Machines are also being designed to operate with fewer emissions, contributing to a cleaner manufacturing process.
Another notable trend is the use of advanced materials in the die set and tooling. As manufacturers push for lighter, stronger, and more durable materials, tools and dies used in transfer die forming are evolving to withstand higher stresses and temperatures. The use of coated dies and wear-resistant materials increases tool life, reduces maintenance costs, and improves overall production efficiency. This is especially important when working with high-strength materials like titanium or advanced alloys, which require specialized tooling to maintain the precision and integrity of the parts being formed.
The ongoing demand for customization in manufactured parts is also driving the evolution of transfer die forming technology. In industries such as aerospace, where parts need to be tailored to specific performance characteristics, the ability to customize tooling quickly and effectively becomes essential. This is where digital technologies come into play—advanced CAD (Computer-Aided Design) and CAM (Computer-Aided Manufacturing) software allow for the rapid development of customized tooling, reducing the lead time needed to bring new parts to production. This level of customization enables manufacturers to meet the specific needs of their customers more efficiently, offering faster turnaround times and the flexibility to adjust to market demands.
The future of transfer die forming machines will likely involve even deeper integration with additive manufacturing techniques. For example, 3D printing could be used to create more complex geometries or lightweight features in parts, which could be formed in tandem with traditional die forming. A hybrid approach would enable manufacturers to push the boundaries of what’s possible in part design, creating lighter, stronger, and more efficient parts without the constraints of traditional forming methods. This synergy could lead to the production of complex metal components with intricate internal structures that reduce material waste and improve performance.
In terms of sustainability, another major focus for transfer die forming is the reduction of material waste. Since the process involves feeding a flat sheet of metal into the die, there is often leftover material. However, advanced nesting algorithms in modern machines can optimize material layout to minimize scrap. This makes the process more cost-effective and environmentally friendly, as less material is wasted, and recycling of scrap metal can be incorporated into the production process. Additionally, the trend towards using recycled metals in manufacturing continues to grow, and transfer die forming machines can be designed to accommodate a wider range of recycled materials, further enhancing the sustainability of the process.
The automation of transfer die forming machines continues to evolve as well. Not only are these machines becoming more precise and faster, but the ability to integrate them into fully automated production lines is allowing manufacturers to achieve unprecedented levels of efficiency. Fully automated production lines can handle everything from raw material input to finished product output, with minimal human intervention. This reduces labor costs and human error, while also increasing throughput and reducing cycle times.
One area where transfer die forming machines show great potential is in the production of parts for electric vehicles (EVs) and hybrid vehicles. The shift towards lighter, more efficient components in the automotive sector has created demand for high-strength, lightweight metal parts. Transfer die forming can help meet these demands by producing parts that are both strong and light, such as battery enclosures, structural frames, and exterior panels. The ability to quickly adapt to changing designs and materials makes transfer die forming ideal for industries that are undergoing rapid innovation, like electric vehicles.
In conclusion, sheet metal transfer die forming machines are evolving rapidly to meet the demands of modern manufacturing. As they incorporate advanced technologies like AI, IoT, and energy-efficient designs, these machines are becoming more precise, faster, and cost-effective. The ability to handle complex parts with minimal material waste, combined with the ongoing advancements in tooling, automation, and hybrid manufacturing processes, ensures that transfer die forming will remain a key technology in industries ranging from automotive to aerospace, electronics, and beyond. As the focus on sustainability and customization grows, these machines will continue to adapt, providing manufacturers with the tools they need to stay competitive in a rapidly changing market.
Sheet Metal Hot Forming Machine
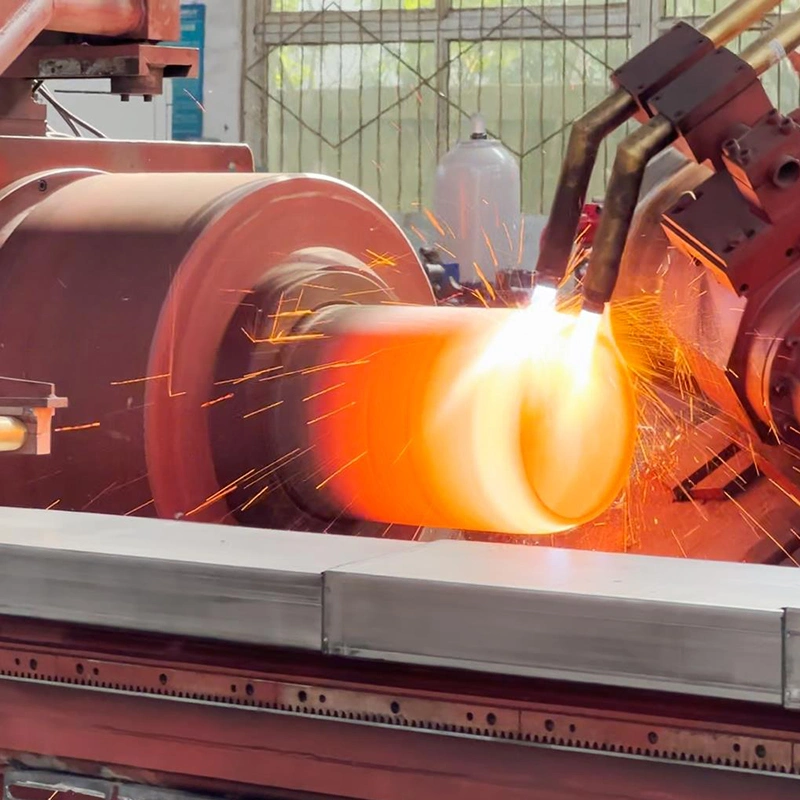
A Sheet Metal Hot Forming Machine is a type of industrial equipment used to shape metal sheets through high-temperature processes. This method is commonly employed to manufacture parts that require complex geometries, precise dimensions, and enhanced material properties, especially for metals that are difficult to form at room temperature. Hot forming processes are widely used in industries such as automotive, aerospace, and heavy machinery, where strength, durability, and material performance are critical.
The primary principle of hot forming is to heat the metal to a temperature that makes it more malleable and easier to shape. This reduces the amount of force required during the forming process, allows for more intricate shapes, and can improve the mechanical properties of the material, such as hardness and tensile strength. This technique is often used for materials like steel, aluminum, titanium, and other high-performance alloys that become more ductile and easier to shape at elevated temperatures.
How Hot Forming Works:
- Heating: The process starts with heating the metal sheet to a specific temperature, typically above its recrystallization temperature. This is a critical step, as the temperature must be carefully controlled to ensure the metal is soft enough to be formed but not too hot to degrade its material properties or cause oxidation. The temperature depends on the material being used; for example, steel may be heated to temperatures between 900°C to 1,200°C, while aluminum requires lower temperatures (around 350°C to 500°C).
- Forming: Once the material reaches the desired temperature, it is transferred to the forming station. In this station, the sheet metal is shaped using dies, presses, or molds that apply mechanical force. This force can be applied using various techniques such as stamping, extrusion, or deep drawing. The hot metal is more pliable, so less force is needed to achieve the desired shape, allowing for the creation of intricate designs and geometries that would be challenging to achieve with cold-forming methods.
- Cooling: After the metal has been formed, it is rapidly cooled, often through water quenching or air cooling, depending on the desired material properties. The cooling rate can influence the final mechanical characteristics of the part, such as its hardness, strength, and ductility. In some cases, the cooling process is controlled precisely to achieve specific mechanical properties, like in the production of high-strength steel components.
Types of Hot Forming Processes:
Several types of hot forming processes can be performed with these machines, including:
- Hot Stamping: Hot stamping is a process where the metal sheet is heated to a high temperature and then pressed into a die to form the part. This method is commonly used in the automotive industry for producing high-strength parts like body panels, structural components, and crash protection parts. The process enables the use of high-strength materials such as boron steel, which become stronger after being formed at high temperatures.
- Hot Rolling: In hot rolling, a metal slab or billet is heated and passed through rollers to reduce its thickness and shape it into sheet metal or other forms. The process is typically used in steel and aluminum manufacturing to produce large quantities of material for further processing.
- Hot Forging: Hot forging involves heating the metal to a high temperature and then shaping it by applying compressive forces, typically using a hammer or press. This process is used for producing parts that require high strength and toughness, such as components for aerospace and automotive applications.
- Hot Extrusion: In hot extrusion, the heated metal is forced through a die to create parts with a consistent cross-sectional profile. This process is commonly used for producing rods, tubes, and complex shapes in industries like aerospace and construction.
Key Benefits of Hot Forming Machines:
- Increased Formability: The most significant advantage of hot forming is that it allows the formation of complex parts that would otherwise be difficult or impossible to produce at room temperature. The elevated temperatures reduce the material’s yield strength, making it more pliable and easier to shape.
- Improved Material Properties: Hot forming can enhance certain material properties, such as improving the hardness, strength, or ductility of the metal. For example, hot stamping of high-strength steels can increase the part’s strength while maintaining its toughness.
- Reduced Force Requirements: Since the material becomes softer at high temperatures, less mechanical force is needed to shape the material. This reduces the wear and tear on tools and presses, extending their lifespan and reducing operational costs.
- Precision and Complexity: Hot forming allows for the creation of intricate, detailed parts with tight tolerances. This is particularly beneficial for industries such as automotive and aerospace, where part complexity and precision are essential for functionality and safety.
- Energy Efficiency: While heating metal to high temperatures requires energy, the ability to form more complex shapes with fewer steps or tools can lead to more energy-efficient processes overall. Additionally, some machines are designed with energy recovery systems that capture and reuse heat, making the process more sustainable.
Applications of Hot Forming Machines:
- Automotive Industry: Hot forming is widely used in automotive manufacturing to produce high-strength parts such as crash protection components, chassis parts, and body panels. The ability to form parts from high-strength steel or aluminum allows manufacturers to reduce vehicle weight while maintaining or increasing the structural integrity and safety of the vehicle.
- Aerospace Industry: Hot forming is used to create lightweight and strong components for aircraft, including structural parts, engine components, and fuselage sections. The high strength-to-weight ratio achieved through hot forming is critical for aerospace applications, where performance and safety are paramount.
- Heavy Machinery: Parts used in construction equipment, industrial machinery, and tools often require hot forming due to their complex geometries and the need for high strength. Hot forming processes allow manufacturers to create durable, long-lasting components capable of withstanding extreme conditions.
- Medical Devices: Some medical components, such as implants, surgical instruments, and prosthetics, are made using hot forming processes. This is particularly useful when working with specialized metals like titanium or alloys that require specific mechanical properties to be safe and effective for medical use.
Challenges and Considerations:
- Energy Consumption: Heating the metal to high temperatures requires substantial energy, which can increase operational costs. Manufacturers are continually looking for ways to improve energy efficiency in the hot forming process, such as by using more efficient heating methods or incorporating waste heat recovery systems.
- Tool Wear: High temperatures can cause significant wear and tear on the dies and molds used in the forming process. As a result, regular maintenance and the use of durable, heat-resistant materials for tooling are essential to ensure consistent quality and minimize downtime.
- Material Oxidation: At high temperatures, metals are more susceptible to oxidation, which can degrade the quality of the material and the final part. Special coatings, controlled atmospheres, or vacuum conditions are often used to prevent oxidation and ensure high-quality parts.
- Cost of Equipment: The initial investment in hot forming machinery can be high, especially for specialized equipment designed to handle high-temperature processes. However, the long-term benefits of increased efficiency, reduced material waste, and higher-quality parts often outweigh the initial costs.
Conclusion:
The Sheet Metal Hot Forming Machine is a critical piece of equipment in modern manufacturing, particularly in industries where high-strength, lightweight, and complex parts are required. With its ability to shape materials at elevated temperatures, this technology offers significant advantages in terms of part complexity, material properties, and overall production efficiency. As materials and technologies continue to evolve, the hot forming process will remain a vital tool in the manufacturing of advanced components for a wide range of industries.
Modern sheet metal hot forming machines are becoming more intelligent and adaptable, thanks to the integration of digital technologies and automation. Advanced control systems now monitor temperature, pressure, and forming force in real time, enabling extremely precise control over the entire process. This not only improves the quality and consistency of formed parts but also allows operators to make immediate adjustments during production, reducing the likelihood of errors or defects. With the rise of Industry 4.0, many hot forming systems are now part of fully connected smart manufacturing environments, where data collected from each machine feeds into a larger system for analytics, predictive maintenance, and overall production optimization.
Another important advancement in these machines is the development of hybrid forming systems that combine hot and cold forming techniques in a single cycle. For example, certain areas of a part can be hot formed for maximum strength, while others are cold formed to maintain ductility or allow for additional machining. These hybrid approaches are especially useful in automotive applications, where parts must balance strength and flexibility across different sections of the same component. This opens up new design possibilities and reduces the need for welding or joining multiple pieces, resulting in stronger, lighter assemblies.
The materials used in tooling for hot forming machines are also evolving. Traditional tool steels are being supplemented or replaced by advanced alloys and ceramic-based materials that offer better thermal resistance and wear characteristics. This not only extends the life of the tooling but also reduces maintenance requirements and downtime. In environments where uptime and part accuracy are critical, such as aerospace or defense manufacturing, even small improvements in tooling performance can translate to significant gains in overall productivity.
In addition to aerospace and automotive, hot forming is gaining ground in industries such as energy, shipbuilding, and even consumer electronics. In the energy sector, components for wind turbines, heat exchangers, and power plants often require materials with high temperature resistance and complex shapes. Hot forming provides a reliable method for manufacturing these parts without compromising material integrity. In electronics, miniaturized structural components made from lightweight alloys are increasingly being hot formed to meet both aesthetic and functional demands.
Safety is another area receiving considerable attention in the design and operation of modern hot forming machines. Since the process involves extremely high temperatures and pressures, robust safety systems are essential to protect operators and equipment. Modern machines feature automated handling systems, temperature shields, real-time monitoring of key parameters, and emergency shutoff mechanisms. Many manufacturers also invest in comprehensive operator training programs to ensure that workers can safely and efficiently operate the machinery.
Sustainability is becoming a driving force in the evolution of hot forming machines. While these machines do consume significant energy during the heating phase, innovations are helping to offset this by improving thermal efficiency and enabling the use of renewable energy sources in manufacturing facilities. Additionally, because hot forming can reduce material waste and produce stronger, lighter parts, it contributes to more sustainable end products—particularly in transportation industries, where reduced vehicle weight leads to lower fuel consumption and emissions.
As global manufacturing continues to move toward more advanced, high-performance materials and precision-driven production standards, sheet metal hot forming machines will remain at the forefront of innovation. Their ability to form complex geometries, tailor material properties, and integrate with automated production systems makes them indispensable tools for modern industry. The next generation of hot forming machines will likely push even further—leveraging artificial intelligence, real-time quality control, and adaptive forming strategies to meet the ever-growing demands of efficiency, customization, and performance.
Looking ahead, the role of simulation and virtual prototyping in hot forming is becoming more prominent. Before any physical forming begins, engineers can now run detailed simulations of the hot forming process using advanced software tools. These simulations allow for the prediction of metal flow, temperature distribution, material thinning, and potential failure points. By validating the forming process in a virtual environment, manufacturers can optimize die design, reduce trial-and-error tooling, and significantly cut down on development time and costs. This virtual approach also supports rapid prototyping, helping companies bring products to market faster while ensuring high quality and consistency from the first production run.
The integration of robotics into hot forming systems is also accelerating, especially in material handling and post-forming operations. Robotic arms equipped with heat-resistant grippers can move hot blanks with precision from furnaces to presses and then to cooling stations, all without human intervention. This not only enhances safety in high-temperature environments but also increases cycle speed and consistency. In some advanced facilities, entire hot forming lines are fully automated, with robots coordinating every step of the process, including die lubrication, part extraction, and quality inspection via machine vision systems.
Quality control itself is evolving with technologies like laser scanning, ultrasonic testing, and thermal imaging now commonly used to inspect hot-formed parts in real-time. These non-contact methods can detect surface defects, dimensional deviations, or internal inconsistencies without damaging the part, ensuring only components that meet strict standards move forward in the production process. This level of automated quality assurance is critical in applications where failure is not an option, such as aircraft structural components or safety-critical automotive parts.
Customization is another frontier for hot forming. As industries like aerospace and defense increasingly demand bespoke parts made from exotic alloys or with highly specific performance characteristics, hot forming is adapting with greater flexibility. Machines can now be configured quickly for short production runs or to accommodate unusual geometries, making them suitable not only for mass production but also for high-mix, low-volume environments. The ability to adapt forming parameters on the fly—such as adjusting press force, die temperature, or forming speed—enables precise tuning of material properties for specialized applications.
From an economic standpoint, advancements in energy management are also helping to make hot forming more accessible to a wider range of manufacturers. Induction heating, for example, is being adopted as a more efficient alternative to traditional gas-fired furnaces. Induction systems can heat metal blanks faster and more uniformly, and they can be turned on and off rapidly, reducing idle energy consumption. Combined with thermal insulation improvements and regenerative heat systems, these innovations are significantly reducing the energy footprint of hot forming operations.
Ultimately, the continuous development of sheet metal hot forming machines reflects the broader trends shaping modern manufacturing: smarter systems, cleaner processes, and more adaptive capabilities. As industries demand lighter, stronger, and more efficient products—whether it’s for vehicles, aircraft, power systems, or even advanced consumer devices—hot forming is increasingly the method of choice for shaping materials to meet those goals. It’s a field where metallurgy, automation, and digital intelligence converge, and its future looks to be just as hot as the processes it uses.
Sheet Metal Nibbling Machine
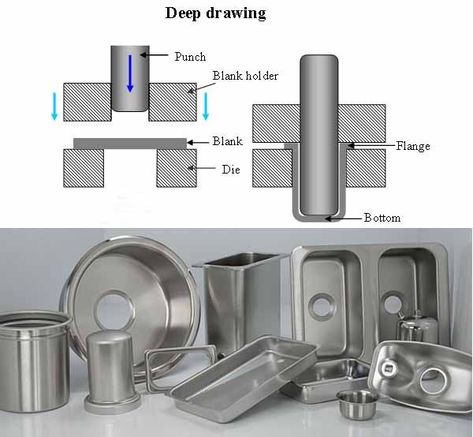
A Sheet Metal Nibbling Machine is a precision fabrication tool used to cut complex shapes or intricate patterns into sheet metal. It operates by rapidly punching a series of small, overlapping holes in the material, effectively “nibbling” away the unwanted metal to create the desired contour or cutout. This method is particularly useful for producing irregular shapes, internal cutouts, and curves that are difficult to achieve with traditional shearing or punching tools.
The nibbling process is highly versatile and allows for great flexibility in design, making it ideal for prototype work, short-run production, and applications where custom shapes are needed without the expense of dedicated dies. The machine uses a punch-and-die mechanism, where a small punch repeatedly moves up and down through the sheet, guided by either a CNC-controlled table or a manually guided carriage. The spacing and speed of the punches can be adjusted based on material thickness, type, and the desired finish quality.
One of the major advantages of a nibbling machine is its ability to cut inside contours and produce slots or perforations without requiring a pre-drilled pilot hole. The punch can start from the middle of the sheet, offering unmatched access and flexibility compared to traditional cutting tools. Additionally, since nibbling removes only a small amount of material with each stroke, it generates less heat and distortion, preserving the flatness and structural integrity of the workpiece.
Modern nibbling machines often come equipped with CNC (Computer Numerical Control) systems, enabling automated, high-precision cutting based on digital designs. This makes them suitable for use in industries such as electrical enclosures, metal signage, HVAC components, and custom panels. Some machines also feature interchangeable punch shapes, allowing operators to quickly switch between round, square, or custom geometries without extensive retooling.
Despite its strengths, nibbling does leave a slightly rough edge or “tooth mark” finish due to the overlapping punch marks. However, this can be smoothed through secondary processes like deburring or grinding if a cleaner edge is required. The process is best suited for thin to moderately thick sheets—typically up to 6mm—depending on the machine’s power and tooling.
With ongoing developments in automation, tooling durability, and CNC integration, sheet metal nibbling machines continue to be a valuable tool for fabricators who need to produce detailed and varied parts without committing to the high cost and lead time of custom dies. Their adaptability, efficiency in small-batch production, and ability to handle complex internal cuts make them an essential part of any modern sheet metal shop.
Modern sheet metal nibbling machines have evolved significantly with the integration of CNC technology, making them capable of executing extremely complex patterns with high accuracy and repeatability. Instead of manually guiding the sheet or relying on templates, operators now use CAD-generated designs to control the punch head’s movement, allowing for precise and consistent results across multiple parts. This digital control also makes it easy to modify or update a design without the need for retooling, which is especially valuable in prototyping environments or custom fabrication shops where flexibility is key.
In terms of speed, while nibbling may be slower than laser or plasma cutting for large production runs, it excels in situations where setup time and tooling costs need to be kept to a minimum. Unlike stamping, which requires a separate die for every shape, nibbling uses a single punch that moves incrementally to achieve the desired outline. This makes it especially effective for low- to medium-volume production where agility and cost control matter more than high throughput.
Noise and vibration are common characteristics of the nibbling process due to the rapid, repetitive punching action. However, modern machines are equipped with noise-reduction features and vibration-dampening structures to enhance operator comfort and reduce wear on components. Safety systems are also built into contemporary designs, including automatic shut-offs, protective guarding, and programmable feed systems that reduce the need for manual handling of materials.
Tooling advancements have further extended the nibbling machine’s capabilities. Hardened punches and dies made from high-performance tool steels or carbide materials enable the machines to work not only with mild steel but also with stainless steel, aluminum, brass, and other non-ferrous metals. Special punch geometries can even be designed for decorative perforations, dimpled surfaces, or ventilation patterns, adding both function and aesthetic appeal to the final product.
Nibbling machines are often found in facilities that require one-off parts or customized sheet metal components. Their ability to cut non-linear paths, irregular holes, and intricate interior shapes makes them indispensable for control panels, signage, switchboards, and various enclosures. Furthermore, the ability to start cutting from any point on the sheet without requiring an edge access allows for more material-efficient layouts, reducing waste and maximizing the use of raw material.
Even though newer cutting technologies like fiber lasers and waterjets offer faster speeds and smoother finishes, nibbling maintains a niche where its simplicity, flexibility, and relatively low operating cost provide a unique advantage. As part of a complete metalworking shop, a nibbling machine complements other tools by handling tasks that would otherwise require expensive or time-consuming alternatives.
Looking to the future, we can expect even smarter nibbling systems that incorporate AI-driven optimization for punch paths, real-time monitoring of tool wear, and automatic adjustment of stroke rates to match material conditions. These improvements will continue to enhance efficiency, extend tooling life, and allow for even more precise and efficient fabrication. The core principle of nibbling—a rapid sequence of small, deliberate cuts—remains as relevant as ever, proving its enduring value in the constantly evolving landscape of metalworking.
Another emerging trend in sheet metal nibbling machines is the integration of multi-tool turrets, which enable the system to switch between different punch shapes and sizes automatically within a single program cycle. This drastically reduces downtime between tool changes and allows for more complex part designs to be completed in one setup. For example, a single workpiece might require round holes, slots, and uniquely shaped cutouts; with a multi-tool system, the machine can execute all of these in sequence without operator intervention. This kind of flexibility is a significant productivity booster, especially in environments focused on lean manufacturing and just-in-time production.
Automation around the nibbling process is also expanding. Sheet feeding systems, automatic part ejection, and even robotic arms are now being used to move material in and out of the nibbling area. This not only speeds up the production cycle but also reduces human error and material handling risks. Combined with intelligent software, machines can track production runs, monitor punch wear, and schedule maintenance based on actual usage data, minimizing unexpected downtime and keeping production on schedule.
Energy efficiency is another area seeing steady improvements. While nibbling machines don’t consume as much power as thermal cutting methods, efforts are still being made to optimize motor performance, reduce idle energy use, and recycle mechanical energy from the punching action. In facilities aiming to meet sustainability targets, these efficiencies help lower the overall carbon footprint without sacrificing performance or precision.
In addition to functional parts, nibbling machines are being used creatively in architectural and design applications. Decorative facades, patterned screens, custom signage, and perforated panels with aesthetic appeal can all be produced through nibbling, especially when paired with advanced CAD tools that allow for the import of graphic patterns or artistic motifs. This opens up new markets for machine shops and fabricators, including interior design, urban architecture, and custom installations.
User interfaces on modern nibbling machines are becoming more intuitive as well. Touchscreen controls, real-time visualizations, and step-by-step programming assistants make it easier for operators of varying skill levels to use the equipment effectively. This democratization of access means even smaller shops or new technicians can produce complex components without extensive training, further broadening the machine’s utility.
Maintenance considerations have also evolved. Many newer models are designed with quick-access tool compartments, modular components, and self-diagnostic features that simplify upkeep. Alerts for punch wear or alignment issues help ensure consistent output quality and reduce the chance of part rejection due to unnoticed tool degradation.
In the competitive landscape of metal fabrication, the nibbling machine continues to hold its own by adapting to new demands with technological enhancements. It provides an unmatched combination of adaptability, precision, and ease of use—making it a valuable complement to laser cutters, plasma tables, and press brakes. As material diversity grows and custom metalwork becomes more prevalent across industries, the nibbling machine remains a trusted tool for turning digital designs into tangible, precision-cut components with speed and economy.
As digital fabrication becomes increasingly dominant, sheet metal nibbling machines are finding new ways to integrate with broader manufacturing ecosystems. For example, many modern systems are now connected to enterprise resource planning (ERP) and manufacturing execution systems (MES), enabling seamless communication between design, scheduling, inventory, and production. This means that once a part is designed in CAD and approved in the planning phase, the data can flow directly to the nibbling machine, which begins production without manual file transfer or setup. This level of connectivity ensures traceability, reduces lead time, and enhances overall workflow efficiency—especially in environments running multiple machines or managing a diverse set of customer orders.
The rise of additive manufacturing hasn’t diminished the role of nibbling—in fact, it’s made its utility even clearer. While 3D printing excels at creating complex parts from scratch, nibbling offers a faster and more economical solution for flat or semi-flat components that need precision cuts, slots, and internal shapes. In hybrid manufacturing setups, it’s common to see parts initially processed by nibbling and then further shaped or assembled using 3D-printed components or other fabrication methods. This synergy creates more options for designers and engineers, who can now combine traditional and emerging techniques for optimal results.
Portability and space efficiency are also being addressed in recent nibbling machine designs. Compact models with modular footprints are increasingly available, making them suitable for smaller shops, mobile workshops, or facilities with limited floor space. Despite their smaller size, these machines still deliver powerful performance, often including programmable settings and multi-material compatibility, proving that high functionality doesn’t always require a large machine.
There’s also a growing focus on operator ergonomics and user experience. Machines are being built with better lighting, noise insulation, and adjustable work surfaces to reduce fatigue and improve comfort during long shifts. Smart sensors track environmental conditions like temperature and vibration, and some even provide live feedback to the operator to suggest optimal cutting speeds or adjustments based on real-time data.
Furthermore, the global push for customization and personalization has increased demand for low-volume, high-variety production capabilities—something nibbling excels at. Whether it’s a one-off control panel for specialized industrial machinery or a limited run of artistic metal panels for a boutique building, nibbling provides the flexibility and control needed to execute the job quickly and with minimal setup time. Its ability to accommodate last-minute design changes without incurring massive tooling costs makes it a favorite in R&D departments, prototyping labs, and specialty manufacturing outfits.
Looking forward, innovations in tooling materials and smart punch technology may soon allow nibbling machines to self-calibrate for different materials or wear levels, pushing toward a future of fully autonomous operation. Combining this with advances in AI and machine learning could enable the machine to not only follow instructions but also recommend better tool paths, detect design inefficiencies, or predict failures before they occur.
The nibbling machine, in its essence, reflects a perfect balance of tradition and innovation. While it’s rooted in a mechanical process that dates back decades, its evolution has kept pace with the demands of the digital age. Whether in a high-tech automated facility or a flexible prototyping shop, the nibbling machine continues to prove its worth as a robust, adaptive, and essential tool in the diverse world of metal fabrication.
Sheet Metal Trimming Machine
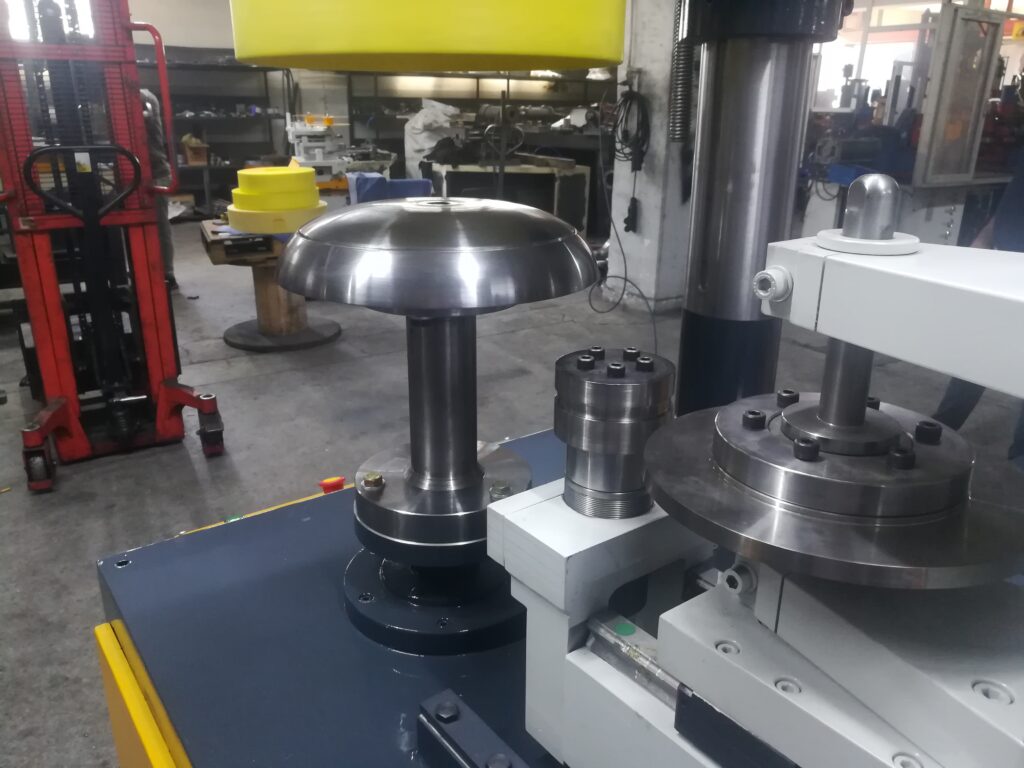
A Sheet Metal Trimming Machine is a specialized piece of equipment designed to remove excess material—commonly referred to as flash, burrs, or overhanging edges—from a metal workpiece after a primary forming or cutting operation. Trimming is an essential step in sheet metal manufacturing, especially for parts that have been deep drawn, stamped, or hydroformed, where excess material often remains along the perimeter or around holes and contours. The trimming process ensures clean, precise edges and prepares the component for final assembly, welding, or finishing operations.
These machines work using various mechanisms, including rotary blades, shearing dies, or press-driven cutters, depending on the type of material, the complexity of the trim line, and the volume of production. For simple, straight-edge trimming, guillotine-style trimmers or shear-based systems are common. For curved or complex contours, CNC-controlled rotary trimming or punch-and-die setups are preferred, providing greater flexibility and repeatability.
In high-production settings—such as the automotive or appliance industries—trimming machines are often integrated directly into forming lines. After a part is drawn or pressed into shape, it’s automatically transferred to the trimming station, where it is clamped and cut in a single cycle. This inline operation saves time, reduces handling, and ensures consistency from part to part. The precision of the trimming operation is critical, especially when the part must meet tight tolerances for fit, finish, or aerodynamic performance.
CNC trimming machines allow for programmable control over cut paths, enabling manufacturers to quickly switch between parts or adjust for material variations. This capability is particularly useful in facilities that produce multiple part types or work with a variety of alloys, as the operator can load a new design file and begin trimming with minimal retooling. Some machines even feature vision systems or laser sensors to automatically align the part before trimming, further improving accuracy and reducing scrap.
Rotary trimming machines, which use spinning blades to cut along the edge of a rotating part, are especially effective for circular or cylindrical workpieces such as automotive wheel wells, cylindrical containers, or turbine covers. These machines can produce a smooth, burr-free edge and often include adjustable guides or fixtures to accommodate different diameters or trim depths. The speed and cleanliness of rotary trimming make it a popular choice in industries where visual appearance and safety are paramount.
Tooling and die design play a crucial role in trimming operations. High-quality, wear-resistant tool steels or carbide inserts are used to maintain edge sharpness and dimensional accuracy over long production runs. Advanced coatings like TiN or DLC may be applied to reduce friction, extend tool life, and maintain edge quality when working with tougher metals like stainless steel or titanium.
Trimming machines also contribute to workplace safety and product quality. By automating a task that would otherwise require manual cutting, they minimize the risk of operator injury and ensure a more uniform result. Parts that are improperly trimmed can interfere with assembly, cause fitment issues, or even lead to structural failures—so consistent, accurate trimming is not just a cosmetic concern, but a critical factor in overall product integrity.
In recent years, trimming machines have benefited from the same technological advancements seen across the metal forming industry: smarter controls, tighter integration with digital design systems, and better energy efficiency. As manufacturers push for more sustainable and agile operations, trimming machines are being built with faster cycle times, reduced maintenance requirements, and easier operator interfaces.
Whether part of a massive industrial production line or a standalone unit in a job shop, sheet metal trimming machines are essential for finishing and refining parts to meet today’s demanding standards. Their combination of speed, accuracy, and adaptability ensures that every edge is clean, every fit is correct, and every product is ready for what comes next—be it paint, polish, assembly, or delivery.
In today’s fast-paced manufacturing environments, trimming machines are not just about cutting—they’re about control, consistency, and contributing to a lean production flow. Many of these machines are now being integrated with real-time monitoring systems that track tool wear, cycle times, and even part consistency. If a deviation is detected—such as an uneven trim or excess burr—alerts are sent to the operator or maintenance team, allowing immediate correction and preventing larger batches of faulty products. This kind of predictive and responsive capability is becoming increasingly important in sectors like aerospace, electronics, and medical devices, where part precision directly affects safety and performance.
Automation plays a growing role here as well. Robotic part loading and unloading has become common, especially for components with sharp edges or awkward geometries that might be hazardous or cumbersome for human operators. Once paired with conveyors or robotic arms, trimming machines can operate continuously with minimal human intervention, maintaining output while freeing workers for tasks that require greater decision-making or craftsmanship. In some high-volume facilities, trimming machines are networked together and coordinated by central control software, optimizing scheduling and tool usage across entire production lines.
Noise reduction and ergonomic enhancements are also making these machines more operator-friendly. Improved enclosure designs, acoustic damping materials, and smoother hydraulic or servo-electric actuation systems help reduce operational noise and vibration. This not only creates a more comfortable working environment but also helps protect machine longevity by minimizing mechanical stress. LED lighting, touchscreen controls, and programmable height adjustments are standard in many modern machines, supporting both productivity and worker well-being.
Sustainability is another area of innovation. Trimming machines are increasingly designed to minimize waste, with better chip collection systems and software that optimizes trim paths to reduce material removal. Scrap material can be automatically sorted and collected for recycling, turning what used to be a messy byproduct into a managed and reusable resource. Some machines even incorporate lubrication-free or dry-cutting technology, reducing the need for oils or coolants and lowering both environmental impact and cleanup costs.
The machines are also playing a crucial role in design flexibility. As more companies shift toward customizable or low-volume production, especially in consumer products and specialty equipment, trimming machines are able to keep up without sacrificing precision. Changing from one trim pattern to another can be as simple as loading a new digital file, making it possible to go from trimming refrigerator doors to complex, curved appliance casings or structural parts for electric vehicles in the same day.
Material science continues to push the limits of what these machines must handle. With the rise of high-strength steels, lightweight aluminum alloys, and composite-metal hybrids, trimming machines have had to evolve with more powerful drives, smarter feedback loops, and more resilient tooling. The ability to maintain clean, burr-free edges across these increasingly tough or delicate materials ensures that downstream processes—like welding, sealing, or cosmetic finishing—can proceed without additional rework or correction.
As these machines become smarter and more capable, their role is expanding from simple material removal to a critical phase in the manufacturing process that directly influences quality, efficiency, and product performance. The line between forming and finishing is blurring, and trimming machines are at the heart of that transition, acting not just as cutters, but as precision instruments within a tightly integrated production system. Their future lies in being even more responsive, connected, and adaptable—ready to meet the needs of both mass production and one-off custom work with equal skill and reliability.
One of the more subtle but powerful shifts happening with sheet metal trimming machines is their role in design feedback loops. As digital twins and simulation-based manufacturing become more prevalent, the data collected during trimming operations—such as force profiles, material springback, or trimming inaccuracies—is being fed back into design software. This enables engineers to tweak part geometry or forming processes upstream, long before any physical failure or inconsistency occurs. In essence, the trimming machine is not only a finisher of parts but also a sensor-rich node in a data-driven ecosystem that supports continuous design improvement.
This has a direct impact on quality assurance. Traditional quality checks often happen after trimming, using manual gauges or coordinate measuring machines (CMMs). But now, many trimming machines are being equipped with in-line inspection capabilities. Vision systems, laser scanners, or tactile probes built into the machine can verify edge dimensions and surface conditions instantly after the cut. This allows for automatic acceptance or rejection decisions on the spot, drastically reducing inspection time and removing bad parts from the workflow before they proceed to finishing or assembly.
The adaptability of modern trimming machines also means they can be used in more diverse applications than ever before. Beyond automotive panels and structural enclosures, they’re now being deployed for aircraft components, electronics chassis, energy equipment, and even custom art installations. Their ability to manage unique geometries and exotic materials—while still maintaining high-speed, consistent cuts—makes them ideal for industries where innovation, rapid iteration, and high standards go hand in hand.
Another factor contributing to their increased use is cost-effectiveness. Compared to full die-based trimming systems, which require expensive and time-consuming tooling for each part shape, modern programmable trimmers can switch from job to job with little more than a file change or a tool swap. This drastically lowers the barrier to entry for shops taking on small batch or high-mix work, and it reduces overhead for manufacturers who need to remain agile in a shifting market.
Add to that the shrinking physical footprint of many new designs, and trimming machines become even more attractive. Space-efficient models with integrated control cabinets, waste management, and modular mounting systems mean they can be easily fitted into crowded production lines or reconfigured when a factory layout changes. This flexibility is especially important for small to mid-sized enterprises competing with larger manufacturers, as it gives them a chance to implement leaner, smarter workflows without investing in overly complex infrastructure.
What ties all of this together is the evolution of the trimming machine into more than just a post-processing tool. It has become a platform for precision, intelligence, and integration—bridging the gap between raw formed material and the clean, defined edges required for modern product assemblies. In doing so, it contributes directly to product quality, production speed, and manufacturing intelligence. The seemingly simple task of edge cutting, when enhanced with today’s technology, becomes a critical differentiator in the quest for better products, faster delivery, and smarter factories.
Sheet Metal Notching Machine
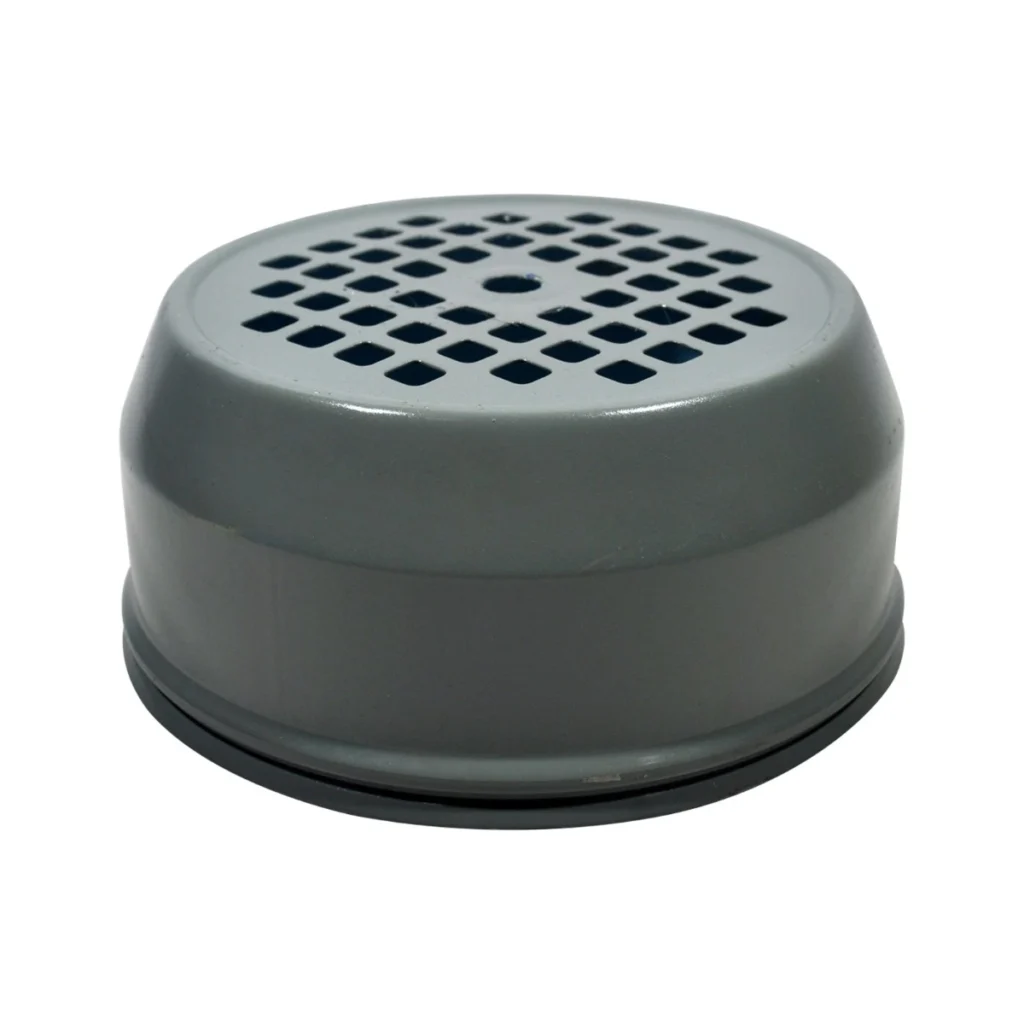
A Sheet Metal Notching Machine is a vital tool in metal fabrication, designed specifically to remove precise segments or “notches” from the edges or corners of a metal sheet. These notches make it easier to fold, join, or assemble sheet metal into more complex shapes like boxes, enclosures, and frames. Notching is often one of the first steps in forming processes where corners need to be removed to allow for clean, accurate bends or welded joints, and is especially common in the production of cabinets, ducts, electrical enclosures, and structural frameworks.
Notching machines come in a variety of forms, including manual, hydraulic, pneumatic, and CNC-operated models. Manual notching machines are typically operated with a foot pedal or lever and are ideal for low-volume jobs or simpler materials like aluminum and mild steel. Hydraulic and pneumatic models, on the other hand, provide greater power and speed for processing tougher materials or higher production volumes. CNC notching machines take this even further with programmable control, enabling precise and repeatable cuts on complex patterns or at multiple angles.
One of the most popular types is the corner notching machine, which removes a right-angled section from the sheet’s edge, typically at 90 degrees. These machines are used extensively for creating corners that fold together neatly, minimizing overlap and material stress. Adjustable angle notching machines go a step further by allowing users to modify the angle of the notch cut—anywhere from 30° to 140° in most cases—enabling the production of more complex geometries without changing tooling.
The cutting mechanism typically involves two high-strength steel blades, arranged in a V or L shape, which descend simultaneously to shear out the notch with a clean edge and minimal burr. These blades are designed to withstand thousands of cycles without losing sharpness, and they can be re-sharpened or replaced when needed. Modern machines also feature back gauges and stops that allow precise positioning of the workpiece, ensuring that each notch is placed exactly where it’s needed.
CNC-controlled notching machines enhance both precision and efficiency by allowing the operator to program multiple notching positions and patterns in a single setup. This eliminates manual measuring and repositioning, greatly increasing throughput and reducing the risk of human error. They also integrate easily with CAD/CAM systems, enabling direct use of digital part designs for programming, which is particularly useful for custom jobs and short-run production.
In high-volume applications, notching is often part of an automated line, where a sheet progresses from punching to notching, bending, and even welding without human handling. In these environments, notching machines are equipped with material handling systems and sensors to ensure alignment, part tracking, and cycle monitoring. This integration not only speeds up production but also improves consistency and reduces material waste.
Tooling is a critical part of the notching process, and advances in materials and coatings have made notching tools more durable and versatile. Hardened tool steel and carbide-tipped blades are common, capable of handling everything from galvanized steel to stainless alloys without premature wear. Some machines also support interchangeable tooling heads, allowing a single unit to perform a range of notch shapes, including rectangular, V-shaped, or even custom profiles.
Safety is also a major consideration. Modern notching machines include features such as light curtains, two-hand safety controls, emergency stop buttons, and automatic blade retraction to protect the operator. Additionally, clear guarding and ergonomic work surfaces improve operator visibility and comfort, which contributes to both safety and productivity.
From a cost-efficiency standpoint, notching machines are relatively low maintenance and high impact. They require minimal consumables, have compact footprints, and deliver immediate results with minimal training. For fabrication shops working with architectural panels, HVAC components, or custom metal parts, the notching machine offers a fast and reliable way to prepare sheet metal for bending and assembly.
As fabrication becomes more digitized, notching machines are evolving in parallel—offering smarter software, IoT integration for machine monitoring, and even adaptive cutting that compensates for material thickness variations. In essence, the sheet metal notching machine continues to serve as a precise, efficient, and indispensable asset in the metalworking industry, playing a quiet but crucial role in shaping the clean corners and joints that hold modern structures and products together.
In addition to the standard corner and edge notching, there are more specialized variations of sheet metal notching machines that allow for intricate shapes and profiles. Some of these advanced models are capable of creating slots, holes, and custom patterns within the metal sheet, broadening the machine’s application in industries like automotive, electronics, and appliance manufacturing. The ability to handle a variety of materials, including non-ferrous metals like aluminum and copper, as well as tougher alloys, makes the notching machine incredibly versatile for different sectors.
CNC notching machines, in particular, offer a level of flexibility that is unmatched by manual or hydraulic systems. With these machines, users can input notching designs directly from CAD software, allowing for quick changes in part design, more complex cutting patterns, and greater precision. The precision of CNC control also reduces the likelihood of defects like improper notches, which could affect assembly and overall product strength. Furthermore, because these machines are often equipped with advanced sensors, they can detect material thickness variations and automatically adjust the cutting parameters, ensuring consistent results.
In the manufacturing of metal enclosures, a notching machine ensures the proper fit of metal panels and frames that need to be joined together by welding, riveting, or fastening. Without proper notching, the metal sheets may not align correctly during assembly, leading to a misaligned product or the need for additional manual adjustments. For example, in HVAC systems, notched metal components fit together tightly, reducing the amount of material wasted and improving both the performance and aesthetics of the final product.
Another benefit of the notching machine is its ability to perform high-speed operations, especially with advanced hydraulic or pneumatic presses. This makes them an ideal tool for high-volume production environments, where numerous parts must be processed quickly and consistently. For shops focusing on large orders or batch production, a notching machine with a quick-change tooling system can help reduce downtime and improve efficiency, as it enables faster tool swaps between different notching shapes and sizes.
The increasing automation of sheet metal notching machines also plays a key role in reducing labor costs and human error. With automated loading and unloading systems, as well as integrated production lines, the need for manual labor is minimized, allowing operators to focus on higher-level tasks such as programming, machine maintenance, and quality control. Additionally, by incorporating real-time machine monitoring systems, operators can track performance, detect malfunctions early, and maintain steady production rates.
Beyond traditional applications, sheet metal notching machines are increasingly being employed for custom metalwork—whether it’s for architectural designs, furniture, or art installations. Their ability to execute precise, intricate cuts in various sheet sizes and shapes makes them invaluable for industries requiring unique, one-of-a-kind pieces. This trend is amplified by the growing demand for personalized or bespoke products that cater to niche markets.
Energy efficiency is another important area where manufacturers are pushing for improvements in notching technology. With increasing pressure to reduce carbon footprints, modern machines are designed to operate more efficiently. Enhanced hydraulic systems or servo-electric drives, for example, allow for energy savings during the cutting process. Moreover, improvements in tool durability and precision lead to reduced material waste, further promoting sustainability.
As with many other metalworking tools, advancements in digitalization and connected manufacturing are transforming sheet metal notching. Machine learning algorithms can now predict tool wear based on usage patterns, adjusting operational settings in real time to minimize downtime and prevent errors. These systems can also collect valuable data that can be analyzed to optimize the entire production line, giving manufacturers insights into production bottlenecks, tool performance, and maintenance schedules.
The future of notching machines is also expected to include increased integration with augmented reality (AR) and virtual reality (VR). These technologies will likely allow operators to visualize parts and processes in a three-dimensional space before executing a cut, providing further confidence in the accuracy of their notching operations. By viewing a digital representation of the finished product, operators can preemptively address potential design flaws and make adjustments as necessary before any physical cutting takes place.
In conclusion, the sheet metal notching machine continues to be an essential and highly adaptable tool in modern metal fabrication. As industries push for greater precision, efficiency, and customization, these machines will keep evolving with advancements in automation, digital integration, and material science, helping to shape the next generation of high-quality metal products with speed and precision. Their versatility and precision ensure that they remain a cornerstone of sheet metal processing, whether for mass production or one-off custom designs.
As the demands on the manufacturing sector continue to evolve, sheet metal notching machines are becoming increasingly sophisticated, with features that go beyond simply cutting material. In particular, smart technology is having a significant impact on how these machines are utilized. IoT (Internet of Things) connectivity allows machines to communicate with other equipment in the production line, providing real-time data on performance, material usage, and even part quality. This not only enhances production efficiency but also allows operators and managers to make informed decisions about process adjustments, maintenance schedules, and resource allocation.
With the integration of predictive maintenance tools, operators can monitor the health of the machine through sensors and data analytics. These tools can predict when components such as blades, hydraulic pumps, or even electronic systems will need maintenance or replacement, preventing unexpected downtime and costly repairs. By continuously analyzing machine performance, the system can alert technicians before an issue arises, ensuring that production remains uninterrupted and quality standards are met consistently.
Another growing trend is the shift towards multi-functional notching machines, which can perform notching, punching, and even small hole punching in a single machine. These versatile machines eliminate the need for separate operations and streamline the overall production process, saving time and reducing the amount of equipment required in a shop. For manufacturers that work with a range of different part types or materials, having a single machine that can handle multiple processes adds a significant amount of flexibility without sacrificing precision.
Automation continues to play a key role in the evolution of notching machines, with automated loading and unloading systems becoming standard in many high-volume environments. Automated systems allow parts to be loaded into the machine, notched, and then unloaded or moved to the next stage of production with minimal human intervention. These systems are particularly valuable in environments that require a high level of consistency and speed, as they ensure that each part is handled in the same way, reducing the chance of errors.
Moreover, advancements in software development are also contributing to the efficiency of notching machines. Many modern machines come equipped with software that helps operators quickly set up the machine, adjust parameters, and simulate the cutting process to ensure that the notching will be performed as expected. This software can also interface with other parts of the production line, improving overall coordination and reducing the potential for costly mistakes. By allowing for the optimization of the cut paths and automatically adjusting the process to match different material types, thicknesses, or configurations, software helps to ensure high-quality cuts and minimal material waste.
The increasing demand for customized parts in industries such as aerospace, automotive, and electronics has prompted innovations in notching technology to allow for greater design flexibility. As product designs become more intricate and unique, notching machines are increasingly being equipped with features that allow them to handle complex geometries and non-standard shapes. These machines offer the ability to not only notch in traditional straight lines but also along curves, angled cuts, and custom patterns, enabling manufacturers to meet the requirements of specific, high-precision applications.
Another exciting development is the integration of artificial intelligence (AI) and machine learning algorithms into notching processes. These technologies can be used to optimize the cutting strategy, improving the speed of operation while maintaining quality. For instance, AI can analyze past cutting data to learn the best parameters for different types of material or geometry, adjusting the process automatically to achieve the best results. AI-driven systems can also detect potential defects or inconsistencies in real-time, ensuring that all parts meet strict tolerances without requiring additional human oversight.
At the same time, sustainability concerns are increasingly shaping the design and operation of notching machines. Newer machines are being developed to be more energy-efficient, consuming less power while still delivering high-performance cutting. By optimizing the cutting speed and energy use, manufacturers can not only reduce operating costs but also contribute to a greener manufacturing process. Additionally, waste reduction technologies—such as improved chip collection and recycling systems—are helping manufacturers better manage scrap material, turning what was once waste into a valuable resource that can be reused or sold.
As industries become more globalized and the demand for rapid, high-quality production increases, the ability to produce large batches of precision parts quickly becomes a competitive advantage. Sheet metal notching machines, with their growing automation, integration, and smart technologies, are poised to play an even larger role in meeting these needs. Whether it’s through faster cycle times, more precise cuts, or the ability to handle diverse materials, the next generation of notching machines will continue to be a critical part of the sheet metal fabrication process.
Ultimately, the evolution of sheet metal notching machines reflects a broader shift in manufacturing towards greater efficiency, flexibility, and intelligence. By combining the latest technological innovations—such as AI, IoT, and automation—with traditional mechanical processes, these machines are shaping the future of metalworking, allowing manufacturers to create higher-quality products at lower costs, with greater speed and precision. The role of notching in metal fabrication is only growing, with no sign of slowing down as industries continue to demand greater customization, shorter lead times, and better performance.
As industries continue to evolve and demand for high-precision, high-quality metal components increases, the role of sheet metal notching machines will only become more critical in the production process. The integration of advanced control systems into these machines allows for even greater adaptability. For example, machines now often feature touchscreen interfaces, making them easier to operate, with intuitive controls that allow operators to quickly adjust settings, select tooling, and optimize cycle times. This not only streamlines operations but also reduces the likelihood of human error, improving overall quality and consistency in production.
The growing emphasis on lean manufacturing is driving further innovation in notching technology. By minimizing waste, reducing production time, and improving the use of available materials, lean principles align perfectly with the capabilities of modern notching machines. The ability to nest parts within a sheet metal layout—maximizing material usage and minimizing scrap—has become a key function in many automated systems. Software advancements in nesting algorithms allow operators to efficiently plan out notching and cutting patterns, optimizing material utilization and improving cost-efficiency. This is especially important in industries like aerospace or automotive, where material costs are high, and waste reduction can have significant financial and environmental benefits.
Additionally, the introduction of augmented reality (AR) in machine setups and operation is a trend that is making its way into notching machines. AR systems can assist operators by superimposing digital instructions or part diagrams onto the physical workspace, making it easier to align materials or adjust machine settings in real-time. This digital overlay can be particularly helpful in environments where precision is paramount, and machine operators may need to quickly change between complex part setups or troubleshoot issues during production. In highly dynamic production environments, AR could drastically reduce setup times and help operators stay on top of machine performance, leading to greater overall efficiency.
Another notable advancement is the increased capability of multi-material processing. While traditional notching machines were primarily designed for use with sheet metal, newer machines can accommodate a wide range of materials, from plastics and composites to more advanced alloys and metals with varying thicknesses. These machines are equipped with specialized tooling and cutting mechanisms that can handle the different properties of each material while still delivering clean, precise notches. This is particularly valuable for industries that require the use of multiple materials in a single product, such as the automotive and electronics industries, where lighter, stronger, or more heat-resistant materials are often integrated into a single design.
With the increasing sophistication of robotic integration, sheet metal notching machines are also becoming part of larger, more automated workflows. Robots are now frequently used in conjunction with notching machines to load and unload parts, further reducing labor costs and enhancing efficiency. In many setups, the machine is connected to a network of robotic arms that can automatically move parts between different stations in the production line. This level of automation is particularly beneficial in high-volume environments, where the speed of production and consistency are critical. It also helps reduce operator fatigue and ensures that work can continue around the clock without interruption, contributing to a 24/7 manufacturing capability in some facilities.
Furthermore, advancements in material handling systems are improving the way materials are processed before and after being notched. Conveyor belts, robotic arms, and even drones are being used to transport sheet metal parts through the factory efficiently. These systems ensure that parts are properly aligned before being fed into the notching machine, and they can also handle parts after notching, moving them seamlessly to the next step in the production process. The automation of material handling reduces the risk of errors, such as misalignment or dropped parts, which can lead to defects or delays.
The data-driven manufacturing trend also plays a significant role in enhancing the capabilities of notching machines. By collecting and analyzing data from machines, manufacturers can fine-tune the operation of notching machines to maximize uptime, improve cutting speeds, and optimize the quality of the finished parts. These systems can track key performance indicators (KPIs) such as machine utilization rates, downtime, energy consumption, and output quality. Machine learning algorithms are increasingly being used to process this data and recommend process adjustments, which can lead to more efficient operations and higher product quality.
The demand for high-precision, low-tolerance metal parts is increasing, especially in industries like medical devices, electronics, and defense. As these industries require ever more complex parts, sheet metal notching machines are evolving to meet these challenges. With the increased use of laser-assisted notching or water jet cutting, some machines can produce extremely fine, detailed cuts with minimal material deformation. These processes are ideal for parts that require precision, especially those with complex, intricate patterns or small cuts that would otherwise be difficult to achieve with traditional mechanical notching.
Finally, customization continues to drive growth in the use of sheet metal notching machines. The ability to adjust parameters like notch depth, angle, and shape allows manufacturers to respond quickly to customer requests for custom parts or modifications. In fact, some companies are now specializing in short-run, custom sheet metal parts, with notching machines that are capable of rapidly adapting to different designs, without the need for extensive tooling changes or setup times. This trend is especially prevalent in small-batch manufacturing or just-in-time production systems, where flexibility and speed are key factors.
In conclusion, sheet metal notching machines are far more than just cutting tools—they are highly adaptable, intelligent pieces of equipment at the heart of modern manufacturing systems. As technology continues to advance, these machines will become even more integral to industries ranging from aerospace and automotive to electronics and medical devices. Their ability to process complex shapes, handle diverse materials, integrate with automation systems, and provide real-time feedback will continue to drive efficiency and quality in metal fabrication, enabling manufacturers to meet the growing demand for precision-engineered products in a competitive global market.
Sheet Metal Shearing Machine
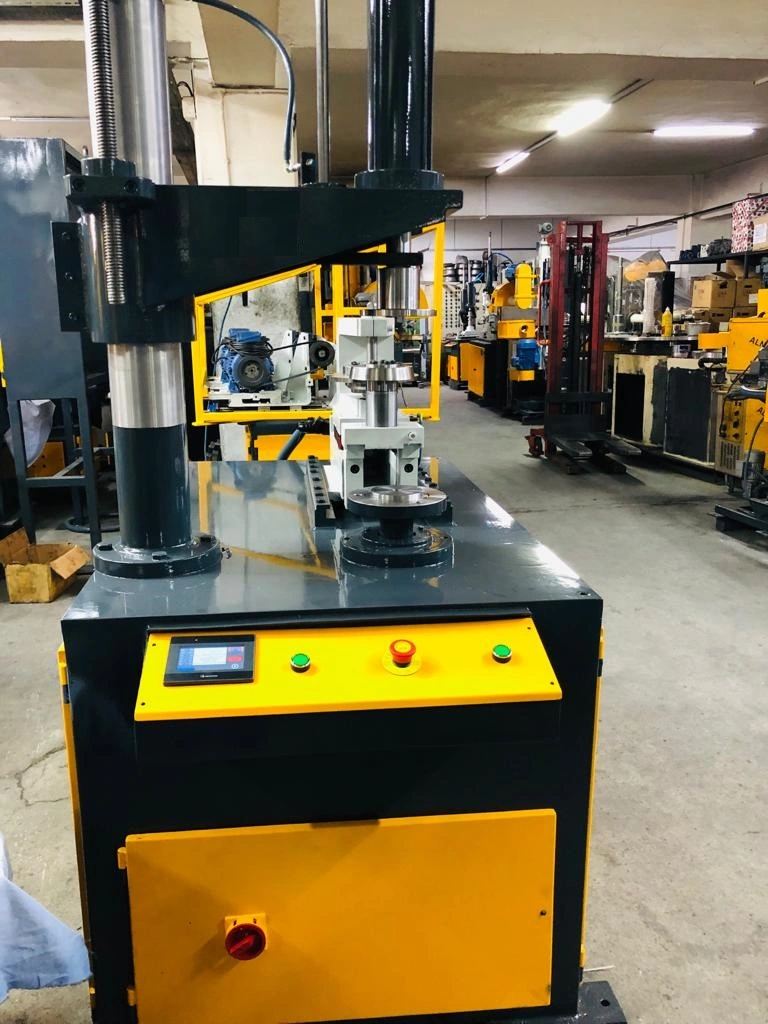
A Sheet Metal Shearing Machine is an essential tool in the metal fabrication industry, designed to cut sheet metal into specific shapes and sizes without the use of a die. This machine operates on the principle of applying a shear force to the material, causing it to fracture and form a clean edge. Shearing machines are highly versatile, capable of cutting a wide range of materials, including steel, aluminum, copper, and other metals, making them indispensable in a variety of industries like automotive, construction, and appliance manufacturing.
The operation of a sheet metal shearing machine typically involves two major components: the upper blade and the lower blade. These blades are positioned with a slight gap between them, and as the upper blade descends, it applies a shearing force to the sheet metal. This force causes the metal to break cleanly along the cutting line, with minimal deformation to the edges. The process is similar to cutting paper with scissors, but with much higher force and precision to accommodate the hardness and thickness of sheet metal.
Shearing machines can be classified into different types based on their mechanism of operation:
- Mechanical Shearing Machines: These machines use a flywheel and motor-driven system to apply the shearing force. They are typically used for cutting thinner sheet metal and are often seen in smaller workshops or factories with lower production volumes.
- Hydraulic Shearing Machines: These are more powerful and commonly used for cutting thicker materials. They use hydraulic pressure to control the movement of the upper blade, providing more precise control over cutting force and speed. Hydraulic shears are popular for industrial applications where large volumes of sheet metal need to be processed quickly and efficiently.
- Pneumatic Shearing Machines: Similar to hydraulic machines but using air pressure instead of hydraulic fluid to control the blade’s movement. Pneumatic shears are often used for lighter, quicker cutting jobs and are ideal for applications requiring rapid production cycles.
- CNC Shearing Machines: Computer Numerical Control (CNC) shearing machines are equipped with advanced controls that allow for precise programming of cutting parameters. Operators can input specific measurements, cutting angles, and blade movements directly from CAD software, allowing for highly accurate and repeatable cuts. CNC shears are ideal for high-precision manufacturing, where complex shapes or multiple cuts are required.
One of the significant advantages of using a shearing machine is the speed at which it can cut metal. Shearing machines can process large sheets of metal quickly, making them an ideal choice for batch production or when high-volume cuts are required. These machines also provide excellent edge quality, with minimal burrs or deformation, especially when compared to other cutting methods like punching or laser cutting.
Shearing machines are also versatile in terms of the thickness of material they can handle. Hydraulic shearing machines, for instance, can accommodate thicker materials, while mechanical or pneumatic shears are better suited for thinner gauge materials. The cutting capacity of a shearing machine is typically determined by its blade length and the force it can apply, with larger machines capable of handling sheets of greater width and thickness.
Many modern shearing machines come equipped with back gauges that help position the material accurately before cutting. These back gauges are adjustable, allowing for precise control over the length of the cut and ensuring that each part is consistent in size. Digital readouts and automated control systems are also becoming common, enabling operators to input precise measurements and cut multiple parts with minimal setup time.
One of the critical challenges in shearing is material deformation, which can occur if the shearing force is not properly adjusted for the thickness or hardness of the metal. To prevent this, advanced shearing machines often feature adjustable blade gaps and cutting angles, allowing operators to fine-tune the settings for each specific material. Shear force distribution is also important, as improper force can lead to unwanted warping or bending of the material, compromising the quality of the cut.
Safety is a major consideration in the design of sheet metal shearing machines. Modern shearing machines are equipped with various safety features, such as two-hand operation controls, light curtains, and emergency stop buttons. These features ensure that the operator’s hands or body are kept at a safe distance from the moving blades, minimizing the risk of injury. Additionally, proper machine guarding and safety protocols are essential in maintaining a safe work environment when using these machines.
In terms of maintenance, shearing machines require regular care to ensure they remain operational and produce high-quality cuts. Routine checks of the blades, hydraulic system (in hydraulic machines), and the overall alignment of the machine are necessary to avoid downtime and maintain cutting precision. Blade sharpening and replacing worn-out blades are key maintenance tasks that help extend the life of the shearing machine and maintain performance.
Advances in automation are also revolutionizing sheet metal shearing. Automated systems allow for the continuous feeding of material into the machine, with minimal human intervention. This automation is particularly valuable in high-volume manufacturing settings, where it can greatly reduce labor costs and improve overall production efficiency. Furthermore, robotic arms and conveyors are often integrated into shearing systems, enabling materials to be automatically loaded, cut, and unloaded, further enhancing the speed and consistency of the process.
In addition to traditional sheet metal applications, modern shearing machines are being used in the solar panel and wind turbine industries, where the demand for clean, precise cuts on large sheets of metal is growing. These machines are also being adapted for cutting other materials such as plastics, composite materials, and even advanced alloys like titanium, expanding their versatility and reach in various markets.
The evolution of shearing machines is not limited to improving their cutting capabilities. Energy efficiency has also become a priority for manufacturers. Newer models are designed to reduce energy consumption while maintaining cutting speed and force, addressing both cost and environmental concerns. This is particularly important in large-scale manufacturing plants where energy use can be a significant portion of operational expenses.
In conclusion, sheet metal shearing machines remain a cornerstone of modern metal fabrication. With ongoing advancements in automation, precision, and versatility, they are becoming more efficient, safer, and better equipped to handle the increasingly complex demands of today’s manufacturing industries. Whether for high-volume production or precise, custom cuts, shearing machines offer a fast, effective, and reliable way to shape sheet metal into the parts needed for everything from structural components to consumer products. As industries continue to evolve, so too will the technology behind sheet metal shearing, offering even more advanced features that will push the boundaries of what is possible in metalworking.
Sheet metal shearing machines continue to evolve, driven by the increasing demand for precision, speed, and efficiency in the manufacturing sector. These machines are not only integral to industries requiring bulk metal cutting but are also gaining attention in more specialized fields. For example, the automotive industry relies on shearing machines to produce components for vehicles, where tight tolerances and the ability to cut through a variety of materials are essential. The aerospace sector similarly benefits from shearing machines that can handle tougher alloys, creating parts that must meet rigorous safety and performance standards.
As these industries continue to advance, so does the technology that drives sheet metal shearing. Manufacturers are increasingly integrating advanced sensor technology into shearing machines, enabling the machine to automatically adjust settings based on real-time data. This means the machine can monitor things like material thickness, hardness, and even temperature fluctuations to optimize cutting force and ensure the highest quality cuts, regardless of the material being processed. These sensors help prevent issues like uneven cutting, material deformation, or excessive wear on the blades.
The digitalization of sheet metal processing also plays a significant role in the modernization of shearing machines. In addition to CNC control, which allows operators to input and program specific cutting parameters, many shearing machines now incorporate IoT-enabled systems. These systems allow for remote monitoring and diagnostics, where operators can track the performance of the machine from a distance and receive notifications about potential issues before they become problems. This remote connectivity also aids in predictive maintenance, where machine components are monitored for signs of wear or failure, reducing downtime and improving the longevity of the equipment.
With the rise of Industry 4.0, sheet metal shearing machines are becoming smarter and more connected to larger production systems. Through integrated software, shearing machines can now communicate with other parts of the production line, helping streamline workflows and increase overall efficiency. For instance, once a sheet metal part is sheared, it can be immediately sent to the next stage of production, whether it’s further processing, assembly, or packaging, all while keeping track of production rates and material usage. This creates a more cohesive and automated workflow, which is essential for high-demand production environments.
In addition, the design and materials used in shearing machines have advanced significantly. Manufacturers are opting for high-performance alloys and coatings for the blades and critical components to increase durability, reduce friction, and prolong the lifespan of the machine. These innovations are essential in high-volume operations, where machines may run continuously for long hours. The development of self-lubricating materials has also reduced the need for frequent maintenance, helping to lower operational costs and improve machine reliability.
One of the emerging trends is the growing emphasis on energy efficiency. As global sustainability standards continue to rise, there is increasing pressure on manufacturers to minimize their environmental impact. New shearing machines are being designed with more energy-efficient motors, hydraulic systems, and controls that reduce power consumption without sacrificing performance. Some machines now feature energy recovery systems, which capture and reuse energy from the machine’s movements, further reducing energy use and operating costs. These innovations not only contribute to sustainability goals but also appeal to manufacturers looking to lower their operational overhead.
The integration of artificial intelligence (AI) is also making waves in the shearing machine industry. By analyzing historical cutting data, AI algorithms can recommend adjustments to cutting parameters in real time, helping to optimize cutting speeds, material usage, and edge quality. Over time, the machine learns from each cut, improving its efficiency and minimizing waste. This ability to self-optimize and adapt to changing conditions is particularly valuable in custom manufacturing settings, where no two parts are exactly the same, and precise adjustments are often required.
Another critical aspect of the shearing machine’s evolution is its ability to handle more complex materials. While traditional shearing machines were primarily designed for sheet metal, modern machines can handle a range of advanced materials, including composite materials and high-strength steels. These materials require different cutting techniques and more advanced equipment to handle their unique properties. For instance, composite materials may require a slower cutting speed to prevent cracking, while high-strength steels demand higher shear forces and specialized tooling to ensure clean cuts without excessive tool wear. Shearing machines that can adapt to these materials open up new possibilities for industries like renewable energy, where cutting parts for wind turbines and solar panels requires the use of advanced materials.
With the demand for more customized production and shorter lead times, sheet metal shearing machines are increasingly being used in small batch production environments. Traditionally, shearing was associated with high-volume, mass production. However, with advancements in CNC and robotic integration, shearing machines can now be quickly reprogrammed to produce small quantities of customized parts. This flexibility is especially valuable in industries like electronics, where rapid prototyping and small-batch production runs are common. In these environments, being able to quickly change cutting parameters or switch between different material types without extensive downtime is a significant advantage.
Automation continues to play a critical role in the growth of sheet metal shearing technology. Fully automated systems that include material handling, cutting, and post-processing are becoming increasingly popular in high-output manufacturing. These systems can operate around the clock with minimal human intervention, ensuring consistent output and reducing the likelihood of human error. Automation not only speeds up production but also improves the overall safety of the operation by minimizing the need for workers to interact directly with the machine while it is running.
In industries where precision is key, such as medical device manufacturing or aerospace, shearing machines have become critical tools for producing components with tighter tolerances. These industries demand that every part meet exact specifications, and modern shearing machines equipped with advanced controls can deliver the precision required for these high-stakes applications.
Finally, there is an increasing trend toward integrating shearing machines into larger digital ecosystems. As manufacturing moves towards digital twins, smart factories, and real-time data integration, shearing machines are becoming more than just standalone pieces of equipment. They are being integrated into broader systems that include quality control, production scheduling, and even supply chain management. This digital integration allows manufacturers to have a real-time overview of their production processes, optimize operations, and ensure that each part produced meets the highest standards.
As the demand for high-quality, cost-effective, and customized sheet metal parts continues to rise, sheet metal shearing machines will remain at the forefront of manufacturing innovation. With ongoing advancements in automation, digitalization, and material processing, these machines will not only enhance productivity but also offer greater flexibility, precision, and efficiency. Whether in high-volume or custom manufacturing environments, shearing machines will continue to play a vital role in shaping the future of metalworking.
The continued evolution of sheet metal shearing machines will likely focus on expanding their capabilities and improving their integration into smart factories. The advent of smart manufacturing technologies such as the Internet of Things (IoT), artificial intelligence (AI), and machine learning will enable shearing machines to become even more intelligent and adaptable. Through IoT connectivity, machines can transmit data in real time, allowing operators to monitor performance, adjust settings, and troubleshoot remotely. This will significantly improve downtime management and reduce the need for on-site maintenance by predicting failures before they occur.
Machine learning will play a pivotal role in improving the efficiency and accuracy of sheet metal shearing. Over time, AI algorithms can learn from past cutting patterns, material properties, and production data to automatically optimize settings for new jobs. The system can adjust cutting speeds, pressure, and blade gaps based on these inputs to reduce waste, improve cutting quality, and speed up production cycles. The result is a more autonomous machine that requires less human intervention and can produce parts with greater consistency, even in the face of varying material types or production conditions.
Additionally, the ongoing focus on sustainability will likely drive more energy-efficient innovations in shearing machines. Manufacturers are increasingly aware of the need to reduce their carbon footprint, and machines designed with energy efficiency in mind will help meet these goals. Features like variable-speed drives that adjust motor speed according to demand, regenerative braking systems, and energy-efficient hydraulic systems are expected to become more commonplace. These advancements help to minimize energy consumption during the machine’s operation, reducing both costs and environmental impact.
The use of advanced materials in shearing machines is another area of significant progress. Manufacturers are now experimenting with cutting-edge materials, such as ceramic-coated blades or high-performance alloys, to improve blade longevity and reduce wear and tear during heavy use. The development of self-healing materials in the blade construction could be a groundbreaking innovation, extending the life of shearing machines and reducing downtime for maintenance.
As industries place increasing demands on manufacturers to reduce lead times and offer customized products, shearing machines are adapting to these needs. With real-time programmable settings, operators can adjust the machine quickly to accommodate various sheet thicknesses, materials, and cutting patterns without extensive retooling or delays. The ability to cut multiple parts with different shapes or sizes from a single sheet of metal, a process known as nesting, is becoming more sophisticated with the use of software that automatically arranges parts for optimal material utilization.
The integration of robots into the shearing process is another major trend. Robotic arms can load and unload materials, position the metal accurately, and remove cut parts from the machine, reducing manual handling and increasing throughput. This combination of robotics and shearing technology helps create highly automated and flexible manufacturing environments capable of processing different metal parts continuously, without the need for human intervention.
In addition, automation and robotics make it possible to adapt shearing machines for a wider range of custom and low-volume production scenarios. Historically, shearing machines were best suited for high-volume, repetitive production. However, with the integration of robotics and software-driven systems, they are increasingly being used in industries that require flexibility, like custom fabrication and small-batch production. These machines can now quickly switch between different materials, sizes, and cut patterns, catering to industries such as medical device manufacturing, electronics, and consumer goods, where shorter production runs and specialized components are common.
As manufacturers aim to improve part quality, the focus is shifting to reducing burrs, deformation, and cutting edge roughness. Innovations in blade design, as well as more precise control over cutting force and speed, will help mitigate these issues. With better blade materials, coatings, and sharper edges, shearing machines will be able to provide parts with cleaner edges, reducing the need for post-processing operations such as deburring or polishing. Furthermore, the enhanced cutting precision will result in more accurate dimensions, especially when dealing with complex, high-tolerance parts that are required in industries like aerospace or defense.
In terms of user experience, there’s a growing trend to simplify machine operation through more intuitive interfaces and smart controls. The integration of augmented reality (AR) and virtual reality (VR) into shearing machines could potentially enable operators to visualize cutting parameters, material positioning, and tool wear in real time. AR and VR technologies could help train new operators faster, offering a more interactive and immersive training experience while also providing real-time assistance during operation.
Maintenance will also benefit from advances in predictive analytics. Modern shearing machines are increasingly equipped with sensors that monitor various parameters, such as motor performance, hydraulic pressure, and blade wear. These sensors feed data into a central system that analyzes trends and predicts when maintenance is needed, allowing for just-in-time servicing and reducing unplanned downtime. Predictive maintenance can significantly lower costs, prevent production interruptions, and improve overall machine lifespan.
As the global manufacturing landscape continues to evolve, the demand for faster, more precise, and energy-efficient sheet metal cutting solutions will continue to grow. Advanced shearing machines, with their intelligent capabilities, will play a vital role in meeting these needs. With enhanced automation, better material handling, and cutting-edge technology, these machines will not only improve manufacturing productivity but also enable the creation of high-quality, custom metal parts faster and more efficiently than ever before.
Ultimately, sheet metal shearing machines will continue to be a cornerstone of modern metalworking. As they evolve, their ability to adapt to a wide range of materials, products, and production needs will make them even more indispensable in shaping the future of manufacturing. With each innovation, the industry moves closer to a future where automation, sustainability, and precision are the key drivers of growth and efficiency in sheet metal fabrication.
Sheet Metal Curling Machine
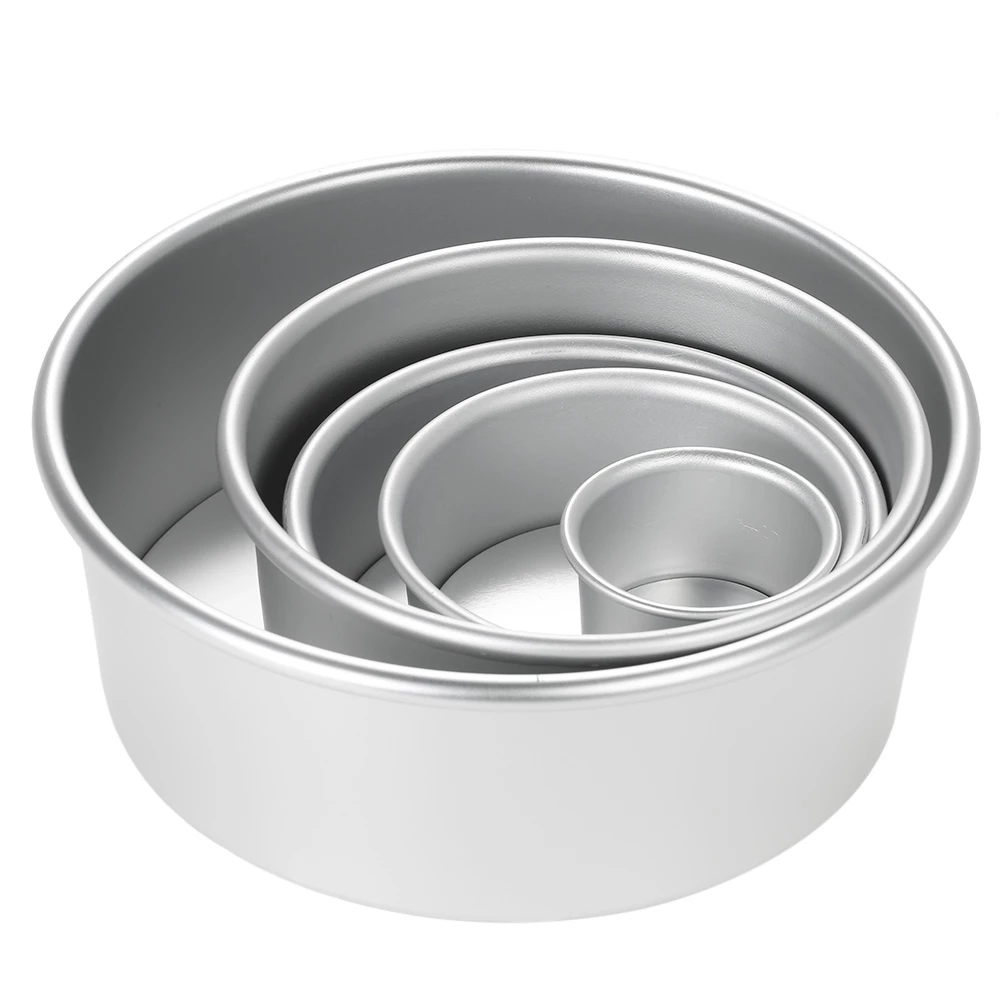
A Sheet Metal Curling Machine is used in the metalworking industry to create a smooth, rolled edge or curl on the perimeter of a sheet metal part. This process is commonly used to form edges on metal sheets for a variety of applications, including creating closed edges for cosmetic purposes, reinforcing edges to increase strength, or preparing sheets for further processing. The machine works by bending or curling the metal sheet around a set of rollers or tools to form a circular or cylindrical shape at the edges of the material.
The curling machine typically consists of several key components:
- Rollers or Dies: These are the primary tools that shape the edges of the sheet metal. The rollers are positioned to create the desired radius or curl at the edges of the sheet as it passes through the machine.
- Material Feed Mechanism: This system ensures the smooth feeding of sheet metal into the curling machine, ensuring consistent performance and quality. The material can be fed manually or automatically, depending on the machine’s design.
- Adjustable Pressure and Speed Settings: To ensure precision, most curling machines have adjustable settings that allow operators to control the pressure and speed at which the sheet metal moves through the rollers. This helps in achieving the desired curl radius and thickness of the material.
- Drive Mechanism: This includes motors and gears that power the rollers and ensure the consistent movement of the sheet metal through the machine. The drive system must be robust enough to handle various thicknesses of sheet metal and maintain control over the curling process.
- Control Panel: The control panel allows operators to set specific parameters for the curling process, such as the curl radius, material feed rate, and machine speed. Modern curling machines often feature CNC controls, enabling operators to program and fine-tune settings for different materials and part requirements.
Types of Sheet Metal Curling Machines
- Manual Curling Machines: These are simpler machines that require the operator to manually feed the sheet metal through the rollers. They are typically used for small-scale production or where only a small amount of curling is needed.
- Hydraulic Curling Machines: Hydraulic systems are used to apply pressure more precisely and are often found in larger machines for industrial use. These machines are well-suited for thicker materials or larger production runs, as the hydraulic system provides more control over the curling force.
- CNC Curling Machines: These advanced machines are equipped with computer controls that allow for precise programming of curl sizes, angles, and speeds. They can be used for complex or high-precision applications where exact curl dimensions are required.
- Roll Forming Curling Machines: These machines are used for more continuous curling processes, where large coils of sheet metal are processed into products that require a consistent edge curl along the length of the material. Roll forming machines are ideal for high-volume production.
Applications of Sheet Metal Curling Machines
- Edge Reinforcement: One of the most common applications is to curl the edges of sheet metal parts to provide additional strength. This is particularly important in applications where the edges are subject to mechanical stress, such as in automotive parts or appliance components.
- Cosmetic Purposes: In some industries, sheet metal parts need to have curled edges for aesthetic reasons. For example, consumer products, decorative panels, and architectural components often use curled edges for a polished, finished appearance.
- Joining and Sealing: Curling machines can also be used to prepare edges for joining or sealing. For example, curled edges can be used to create a strong, interlocking joint between parts, making them suitable for use in containers, ducts, or tubing.
- Preparation for Further Processing: In some cases, the curling process is performed to prepare parts for further processes like welding, bending, or forming. The curled edge may facilitate easier handling or alignment of the parts in later stages of production.
- Manufacturing Cylindrical or Tubular Shapes: Sheet metal curling machines can be used to form cylindrical or tubular shapes from flat sheet metal. This is often used in industries like HVAC (heating, ventilation, and air conditioning), where ducts and tubes are fabricated with curled edges for better sealing and structural integrity.
Benefits of Sheet Metal Curling Machines
- Precision and Consistency: With modern CNC systems, sheet metal curling machines can produce highly accurate and repeatable curled edges, ensuring consistent quality for every part.
- Enhanced Strength and Durability: Curling the edges of sheet metal adds reinforcement, which can help the parts withstand higher mechanical stresses and prevent edge distortion during handling or use.
- Aesthetic Appeal: The curling process provides a smooth, rounded edge that is aesthetically pleasing, making it ideal for products where the appearance of the metal is important.
- Versatility: Curling machines can handle a wide range of materials, including different types of metals such as steel, aluminum, brass, and copper. This makes them useful in many industries, from automotive manufacturing to consumer goods production.
- Increased Production Efficiency: For high-volume applications, sheet metal curling machines can streamline production by automating the edge-forming process, reducing the need for manual labor and increasing overall output.
Considerations for Choosing a Sheet Metal Curling Machine
- Material Type and Thickness: It’s essential to select a curling machine that can handle the specific type of sheet metal and thickness you plan to work with. Some machines are better suited for thin sheets, while others can handle thicker, stronger materials.
- Curl Radius and Angle: Different applications require different curl radii and angles. Machines with adjustable settings give operators flexibility in achieving the desired edge curl.
- Production Volume: For high-volume manufacturing, CNC or hydraulic curling machines offer higher efficiency and consistency. Manual or simpler machines are suitable for smaller batch sizes or less frequent use.
- Speed and Automation: For mass production, automated systems or machines with faster processing speeds can reduce cycle times, increasing productivity and reducing labor costs.
Conclusion
Sheet metal curling machines are vital tools in various industries where precise and consistent edge forming is necessary. Whether for reinforcing metal edges, creating decorative features, or preparing parts for further processing, curling machines provide high levels of flexibility, precision, and efficiency. With continuous advancements in technology, such as the integration of CNC controls, hydraulic systems, and automation, these machines will continue to improve in terms of performance, accuracy, and versatility. As industries demand more efficient and high-quality manufacturing processes, sheet metal curling machines will remain integral to the production of high-quality metal parts.
Sheet metal curling machines are essential in many industries where precision in edge forming and material handling is required. Their ability to create smooth, rounded edges on sheet metal makes them a key part of the metalworking process, especially when dealing with materials that need to be reinforced, aesthetically enhanced, or prepped for further processing. These machines cater to various industries, ranging from automotive to HVAC, where the demand for precision and consistent production is high.
The curling process helps to reinforce sheet metal, providing extra strength to edges that may otherwise be prone to bending or damage. This is especially valuable in industries like automotive manufacturing, where parts need to withstand harsh conditions and mechanical stress. By curling the edges, manufacturers can create more durable products that will perform better in the long term. Additionally, the curled edges can be designed to create stronger joints when parts are welded or otherwise fastened together, contributing to the overall structural integrity of the final product.
Beyond the functional benefits, sheet metal curling is often used for aesthetic purposes. Many consumer goods and appliances use curled edges to give the product a polished and finished appearance. For example, decorative panels, signage, and kitchen appliances often feature curled metal edges for both visual appeal and safety. These rounded edges are less likely to be sharp, which is an important safety consideration in products that come into frequent contact with people.
In high-volume production environments, curling machines can significantly reduce the time and labor required to finish sheet metal parts. Fully automated machines can continuously process large quantities of materials, cutting down on manual labor and ensuring that each part is consistent in quality. The ability to fine-tune settings for various materials and production needs allows manufacturers to achieve high levels of efficiency without sacrificing precision.
Another crucial aspect of sheet metal curling is its role in preparing metal parts for further processing. After curling, parts are often sent to additional stages of manufacturing, such as welding, forming, or bending. The curled edges make it easier to handle and align the parts, and they may even be essential for certain types of joining techniques. For example, curled edges can be used in forming tubes or ductwork in HVAC systems, where metal parts need to interlock tightly for better sealing.
In terms of the future, the sheet metal curling machine is expected to continue evolving. As automation and robotics become more integrated into manufacturing, curling machines will become part of larger automated production lines. With the use of AI-powered controls and IoT integration, these machines will be able to automatically adjust settings based on the material being processed, the desired curl size, and even real-time production feedback. This will reduce the likelihood of human error and allow operators to focus on optimizing other aspects of production, leading to a more efficient and streamlined process.
Furthermore, advancements in materials will allow sheet metal curling machines to handle a broader range of metals, including newer high-strength alloys and composites. This opens up new possibilities for industries like aerospace, where the need for precise, lightweight, and durable parts is essential. As manufacturers demand more versatility, curling machines will become more adaptable, able to handle diverse materials and different thicknesses with ease.
Overall, sheet metal curling machines are becoming more efficient, versatile, and technologically advanced. With smart controls, automation, and advanced materials, these machines will continue to play a critical role in the manufacturing of high-quality metal products. Their ability to provide strength, functionality, and aesthetics makes them invaluable tools for industries ranging from automotive to electronics, where the need for precision and consistency is paramount.
As the sheet metal industry continues to evolve, the role of sheet metal curling machines will expand beyond traditional applications, adapting to the increasingly complex demands of modern manufacturing. The integration of advanced technologies will allow these machines to become even more intelligent, capable of making real-time adjustments during the curling process. This will lead to higher levels of precision and greater flexibility in handling a diverse range of materials and geometries.
One of the key drivers of this transformation is the adoption of smart manufacturing systems. With the increasing presence of Industrial Internet of Things (IIoT) technology in manufacturing environments, curling machines will be able to communicate directly with other machines and central control systems. This integration will allow for better coordination across the entire production line. For instance, if a curling machine detects an anomaly in the material or curling process, it can alert the operator or automatically adjust to correct the issue. This level of automation and self-correction not only improves efficiency but also reduces the risk of defects and the need for manual intervention.
The evolution of data analytics in manufacturing will also play a significant role in the advancement of sheet metal curling machines. As these machines generate vast amounts of data during operation, this data can be analyzed to detect trends and patterns that might indicate wear on components, impending machine failure, or inefficiencies in the curling process. By using this information, manufacturers can adopt a predictive maintenance approach, reducing downtime and maintenance costs while ensuring the curling machines are always running at peak performance.
Additionally, advanced sensor technology will allow curling machines to provide real-time feedback on key parameters such as material thickness, surface condition, and curling radius. This will further improve quality control, as adjustments can be made instantly to account for variations in material properties, ensuring that the final product meets the required specifications. Sensors can also help track the wear and tear of rollers or dies, signaling when maintenance or replacement is necessary, thus extending the lifespan of the machine.
As material science progresses, the types of metals that sheet metal curling machines can handle will expand. This is particularly important as industries push for the use of lighter, stronger materials to meet environmental goals or improve the performance of their products. Aluminum, titanium alloys, and even composite materials are gaining traction in sectors like automotive, aerospace, and renewable energy. For instance, in the automotive industry, manufacturers are increasingly looking to reduce vehicle weight to improve fuel efficiency and reduce emissions. Curling machines will need to adapt to these materials, ensuring they can form precise, strong curled edges without compromising the material’s integrity.
The shift towards sustainability will also have an impact on the sheet metal curling industry. Manufacturers will seek more energy-efficient solutions that reduce consumption and minimize waste. Machines that can use less power and regenerate energy during operation will become more desirable. Additionally, with increased pressure to reduce material waste, there will be a growing demand for curling machines that can optimize material usage, ensuring that scrap rates are minimized, and raw material costs are kept under control.
Moreover, with an increasing number of industries turning toward customization and shorter production runs, the ability to quickly switch between different curl sizes, shapes, and material types will become essential. The incorporation of modular tooling systems and programmable rollers will allow manufacturers to easily adjust the machine setup for varying production needs, enabling them to cater to small-batch, custom, or prototype production without requiring significant downtime or retooling.
For small to medium-scale manufacturers, the availability of affordable, compact curling machines with advanced features will open new opportunities. These machines will allow smaller companies to compete in markets that require high-quality sheet metal parts, enabling them to access industries such as electronics, medical devices, and even the fashion industry, where metal components are increasingly used in jewelry or wearable tech.
On a larger scale, as demand for global production increases, manufacturers will need to ensure that their operations are scalable. Cloud-based systems and remote monitoring will enable manufacturers to track and manage multiple curling machines across different locations or production facilities. This can be particularly useful for companies operating in global supply chains, allowing them to oversee production in real-time and maintain consistent quality standards across multiple plants.
In the future of sheet metal curling, there is also the potential for more custom-designed machines for specialized applications. Manufacturers will look for machines that can handle unique materials or create complex forms that are not possible with traditional methods. For example, the aerospace industry may require precise curls on lightweight but high-strength alloys, while the furniture industry could need machines capable of curling decorative metals into intricate shapes and designs.
As industrial automation continues to rise, the role of human operators will evolve from direct machine control to overseeing systems that require minimal intervention. Operators will increasingly focus on monitoring machine performance, quality assurance, and production optimization, rather than engaging in the manual operation of machines. The advent of augmented reality (AR) and virtual reality (VR) may also provide enhanced training experiences, offering immersive ways for operators to understand machine functions and troubleshoot potential issues without being physically present.
The cost-effectiveness of sheet metal curling machines will remain a critical factor in determining their widespread adoption. As technology advances, the price of advanced machines will likely decrease, making them more accessible to a broader range of manufacturers, including those with limited capital investment. This democratization of technology will allow small and medium-sized businesses to take advantage of the same capabilities that were once only available to larger enterprises, leveling the playing field in many industries.
Ultimately, the future of sheet metal curling machines will be shaped by the ongoing pursuit of greater efficiency, precision, flexibility, and sustainability. These machines will continue to evolve to meet the ever-changing demands of global industries, ensuring that manufacturers can produce high-quality, durable, and aesthetically pleasing products with reduced environmental impact and optimized production processes. As industries embrace Industry 4.0 technologies, sheet metal curling machines will be at the forefront of the next generation of smart manufacturing, where automation, data analytics, and advanced material handling converge to drive innovation and progress across the sector.
Sheet Metal Hemming Machine
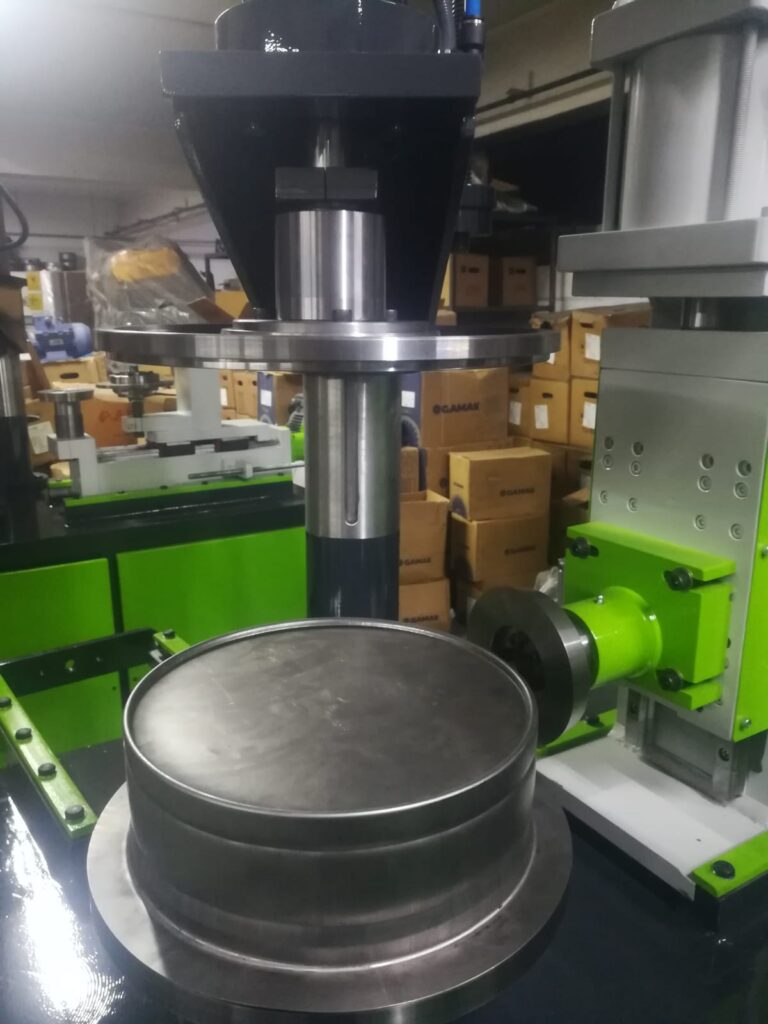
A Sheet Metal Hemming Machine is a specialized machine used in the metalworking industry to create a hem on the edges of sheet metal parts. Hemming involves folding the edges of the metal over itself to form a smooth, reinforced, and often aesthetically pleasing edge. The process improves the part’s structural integrity and can be used for both functional and cosmetic purposes. Hemming is commonly used in industries such as automotive manufacturing, appliance production, and HVAC systems.
The main purpose of a hemming machine is to produce a clean, strong, and finished edge on a variety of sheet metals, including steel, aluminum, and other alloys. Hemming is particularly important in applications where the edges of metal sheets need to be folded over to either strengthen the part or give it a smooth finish. Hemmed edges are often seen in automobile doors, trunk lids, roof panels, and appliance housings, where a neat, smooth finish is essential both for functional purposes and aesthetic appeal.
Key Components and Features
- Die Set: The die set is a critical part of a hemming machine, consisting of a male and female die that work together to form the hem. The male die pushes the sheet metal over the female die to create a uniform fold at the edges.
- Punch and Press Mechanism: The punch is used to fold the sheet metal over the die, applying pressure to ensure a tight, consistent hem. The press mechanism is often hydraulic, pneumatic, or mechanical, depending on the design of the machine and the material being worked on.
- Feed and Positioning System: The sheet metal is fed into the hemming machine and positioned accurately to ensure that the hems are consistent across the entire length of the part. This can be done manually or automatically, with many modern machines featuring automated feeding systems to increase speed and precision.
- Hemming Rollers: Some advanced hemming machines use a set of rollers to complete the hemming process. These rollers gradually bend the edge of the metal, often in multiple passes, until the desired angle and shape are achieved.
- Control System: Modern hemming machines are typically equipped with CNC (Computer Numerical Control) systems that allow operators to program specific hemming angles, radii, and feed speeds. This allows for precise control and ensures consistent results across different production runs.
- Hydraulic or Pneumatic Power: Depending on the machine, hydraulic or pneumatic systems are used to apply the necessary force to create the hem. These systems provide the strength required to bend thicker metals without causing damage or defects.
- Safety Features: Given the forces involved in the hemming process, modern machines are equipped with various safety features to protect operators. These include emergency stop buttons, safety guards, and automatic shutoff systems that activate if the machine detects any anomalies during operation.
Types of Hemming Machines
- Manual Hemming Machines: These are basic machines where the operator manually feeds the metal sheet and operates the punch to form the hem. These machines are typically used for small production runs or for manufacturers that only need to hem parts occasionally.
- Automated Hemming Machines: Automated models are designed for high-volume production. These machines feature automatic feeding, positioning, and control systems, making them ideal for mass production environments. The automation ensures uniformity in the hemming process and increases throughput.
- Roller Hemming Machines: These machines use rollers to fold the sheet metal edges over progressively, usually in multiple passes. They are particularly useful for parts that require a more gradual, less aggressive bending process. Roller hemming is often used in industries like automotive manufacturing, where parts need to maintain specific structural properties.
- CNC Hemming Machines: These advanced machines are controlled via a CNC system, allowing for precise programming and adjustment of hemming parameters. They are used in applications that require high levels of precision and repeatability, such as in the aerospace and automotive industries.
Applications of Sheet Metal Hemming Machines
- Automotive Manufacturing: Hemming is widely used in automotive assembly for parts such as doors, trunk lids, roof panels, and fenders. The hem provides a smooth, finished edge and reinforces the part’s structure. In some cases, hems are used to conceal sharp edges, which improves safety and appearance.
- Appliance Industry: Household appliances such as washers, dryers, and microwave ovens often have hemmed edges for both strength and appearance. The hemming process ensures that parts are structurally sound while providing a clean, professional look.
- HVAC Systems: Sheet metal hems are used in the construction of ductwork, ventilation systems, and other components where edges need to be reinforced or covered. Hemming improves the strength and durability of these parts, which are often subjected to mechanical stress.
- Furniture and Decorative Metalwork: In industries where metal parts serve decorative or functional purposes, hemming provides an aesthetic finish and improves the structural performance of the part. This is common in metal furniture, signage, and architectural elements.
- Electronics Enclosures: Enclosures for electronic components, including server racks and computer housings, often feature hemmed edges to provide additional strength, support, and protection against external forces.
Benefits of Sheet Metal Hemming
- Increased Strength: Hemming strengthens the edges of sheet metal parts, providing additional resistance to deformation and mechanical stress. This is particularly important in industries like automotive manufacturing, where parts are subject to impact forces.
- Aesthetic Quality: Hemming creates a smooth, clean edge that enhances the appearance of metal parts. This is important for products that will be visible to consumers, such as in the automotive or appliance industries.
- Improved Safety: By folding the edges of sheet metal, the hemming process eliminates sharp, dangerous edges that could cause injury during handling or in the finished product.
- Cost Efficiency: Hemming can reduce the need for additional finishing operations, such as deburring or edge grinding. By incorporating hemming into the manufacturing process, companies can save time and reduce costs.
- Versatility: Hemming machines can be used on a wide variety of metals, including aluminum, stainless steel, and mild steel. This versatility makes them suitable for use in many different industries and applications.
Considerations for Choosing a Sheet Metal Hemming Machine
- Production Volume: For high-volume production, automated or CNC-controlled hemming machines are recommended due to their speed and consistency. For smaller production runs, manual or semi-automated machines may be more cost-effective.
- Material Type and Thickness: The thickness and type of metal being processed will influence the choice of machine. Thicker materials may require hydraulic machines with greater force capabilities, while thinner metals can often be processed with lighter, more economical machines.
- Edge Specifications: The specific type of hem required (e.g., closed hems, open hems, or double hems) will determine the machine setup. It is important to choose a machine capable of handling the particular edge specifications needed for the parts being produced.
- Automation Requirements: If the goal is to achieve a high level of consistency across production runs, automated or CNC-controlled machines offer the best solution. These machines allow for precise control over the hemming process and can easily switch between different part configurations.
- Space and Budget: The size of the machine and the budget available will also impact the choice. While automated machines can be larger and more expensive, they may be necessary for large-scale production. Smaller operations or companies with limited budgets may opt for simpler manual or semi-automated machines.
Conclusion
Sheet metal hemming machines are crucial for industries that require high-quality, durable, and aesthetically pleasing edges on sheet metal parts. They provide strength, improve safety, and enhance the visual appeal of products across various sectors, from automotive and appliances to electronics and HVAC systems. With the continued advancement of automation, CNC control systems, and material handling technologies, the hemming process is becoming more efficient, precise, and cost-effective. The future of sheet metal hemming will likely involve even greater integration with smart manufacturing systems, allowing manufacturers to produce high-quality parts at an even faster rate, with greater precision and minimal waste.
Sheet metal hemming machines play a vital role in a variety of industries, providing efficient, consistent, and high-quality edge finishing for sheet metal parts. Their ability to create smooth, reinforced edges not only enhances the structural integrity of components but also improves safety and aesthetics, making them crucial in the manufacturing of products like automotive parts, household appliances, and HVAC systems. These machines are designed to fold the edges of the metal over itself, providing a clean and finished look that is both functional and visually appealing.
Hemming can be used for different purposes. On one hand, it reinforces sheet metal parts, especially in applications where parts will undergo mechanical stress. In automotive manufacturing, for example, hems on doors and other panels increase strength, preventing deformation and enhancing durability. On the other hand, hems provide a polished finish to parts that are visible to consumers, such as appliances and consumer electronics, where a smooth, clean edge improves both the functionality and the overall appearance of the product.
The versatility of these machines allows them to handle various materials, from aluminum to high-strength steels. Depending on the application, the machines are designed to accommodate different thicknesses and material types, ensuring they can perform the hemming process effectively and accurately. The strength of the machine, especially in automated systems, allows for greater control and uniformity across production runs, which is particularly valuable in high-volume manufacturing environments. In these settings, CNC-controlled or automated hemming machines offer precision and efficiency, minimizing human intervention while ensuring consistent results.
Moreover, advancements in technology have led to the incorporation of IoT and AI into hemming machines, allowing for real-time monitoring and adjustments during production. This integration ensures that any inconsistencies or issues are detected and addressed immediately, reducing the chances of defects and increasing the overall efficiency of the manufacturing process. These machines can be programmed to automatically adjust the hemming parameters, such as angle and force, to meet the specific requirements of the material and part being processed. This flexibility is important in industries that produce a wide variety of parts, each with different specifications.
The process of hemming also contributes to cost savings by eliminating the need for additional finishing processes such as deburring, grinding, or edge smoothing. The hemmed edges are typically clean and safe to handle right off the machine, which reduces the time and resources spent on post-production finishing. As a result, companies can streamline their operations, reduce waste, and lower production costs.
One of the ongoing trends in the industry is the shift toward sustainability. With a growing focus on reducing environmental impact, manufacturers are seeking more energy-efficient machines and processes. Modern sheet metal hemming machines are designed to minimize energy consumption while improving production efficiency. In addition, as industries continue to push for lighter and stronger materials, these machines will adapt to handle newer alloys and composites, ensuring that manufacturers can meet the demands of emerging technologies.
In addition to improving efficiency and quality, hemming machines are becoming more compact and user-friendly, making them accessible to smaller manufacturers and companies with limited space. These advancements enable businesses to adopt high-quality edge finishing processes without needing large, expensive equipment. The affordability and compact nature of these machines open up new possibilities for small to medium-sized enterprises to compete in industries that require precise and consistent metalwork.
As industries continue to evolve and demand more advanced materials, customization, and flexible production, sheet metal hemming machines will remain essential. Their ability to produce high-quality, durable, and visually appealing parts will ensure they remain indispensable in sectors such as automotive, aerospace, electronics, and construction. With ongoing advancements in automation, smart manufacturing, and material science, the future of hemming technology is poised to offer even greater precision, versatility, and efficiency, enabling manufacturers to meet the challenges of an ever-changing marketplace.
As the demand for more specialized and high-performance metal components grows, sheet metal hemming machines will continue to evolve to meet the needs of modern manufacturing. The integration of robotics and artificial intelligence will allow for even more sophisticated control over the hemming process, enhancing the ability to handle complex geometries and new materials. This could be particularly important in industries like aerospace, where components need to be both lightweight and extremely strong. The combination of advanced sensors and machine learning algorithms will ensure that each part is formed with the utmost precision, reducing the likelihood of defects and ensuring that parts meet strict tolerances.
In addition to the technological improvements in hemming machines themselves, the development of advanced materials will shape the future of the industry. As new materials such as carbon fiber composites, titanium alloys, and ultra-high-strength steels become more common in sectors like aerospace and automotive manufacturing, sheet metal hemming machines will need to adapt to process these harder and more complex materials. These materials often require specialized processes due to their unique properties, such as increased hardness or the need for heat management during forming. Hemming machines will incorporate new techniques and innovations to deal with the challenges posed by these materials, ensuring that the hemming process can maintain the same level of precision and quality as with traditional sheet metals.
Another important area of growth is the increased automation of the entire production line. Sheet metal hemming machines will increasingly become part of integrated, automated systems where they work in conjunction with other machines such as press brakes, laser cutters, and welding stations. These systems will allow for just-in-time production, where parts are produced as needed without excess inventory or delays. The seamless coordination between machines and automatic data exchange will ensure smooth production flows, reducing downtime and improving overall efficiency.
The focus on sustainability in manufacturing processes will also continue to influence the development of sheet metal hemming machines. Manufacturers are expected to move toward zero-waste production practices, and recyclability will become a key consideration in the design of machines. Hemming machines will likely be developed with more efficient energy use and materials management in mind. Furthermore, they may incorporate features that allow for material reclamation or reuse of scrap metal, helping reduce the environmental footprint of the production process.
Another trend in the future of sheet metal hemming machines is the customization of parts. As consumer demand for personalized products rises, the ability to easily modify machine settings to produce unique or custom-shaped parts will become increasingly important. CNC-controlled hemming machines are particularly suited for this purpose, as they can quickly adjust to different part specifications. The flexibility to switch between different part designs with minimal setup time will allow manufacturers to better serve industries that require low-volume, high-precision production.
As businesses strive for greater operational flexibility, modular hemming machines will become more common. These machines, designed with interchangeable components, will allow manufacturers to easily swap out parts for different types of materials, sizes, or applications. This modular approach will not only reduce downtime but also make it easier for manufacturers to adapt to changes in customer demand or new product lines.
In addition, remote monitoring and maintenance capabilities will be increasingly incorporated into sheet metal hemming machines. These systems allow operators to track the performance and health of their machines remotely, providing predictive maintenance alerts based on the data gathered from sensors and connected systems. This reduces the likelihood of unexpected breakdowns and improves the overall uptime of the machine, contributing to a more efficient production process.
The growing demand for smart manufacturing systems will also push the development of advanced machine interfaces for sheet metal hemming machines. These interfaces will provide operators with real-time feedback and detailed analytics on machine performance. They will allow for quick adjustments to parameters such as pressure, speed, and angle, optimizing production based on immediate feedback and data trends. Operators will be able to interact with the machines through touchscreen displays or even augmented reality (AR) interfaces, which will provide step-by-step instructions and real-time error diagnostics.
Finally, the development of hybrid manufacturing techniques that combine traditional sheet metal forming with additive manufacturing (3D printing) will likely impact the future of hemming. These hybrid systems could allow manufacturers to create more complex, lightweight, or customized parts by combining the strength of traditionally formed metal edges with the intricate geometries made possible by 3D printing. This fusion of technologies could open new possibilities for industries such as aerospace, where parts need to be both strong and lightweight, and where customization is often key.
In conclusion, the future of sheet metal hemming machines looks bright, with continued advancements in technology, automation, and materials science. These machines will become more precise, versatile, and sustainable, enabling manufacturers to meet the increasing demands for high-quality, customized, and environmentally responsible metal components. The ongoing integration of AI, machine learning, robotics, and IoT will further enhance the performance and capabilities of hemming machines, making them an integral part of the smart manufacturing ecosystem. With these innovations, manufacturers will be better equipped to address the challenges of modern production and meet the evolving needs of their industries.
Sheet Metal Flanging Machine
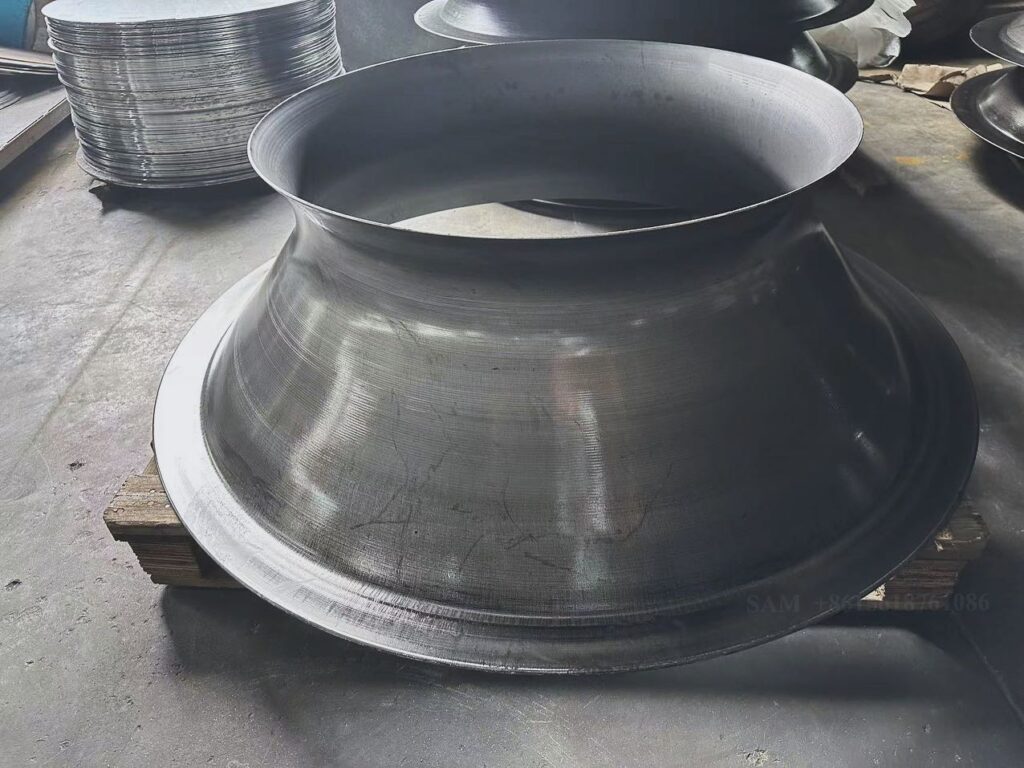
A Sheet Metal Flanging Machine is used to create flanges on sheet metal parts by bending the edges outward, typically at a right angle or in a more complex shape, depending on the design requirements. The flanging process is often used to prepare metal parts for joining, such as through welding or bolting, and is common in industries where metal parts need to be connected securely, like in the automotive, aerospace, and construction sectors. The purpose of flanging is to add strength, provide a flat surface for fasteners, or prepare the metal for further processing like seaming or crimping.
Key Functions and Applications
Flanging is important in a variety of manufacturing processes, as it enhances the rigidity and stability of sheet metal components and allows for easier assembly. Some of the most common applications include:
- Automotive Industry: Flanges are used in car body panels, engine components, and exhaust systems. The flanged edges help with fitting parts together, allowing for stronger joints or easier assembly using bolts and screws.
- Aerospace Industry: In aerospace, flanged edges are often used in the construction of aircraft wings, fuselages, and control surfaces. Flanges ensure that the parts can be securely fastened together and can withstand the high mechanical stresses that these components are exposed to.
- HVAC Systems: In heating, ventilation, and air conditioning systems, flanges are frequently used to connect ductwork, ventilation pipes, and other components. The flanged edges allow for secure and leak-proof connections.
- Construction: Sheet metal flanges are commonly found in the fabrication of structural steel, roofing systems, and metal framing. The flanges provide strong connections between metal elements and are used in the creation of prefabricated panels.
- Industrial Machinery: Many types of machinery use flanged parts for assembly and maintenance. For example, pipes, valves, and filters often have flanged edges to make them easier to install and remove.
Key Components of a Sheet Metal Flanging Machine
- Die and Punch Set: The machine typically uses a set of dies and punches to perform the flanging operation. The male die is shaped to match the flange’s design, while the female die provides the counterpart into which the metal is bent. The punch applies pressure to bend the edge of the sheet metal into the desired shape.
- Press Mechanism: A hydraulic, pneumatic, or mechanical press is used to apply the force required to bend the metal edge over the die. The press ensures consistent pressure to form the flange, allowing for precise control over the shape and angle of the flange.
- Feed System: A manual or automatic feed system positions the sheet metal accurately under the die to ensure uniform flanging across multiple parts. Advanced machines may include CNC control systems to adjust for different flange sizes and positions.
- Control Systems: Modern flanging machines are typically equipped with CNC (Computer Numerical Control) systems, which enable precise adjustments to the flange’s shape and size. Operators can program the machine to form flanges at specific angles, widths, and lengths based on the part’s specifications.
- Clamping Mechanism: To ensure that the sheet metal is securely held in place during the flanging process, many machines are equipped with clamps or grippers that hold the metal firmly against the die.
- Tooling and Adjustments: The tooling used in flanging machines can often be changed to accommodate different metal thicknesses and flange shapes. Some machines include adjustable guide rails, tool holders, and height adjustments to tailor the process to various materials and production requirements.
Types of Sheet Metal Flanging Machines
- Manual Flanging Machines: These are simple machines operated by hand, where the metal sheet is manually positioned and the press is operated by the worker. These machines are best for small-scale or low-volume production where a high degree of automation is not necessary.
- Automated Flanging Machines: These machines use automatic feeding, positioning, and adjustment systems to perform the flanging process. Automated flanging machines are suited for high-volume manufacturing and are often found in mass production environments. They can handle multiple parts in quick succession and ensure consistent quality.
- CNC Flanging Machines: CNC-controlled flanging machines are highly precise and can produce flanges with complex geometries and tight tolerances. These machines are commonly used in industries like aerospace, automotive, and custom fabrication where exact specifications are critical.
- Rotary Flanging Machines: These machines are used to produce continuous or large-diameter flanges. The sheet metal is fed through a rotating die and punch, forming the flange progressively. This type of machine is useful for applications like manufacturing ductwork or pipes.
- Hydraulic Flanging Machines: Hydraulic flanging machines use hydraulic force to apply pressure to the metal sheet. They are particularly effective for processing thicker or harder metals and can create flanges with significant strength. These machines are often used in industries such as heavy equipment manufacturing and structural metalworking.
Advantages of Sheet Metal Flanging Machines
- Strength and Durability: Flanging adds strength to sheet metal components, enabling them to withstand mechanical stress and external forces. The flange creates a larger surface area for attachment, which helps in making more durable and robust connections.
- Enhanced Assembly: By adding flanges to the edges of metal parts, manufacturers make it easier to join parts together using fasteners like bolts, rivets, or welding. This simplifies the assembly process, especially for large or complex structures.
- Improved Aesthetics: Flanging provides a clean, finished edge, which is aesthetically pleasing, particularly in consumer-facing products like appliances, automotive parts, and electronics. It also eliminates sharp edges, reducing the risk of injury during handling.
- Cost-Efficiency: Flanging machines are generally efficient, allowing manufacturers to produce high volumes of parts with minimal setup time. The consistent, precise nature of the process ensures that parts meet the required specifications without the need for additional post-processing.
- Flexibility: Flanging machines are versatile and can be adjusted to produce different types of flanges, from simple right-angle flanges to more complex shapes. This makes them suitable for a wide range of industries and applications.
- Reduced Waste: By accurately forming flanges with minimal material deformation, these machines reduce material waste, ensuring that manufacturers can maintain high levels of productivity while minimizing costs associated with scrap metal.
Challenges and Considerations
- Material Thickness: The thickness and type of sheet metal being used can affect the flanging process. Thicker materials may require more powerful machines to achieve the desired flange shape, while thin materials may be prone to deformation or damage during the process.
- Tool Wear: Over time, the dies and punches used in flanging machines can wear down, leading to less precise flanges. Regular maintenance and tool replacement are essential to ensure the machine continues to operate at peak performance.
- Precision Requirements: For industries that require high precision, such as aerospace and automotive manufacturing, flanging machines must be able to produce flanges with tight tolerances. This can require more advanced machinery, like CNC-controlled systems, which come with higher upfront costs.
- Production Speed: While automated flanging machines can process large volumes of parts quickly, there may be limitations in the speed at which the machine can perform certain tasks, especially when dealing with highly complex parts or multiple material types.
- Customization: Custom parts with specialized flange designs may require specific tooling and setup, adding to the complexity and cost of production. Some machines may need to be modified or adjusted to accommodate these custom designs.
Conclusion
Sheet metal flanging machines are indispensable tools in industries that require strong, durable, and easily assembled sheet metal parts. From the automotive and aerospace sectors to HVAC and industrial machinery, these machines provide a quick and efficient way to create secure, strong, and precise flanges. Whether in high-volume production or custom fabrication, the flexibility, precision, and cost-effectiveness of flanging machines make them a key part of the metalworking industry. As manufacturers continue to push the boundaries of material science and automation, the development of advanced flanging machines will allow for even greater levels of efficiency, accuracy, and customization in sheet metal processing.
Sheet metal flanging machines play a pivotal role in producing high-quality, strong, and precise flanges for a variety of applications. The process of flanging, which involves bending the edges of a metal sheet to form a flange, helps create stronger connections between parts, enhances assembly processes, and ensures that parts meet specific design and functional requirements. These machines are used in industries such as automotive, aerospace, HVAC, construction, and industrial machinery, among others.
One of the main benefits of sheet metal flanging machines is the ability to create a strong, reinforced edge on metal components. The flange improves the structural integrity of the part, making it more durable and resistant to deformation under stress. In automotive applications, flanged edges are often used in body panels, exhaust systems, and engine components, where strength and security are critical. Similarly, in the aerospace industry, flanged edges help ensure the stability and strength of components like wings, fuselages, and control surfaces.
In addition to enhancing strength, flanging machines also contribute to the efficiency of the manufacturing process. By producing clean and consistent flanged edges, these machines simplify the assembly process, making it easier to attach parts using bolts, rivets, or welds. This can save time and reduce the likelihood of errors during the assembly phase. Furthermore, the smooth, finished edges produced by flanging machines improve the overall appearance of the parts, which is particularly important in industries like consumer electronics or appliances, where aesthetics are key.
Flanging machines vary widely in design and function, offering different features and capabilities to suit a wide range of production needs. Manual flanging machines are typically simple, hand-operated machines used for low-volume production or custom work. These machines are ideal for situations where precise control over the flanging process is required but where automation is not necessary. On the other hand, automated and CNC-controlled flanging machines are designed for high-volume production and provide greater precision, allowing for tighter tolerances and more complex flange designs. These machines are particularly useful in industries that require high accuracy and consistency, such as aerospace or automotive manufacturing.
The versatility of flanging machines is also evident in their ability to handle a variety of materials, including steel, aluminum, and other alloys. Depending on the material being processed, the machine may need to apply varying amounts of pressure and force to achieve the desired flange shape. Some machines use hydraulic or pneumatic presses to provide the necessary pressure, while others may rely on mechanical systems for simpler operations.
Over time, the advancements in automation and machine control technology have significantly improved the efficiency and performance of flanging machines. Modern machines are equipped with sensors, programmable controls, and automated feeding systems that ensure greater accuracy and minimize human error. These systems can also be integrated with other machines on the production line, allowing for seamless and continuous manufacturing workflows. This is especially beneficial in environments where large quantities of parts need to be produced quickly without sacrificing quality.
The sustainability aspect of manufacturing is also becoming increasingly important, and sheet metal flanging machines are no exception. Manufacturers are focusing on designing machines that reduce energy consumption, minimize material waste, and increase overall efficiency. Some machines are designed to reclaim scrap metal during the flanging process, allowing for recycling and reducing the environmental impact of production. These machines help manufacturers maintain a more sustainable operation while also reducing operational costs.
As industries continue to evolve and demand more customization and higher performance from their parts, sheet metal flanging machines will continue to play a crucial role. The growing use of advanced materials and the increasing complexity of designs will require even more sophisticated machines that can handle new challenges. In particular, the ongoing development of smart manufacturing techniques, such as IoT integration and real-time monitoring, will further enhance the precision and efficiency of flanging machines, enabling them to operate more autonomously and with greater flexibility.
Flanging machines will continue to be essential in helping manufacturers produce high-quality, durable, and aesthetically pleasing metal parts that meet the rigorous demands of modern industries. With the ongoing advances in automation, materials science, and machine technology, the future of sheet metal flanging machines looks promising, offering even greater opportunities for precision, speed, and customization in sheet metal fabrication.
As the demand for advanced manufacturing capabilities increases, the future of sheet metal flanging machines is bound to be shaped by emerging trends in automation, smart technology, and material science. One of the key developments in the field is the integration of Industry 4.0 concepts, which includes connected devices, machine learning, and real-time data analytics. These innovations allow manufacturers to achieve higher levels of precision and efficiency in the flanging process, as well as providing predictive maintenance capabilities.
Machine learning algorithms will enable flanging machines to adjust and optimize their operation based on real-time data inputs, such as the material’s thickness, hardness, and the desired flange dimensions. This level of adaptability ensures that the machine is always operating at peak performance, minimizing errors and improving part consistency. Additionally, real-time monitoring of key parameters such as pressure, speed, and temperature will help operators track the machine’s condition and performance, enabling them to make adjustments proactively before any problems arise.
Furthermore, robotic automation is expected to play a larger role in sheet metal flanging. Robots can be used for loading and unloading sheet metal from the machine, which increases the throughput of the manufacturing process. When combined with advanced control systems, robotic arms can also assist in positioning and handling large, complex, or delicate parts during the flanging process. By using robots for these tasks, manufacturers can reduce labor costs and improve safety by minimizing human interaction with the machines.
The integration of augmented reality (AR) and virtual reality (VR) technologies is also making its way into sheet metal forming machines, including those used for flanging. These technologies can provide operators with real-time visual feedback on the part’s formation, enabling them to detect and correct any issues in the flanging process more quickly. Operators can use AR glasses or VR interfaces to visualize how the metal is being deformed and to ensure that it is shaped correctly according to the design specifications.
Material science advancements will also influence the future of sheet metal flanging machines. As new materials with different properties—such as lightweight alloys, composites, and high-strength steels—become more prevalent, flanging machines will need to evolve to handle these materials effectively. For example, lightweight materials like aluminum and magnesium may require less pressure to form, while advanced high-strength steels may need additional force or specialized tooling to avoid cracking or warping during the flanging process.
Additionally, the growing demand for sustainability in manufacturing is pushing flanging machine designs toward more energy-efficient systems. As industries become more conscious of their environmental impact, manufacturers are seeking machines that consume less power and generate less waste. New flanging machines are being designed with energy-efficient motors and closed-loop systems to help minimize the carbon footprint of the production process.
The need for more customization in products is another factor driving innovation in flanging machine technology. Consumers and industries alike are looking for more tailored, personalized solutions, which means that flanging machines will need to handle a broader range of part sizes, shapes, and specifications. The ability to quickly switch between different flanging profiles, without the need for extensive retooling, will be essential for manufacturers working in industries like aerospace or automotive, where custom components are often required.
As the trend toward miniaturization continues, there will likely be increased demand for more compact and flexible flanging machines that can be easily integrated into smaller, more specialized production lines. Modular designs could allow manufacturers to scale up or down according to their needs, making it easier to produce both high-volume and custom parts efficiently. These compact systems can be used in industries where space is limited, such as smaller workshops or specialized facilities.
The future of sheet metal flanging machines is also closely tied to globalization and the need for supply chains to be more responsive and flexible. The ability to produce parts locally, with fast turnaround times and minimal waste, will be increasingly valuable as industries move toward on-demand manufacturing and shorter product life cycles. The integration of cloud computing and big data will allow manufacturers to share information across the supply chain and respond more quickly to changing demands or production issues.
Lastly, as automation and digital technologies continue to progress, operators will benefit from user-friendly interfaces that make it easier to set up, monitor, and troubleshoot flanging machines. Touchscreen controls and intuitive software will enable even novice operators to manage complex operations without needing extensive technical training. The rise of smart factories will ensure that the entire manufacturing process, including flanging, is seamlessly integrated with other stages of production, reducing downtime and improving overall throughput.
In summary, sheet metal flanging machines are poised to become more automated, connected, and adaptive in the coming years. Advancements in machine learning, robotics, and augmented reality will allow for more precision and efficiency, while developments in material science will enable flanging machines to handle a wider range of materials. At the same time, the push for sustainability, customization, and smarter manufacturing will drive innovation in machine design and functionality. These trends will ensure that flanging remains a critical process in metalworking for years to come, with machines that are better equipped to meet the evolving needs of manufacturers worldwide.
Sheet Metal Deep Drawing Machine
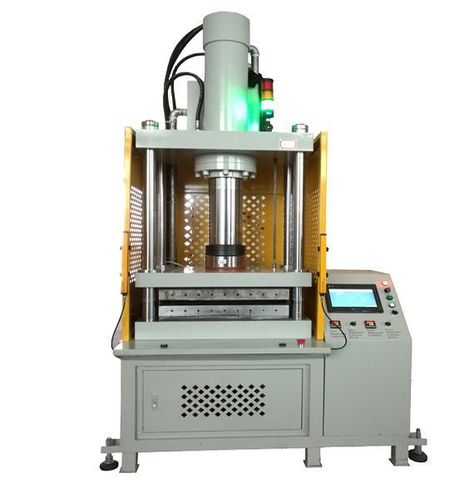
A Sheet Metal Deep Drawing Machine is used in the metalworking industry to create deep, cup-like shapes from flat sheet metal by subjecting it to a drawing process. This is a type of forming process where a flat metal sheet is mechanically drawn into a die, which shapes it into a deeper form without cracking or losing its integrity. This process is widely used in industries such as automotive manufacturing, appliance production, aerospace, medical devices, and consumer goods.
The deep drawing process is most commonly applied to stainless steel, aluminum, and copper, though it can be used on other metals as well. It is ideal for producing parts with complex shapes, deep profiles, and smooth surfaces that are difficult to achieve through other methods, such as stamping or casting.
How It Works
- Material Setup: The process starts with a flat sheet of metal, often called a blank, which is positioned on the die of the machine. The size of the blank will depend on the dimensions of the finished part and the depth of the drawing process.
- Blank Holder: The blank holder is used to secure the sheet metal in place and maintain a uniform pressure across the material as it is drawn into the die. The blank holder ensures that the material does not wrinkle or buckle during the drawing process.
- Punching: The drawing process begins when the punch, a tool with the shape of the finished part, moves downward, pushing the sheet metal into the die cavity. The punch and die work together to form the metal into the desired shape. The pressure exerted by the punch causes the metal to flow into the die, creating the deeper shape.
- Material Flow: As the punch presses the sheet metal into the die, the material stretches, creating the desired depth. The deep drawing process typically works well for forming parts with relatively simple shapes, like cylindrical cups, but can also be used to create more complex geometries with multiple deep cavities.
- Tooling and Die: The die and punch are specially designed to match the shape of the final product. A deep drawing die often consists of two parts: the female die (the cavity into which the metal is drawn) and the male punch (the tool that pushes the metal into the die). In some machines, there are additional tools, such as lubrication systems or guide rails, to assist in the drawing process and prevent issues like metal wrinkling or tearing.
- Finishing: Once the metal has been drawn into the die, the formed part is removed and often undergoes secondary processes such as trimming, deburring, or smoothing to remove excess material or refine the final appearance of the part.
Key Components of a Sheet Metal Deep Drawing Machine
- Pressing Mechanism: Most deep drawing machines use a hydraulic, mechanical, or pneumatic press to apply the necessary force to form the metal. Hydraulic presses are commonly used for deep drawing because they can provide the large amounts of force needed to shape thicker or harder materials.
- Blank Holder: The blank holder is critical for maintaining the integrity of the sheet metal during the drawing process. It holds the sheet securely in place, preventing it from slipping or wrinkling.
- Die and Punch Set: The die and punch work together to form the desired shape. The punch pushes the metal into the cavity, and the die ensures the correct final shape is achieved.
- Lubrication System: Lubrication is often used to reduce friction between the sheet metal and the die during the drawing process. This helps prevent wrinkling, tearing, or galling of the metal.
- Guide Rails: Some deep drawing machines include guide rails to keep the punch aligned with the die and ensure uniform force distribution during the process.
Types of Sheet Metal Deep Drawing Machines
- Single-Action Deep Drawing Press: This is the most basic type of deep drawing machine, where a single press stroke is used to push the sheet metal into the die. It is ideal for relatively simple shapes or low-volume production.
- Double-Action Deep Drawing Press: In this type of machine, two separate actions are used to form the metal. The first stroke pushes the metal into the die, while the second action further shapes the part or deepens the drawing. This type of machine is used for more complex shapes that require additional forming steps.
- Triple-Action Deep Drawing Press: This machine includes three distinct actions, typically for creating deep and complex parts with multiple stages of forming. It provides even greater control over the material flow and can handle more intricate designs.
- Hydraulic Deep Drawing Press: Hydraulic presses are commonly used for deep drawing because they can provide consistent, high-force capabilities. These machines can handle both thin and thick sheets and are often preferred for materials that require high pressure to form.
- Mechanical Deep Drawing Press: Mechanical presses use cams and linkages to create the necessary force for deep drawing. They are faster than hydraulic presses but may not offer as much control over force application. These presses are typically used in high-volume production settings for simpler part designs.
- CNC Deep Drawing Machines: With the integration of CNC (Computer Numerical Control), modern deep drawing machines are capable of precise control over every aspect of the process. CNC machines allow for the adjustment of the drawing force, punch speed, and other parameters, making it easier to produce complex parts with tight tolerances.
Applications of Sheet Metal Deep Drawing Machines
- Automotive Parts: Deep drawing is widely used in the automotive industry to produce body panels, fuel tanks, engine components, and brake drums. The process is ideal for creating complex shapes that require high strength, such as bumper parts, fenders, and doors.
- Aerospace Components: In aerospace, deep drawing is used to create parts like fuel tanks, aircraft fuselages, wing skins, and landing gear components. The process provides the strength required for these critical components, which must withstand extreme pressure and stress.
- Appliance Manufacturing: Household appliances such as washers, dryers, and refrigerators often feature deep-drawn metal parts, including drums, shells, and covers. The deep drawing process allows for the efficient production of these parts while ensuring their durability and smooth appearance.
- Medical Devices: Deep drawing is also used to create parts for medical equipment, such as surgical instruments, implant components, and medical containers. The precision of deep drawing ensures that these parts meet stringent standards for functionality and safety.
- Consumer Electronics: In the production of consumer electronics, deep drawing machines are used to form parts like cases for TVs, computers, and mobile devices. The ability to create complex shapes with smooth surfaces makes deep drawing ideal for this application.
Advantages of Sheet Metal Deep Drawing
- High Strength: Deep drawing creates parts with enhanced strength because the material is stretched and compressed during the process, which can improve its mechanical properties.
- Precision: Deep drawing provides high accuracy and repeatability, making it ideal for producing parts with tight tolerances.
- Material Efficiency: The deep drawing process uses the sheet metal effectively, with minimal material waste. This is especially important for high-value materials like stainless steel, aluminum, and copper.
- Complex Shapes: Deep drawing allows for the creation of parts with complex shapes and deep profiles that would be difficult or impossible to achieve using other methods like stamping.
- Smooth Surfaces: The deep drawing process typically results in parts with smooth, consistent surfaces, which reduces the need for secondary finishing operations.
Challenges and Considerations
- Material Thickness: Dealing with thick sheet metals can be challenging, as they require more force to draw and may be more prone to cracking. Thinner sheets are generally easier to form, but thicker materials may need special tooling or more advanced techniques like multi-pass drawing.
- Wrinkling and Tearing: If the material is not properly controlled or lubricated during the deep drawing process, it can wrinkle or tear. Advanced machine setups, such as using blank holders or variable blank holders, can help mitigate these issues.
- Tooling Costs: Custom tooling for deep drawing, especially for complex shapes or high-volume production, can be expensive. Tool wear and tear also require regular maintenance and replacement, which can add to operating costs.
- Material Selection: Not all materials are suited for deep drawing. Some materials may be too brittle or lack the necessary ductility to form properly. The material must have sufficient elongation properties to avoid defects during drawing.
- Production Speed: Although deep drawing is highly efficient for producing parts in large volumes, it can be slower compared to simpler processes like stamping. The setup times and tooling changes can increase production time, especially in multi-step processes.
Conclusion
Sheet metal deep drawing machines are crucial for creating a wide range of metal parts that require complex shapes, high strength, and smooth surfaces. By using precise pressure and tooling, these machines allow manufacturers to produce intricate, deep components in industries such as automotive, aerospace, appliances, and medical devices. While there are challenges like material selection, tooling costs, and wrinkling, advancements in technology, such as hydraulic presses, CNC systems, and robotic automation, continue to enhance the capabilities and efficiency of deep drawing machines, ensuring that they remain a key process in modern manufacturing.
A Sheet Metal Deep Drawing Machine plays a pivotal role in industries that require the production of complex, deep, and precise metal parts. It is particularly important for creating shapes that are difficult to achieve with other metal forming processes, such as stamping or casting. This machine allows manufacturers to transform flat metal sheets into deeper, more intricate parts, while maintaining the integrity of the material throughout the forming process.
The deep drawing process is highly valued for its ability to produce components with significant depth without compromising on the thickness or strength of the metal. As the sheet metal is drawn into the die, it stretches, which can increase its tensile strength, making it particularly suitable for parts that need to endure mechanical stresses, such as automotive body panels, fuel tanks, and other structural components. The deep drawing process also allows for smooth, consistent surfaces, making it ideal for products that need to maintain aesthetic qualities alongside functional strength.
While it is efficient for large-scale production, deep drawing requires precision at every stage, from material selection to machine setup. The process works best with ductile metals, such as aluminum, copper, or stainless steel, that can withstand stretching without cracking. During the drawing process, the metal is pushed into a die cavity using a punch, which can be configured to create parts with different shapes and dimensions. The success of the process depends on factors like punch speed, force, lubrication, and die design, all of which need to be optimized to avoid common issues such as wrinkling, tearing, or tool wear.
In addition to its mechanical components, modern deep drawing machines often include automation systems that enhance efficiency and reduce human error. Automated systems can adjust parameters in real-time, ensuring that the drawing force is applied consistently and accurately. For manufacturers, these advancements lead to faster production times and higher throughput, making deep drawing machines ideal for industries where high-volume production is required, such as automotive or consumer electronics.
The tooling involved in deep drawing is also an essential factor. Custom dies and punches are designed for specific parts, which can make initial setup costs high. However, once in place, they allow for the high precision required for mass production. These dies must be carefully maintained to ensure that they do not wear down, as tool degradation can lead to defects in the parts being produced.
While deep drawing machines excel in producing high-strength, high-precision components, they are not without their limitations. One of the main challenges is the material thickness, as thicker materials require more force to draw and can be more prone to defects like cracks or wrinkles. Additionally, deep drawing is not suitable for all shapes—parts with intricate features that extend beyond simple depth may require alternative forming techniques.
However, with advancements in machine technology and material science, deep drawing machines are becoming more adaptable and capable of handling more complex tasks. The introduction of hydraulic presses, CNC controls, and multi-step drawing processes has significantly expanded the capabilities of deep drawing machines, allowing them to tackle even more demanding projects with ease. Modern machines often feature automated tool changers and real-time monitoring systems that provide feedback on material flow, die alignment, and part quality, further enhancing the machine’s flexibility and precision.
Sustainability is also becoming an increasingly important consideration in the design and operation of sheet metal deep drawing machines. Manufacturers are exploring ways to reduce energy consumption and minimize material waste during the drawing process. Some machines are now equipped with closed-loop systems that allow for the recycling of scrap metal generated during production. These systems help reduce costs and environmental impact, aligning with global trends toward more sustainable manufacturing practices.
The future of sheet metal deep drawing machines seems poised for continued evolution, driven by technological advancements in automation, machine learning, and materials science. As industries demand more complex, customized parts with higher levels of precision, deep drawing machines will continue to be a critical tool in achieving these goals. Whether it’s in the production of structural components, consumer goods, or aerospace parts, the ability to efficiently and accurately form metal into intricate shapes ensures that deep drawing remains an essential part of modern manufacturing. As the technology progresses, we can expect even more innovation in the materials, machine configurations, and integration with other manufacturing processes, ensuring that deep drawing machines meet the ever-evolving needs of global industries.
As the demand for advanced manufacturing capabilities grows, the role of Sheet Metal Deep Drawing Machines continues to evolve. The increasing complexity of designs and the need for more customized components require innovations in machine design, automation, and process control. One of the key advancements is the integration of digital twins and machine learning into the deep drawing process. A digital twin is a virtual model of the machine or the part being produced, which allows manufacturers to simulate the drawing process before the actual production begins. This simulation can predict potential issues such as material deformation, tool wear, and part defects, providing manufacturers with the ability to optimize the drawing parameters and avoid costly mistakes.
Machine learning algorithms can further improve the deep drawing process by analyzing historical data and making real-time adjustments to parameters like pressure, punch speed, and lubrication. These systems can predict potential problems, such as wrinkling or tearing, and automatically adjust the machine’s settings to prevent them. Machine learning can also be used to identify patterns in production, allowing operators to fine-tune the machine for higher efficiency and fewer defects.
Another significant trend is the use of collaborative robots (cobots) in conjunction with deep drawing machines. Cobots can assist with tasks such as loading and unloading metal sheets, positioning parts, and even monitoring the forming process. By working alongside human operators, cobots enhance the efficiency and safety of the operation, while reducing the risk of injury and increasing overall throughput. These robots can be integrated seamlessly into existing production lines, providing flexibility and scalability.
As the demand for sustainability and energy efficiency increases, deep drawing machines are being designed with more eco-friendly features. Manufacturers are looking for ways to reduce energy consumption during the drawing process, and this has led to the development of energy-efficient presses that use advanced drive systems, such as servo motors, to minimize power usage. Additionally, regenerative braking technologies, often seen in electric vehicles, are being implemented in deep drawing presses to capture and store energy during the forming cycle, which can be used during subsequent cycles, reducing overall energy consumption.
Material advancements are also playing a key role in the evolution of deep drawing machines. As new materials, such as lightweight alloys, composites, and high-strength steels, gain popularity, deep drawing machines need to adapt to handle these materials effectively. These new materials often have different properties, such as higher strength or lower ductility, which can present challenges during the forming process. Deep drawing machines are being designed with enhanced capabilities to handle a wider range of materials, and advanced tool coatings are being developed to reduce wear and tear on tools when forming harder materials.
Furthermore, multi-material parts are becoming more common in industries like automotive and aerospace, where weight reduction and performance are critical. In such cases, deep drawing machines need to be able to form different materials together without compromising the integrity of either material. This requires precision control and the ability to apply different forming forces to different parts of the metal sheet to ensure that the materials remain properly bonded and maintain their desired properties.
The ability to produce smaller and more intricate components is also becoming increasingly important, especially in industries like electronics, where precision is paramount. Advances in micro-forming and nano-manufacturing are allowing deep drawing machines to create extremely small and intricate shapes with high precision. These technologies rely on advanced control systems and specialized tooling that can operate at the microscopic level.
As the industry moves toward more customization and on-demand production, deep drawing machines are evolving to be more flexible. For example, machines that can easily switch between different parts or configurations are becoming more common. Modular designs allow manufacturers to quickly adapt to new part designs or production requirements without major changes to the overall machine setup. This flexibility is especially valuable in industries where the demand for custom parts or low-volume production is growing, such as in aerospace, medical devices, or consumer electronics.
The future of deep drawing is also likely to involve the integration of augmented reality (AR) and virtual reality (VR) for both training and real-time monitoring. AR can provide operators with real-time, on-the-job assistance, overlaying digital information such as optimal settings, machine diagnostics, and part specifications directly onto their field of view. This helps operators make better-informed decisions quickly, reducing the likelihood of errors and improving efficiency.
In terms of global supply chains, deep drawing machines are becoming more connected and integrated into the broader manufacturing ecosystem. Through cloud-based solutions, manufacturers can monitor machine performance in real-time across different locations, making it easier to optimize production schedules and share data with suppliers, distributors, and customers. This connectivity not only improves the efficiency of production but also ensures that manufacturers are able to respond more quickly to changing demands, fluctuating material costs, or unexpected production challenges.
As the focus on digitalization and smart manufacturing grows, deep drawing machines will continue to become more intelligent, connected, and responsive to the needs of modern manufacturers. The integration of big data analytics will allow manufacturers to monitor and analyze every aspect of the deep drawing process, from material behavior to machine performance. With this data, manufacturers can make more informed decisions, optimize processes, and reduce waste, contributing to both better product quality and sustainability goals.
In conclusion, sheet metal deep drawing machines are advancing rapidly, driven by innovations in automation, digitalization, sustainability, and material science. These machines are becoming more precise, flexible, and efficient, enabling manufacturers to produce complex, high-quality parts with minimal waste and maximum efficiency. As industries continue to evolve and demand more customized, lightweight, and high-performance components, deep drawing machines will remain a critical part of the manufacturing landscape, continually adapting to meet these changing needs. The future of deep drawing is bright, with continued advancements in technology making it an even more vital tool for manufacturers around the world.
Sheet Metal Warm Forming Machine
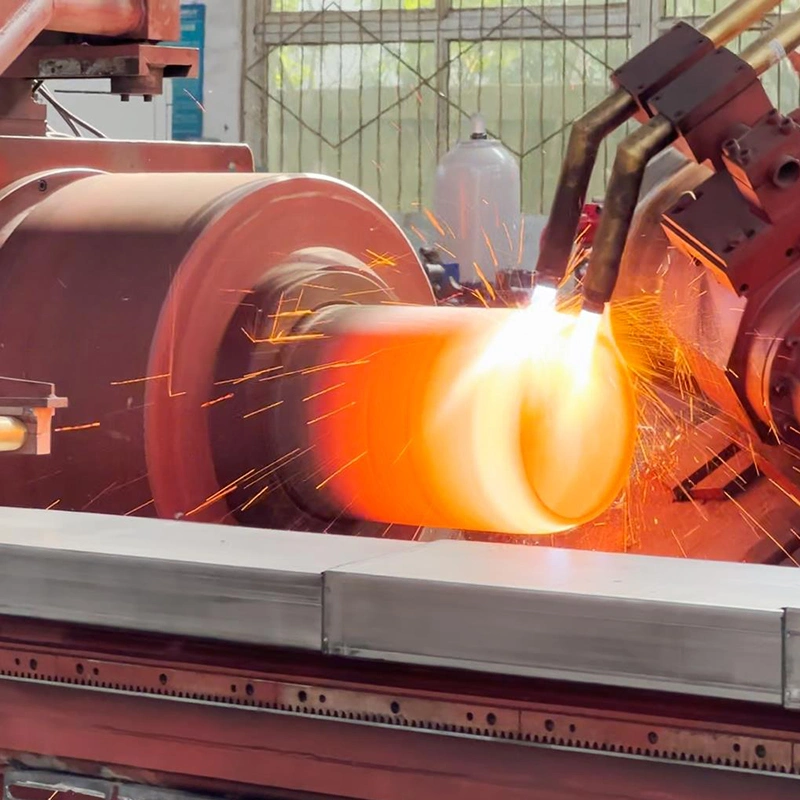
A Sheet Metal Warm Forming Machine bridges the gap between cold forming and hot forming processes, offering manufacturers a highly adaptable solution for shaping metals that are difficult to form at room temperature. Warm forming involves heating the metal to a temperature between ambient and the recrystallization point—typically between 200°C and 700°C—before subjecting it to the forming process. This temperature range enhances the metal’s ductility and reduces yield strength, allowing for more complex geometries and higher precision without the extreme temperatures or energy demands of hot forming.
Warm forming machines are particularly valuable for processing high-strength alloys such as magnesium, titanium, and advanced high-strength steels (AHSS), which can be brittle or prone to cracking in cold conditions. By applying moderate heat, these machines enable the material to stretch and flow more uniformly, resulting in deeper draws, tighter radii, and reduced risk of defects such as wrinkling or tearing. As a result, warm forming is ideal for industries where strength-to-weight ratio is crucial—such as automotive, aerospace, and defense.
Modern warm forming machines are equipped with integrated heating systems, which may include induction heating, heated dies, or radiant heating elements. These systems provide precise temperature control to ensure uniform heat distribution throughout the metal sheet. Maintaining consistent temperature is critical for achieving repeatable part quality, as variations can lead to inconsistent material behavior or formability.
In terms of mechanical design, these machines resemble hydraulic or servo-mechanical presses but with added features for thermal regulation. They typically include thermal insulation on forming tools and platens, as well as temperature monitoring sensors and feedback systems to dynamically adjust forming force and speed based on material response. Some advanced systems also feature multi-zone heating, allowing specific regions of the metal sheet to be heated to different temperatures for even greater control over material flow during forming.
Another benefit of warm forming is the reduction in required forming force compared to cold forming, which helps to extend the life of dies and tooling. The softened material flows more readily, leading to less wear and stress on components, which in turn reduces maintenance requirements and overall tooling costs. This makes warm forming not only a technically advantageous process but also a cost-effective one over time.
From a production standpoint, warm forming is also more energy-efficient than hot forming. It avoids the need for full furnace heating or high-temperature operations, resulting in faster cycle times and reduced energy consumption. This makes warm forming an appealing option for manufacturers seeking to lower their environmental footprint without sacrificing part quality or complexity.
Despite these advantages, warm forming does require careful process control. Managing the transition between heating and forming must be precisely timed to prevent the material from cooling too quickly, which would negate the benefits of the process. Similarly, parts may require post-forming treatments, such as heat treatment or surface finishing, to restore or enhance mechanical properties and surface integrity.
As manufacturing shifts toward lightweight design and high-performance materials, the relevance of warm forming machines continues to grow. They provide the necessary balance between formability and strength, making them indispensable for parts that demand both structural integrity and complex geometries. Continuous innovations—such as integration with smart sensors, automated handling systems, and predictive maintenance algorithms—are further enhancing their capabilities and efficiency.
In summary, sheet metal warm forming machines offer a versatile and efficient solution for forming challenging materials and complex shapes with improved mechanical performance. By combining the benefits of cold and hot forming, they open up new possibilities in product design and manufacturing efficiency, making them a key technology in the evolution of advanced sheet metal processing.
Warm forming technology continues to gain traction across industries as manufacturers push the limits of design complexity and performance requirements. One of the most significant advantages of warm forming machines is their ability to form parts with tighter tolerances and more intricate features than traditional cold or hot forming methods. The combination of moderate heat and controlled pressure enables the material to flow into detailed die cavities without cracking or springback, which is particularly important when precision and dimensional accuracy are essential.
As industries such as automotive and aerospace aim to reduce vehicle weight while maintaining structural safety, materials like aluminum alloys, magnesium, and ultra-high-strength steels are increasingly used. These materials, while advantageous for their lightweight properties, are notoriously difficult to form using conventional cold forming methods. Warm forming machines make these materials much more pliable without compromising their inherent strength, making it possible to produce thin-walled, complex-shaped components that contribute to lightweighting goals without sacrificing performance or durability.
The formability improvement at elevated temperatures also means fewer forming steps are required, which translates into shorter production cycles and reduced labor and tooling costs. In many cases, components that would traditionally require multiple draws or secondary processes such as annealing can now be formed in a single step using a warm forming process. This streamlining of the production line not only improves efficiency but also enhances part consistency and quality, reducing the likelihood of rejects or rework.
Another important benefit lies in the machine’s contribution to improved surface finish and part appearance. The smoother material flow at elevated temperatures allows for reduced surface friction, which in turn decreases the likelihood of galling or die marks on the part. This is particularly valuable in the consumer electronics or appliance sectors, where surface aesthetics are critical. It also means that fewer finishing operations are required after forming, which saves time and resources.
Automation plays a significant role in the effectiveness of warm forming systems. Robotic handling systems are often integrated to transfer the heated sheets quickly from the heating station to the press, minimizing heat loss and ensuring consistent forming temperatures. These robotic systems are synchronized with press cycles to ensure safety, precision, and maximum throughput. Meanwhile, sensors and smart controls monitor every aspect of the forming process in real time, including material temperature, press force, and stroke position, allowing for automatic adjustments to maintain optimal forming conditions.
To further enhance performance and process control, machine manufacturers are incorporating digital solutions such as artificial intelligence and cloud-based monitoring. These systems collect and analyze data from multiple sensors, enabling predictive maintenance and real-time process optimization. Operators can be alerted to wear patterns on tooling or minor deviations in material flow before they become critical issues, reducing downtime and increasing machine longevity.
Despite its many advantages, warm forming does present challenges, particularly in managing heat distribution and energy efficiency. Uniform heating is crucial; otherwise, uneven expansion or hard zones can develop in the material, leading to inconsistent part quality. This is why heating systems are carefully engineered, often with thermal imaging tools and closed-loop feedback systems to ensure temperature uniformity across the entire sheet surface. Modern warm forming machines address these challenges through sophisticated thermal management and active cooling features for the dies and tools, which help maintain a stable and repeatable forming environment.
As sustainability becomes a top priority in manufacturing, warm forming also supports eco-friendly initiatives by reducing energy consumption compared to hot forming processes. Because it requires less heat overall, it consumes significantly less power, and in many cases, manufacturers are exploring ways to harness waste heat or use renewable energy sources to power the heating systems. This aligns well with environmental compliance standards and corporate sustainability goals.
Looking ahead, the role of warm forming machines is expected to grow as demand increases for stronger, lighter, and more efficient components in a wide range of applications. Hybrid materials, such as metal-plastic composites or multi-layered sheets, will present new forming challenges that warm forming is well-suited to address. These developments, along with continuing advancements in machine design, heating technology, and process automation, will keep warm forming machines at the forefront of innovation in sheet metal manufacturing.
In an era where manufacturing efficiency, precision, and sustainability are equally important, warm forming machines strike a powerful balance, offering high-performance forming capabilities that meet the evolving demands of modern industry. With continuous improvements and deeper integration of intelligent technologies, these machines are becoming smarter, more adaptive, and better suited for the complex requirements of next-generation products.
The adoption of warm forming machines is also being accelerated by the increased availability of advanced simulation and modeling software. These tools allow engineers to virtually prototype the entire forming process before any physical tooling is created, dramatically reducing development time and cost. Engineers can test various materials, temperatures, die geometries, and press forces to find the optimal combination for a given part. This not only streamlines production but also enhances innovation, as designers can push the boundaries of what’s manufacturable without the risk of costly trial-and-error on the shop floor.
In practice, this means a manufacturer can design thinner, lighter, and more geometrically complex parts with confidence that they will perform under real-world conditions. For example, in the automotive industry, warm forming machines are often used to produce intricate structural components like B-pillars, floor pans, and engine cradles that must meet exacting crash safety standards while contributing to overall vehicle weight reduction. The ability to form such parts without the drawbacks of springback or tearing opens new design freedoms and enhances vehicle performance, fuel efficiency, and safety.
Warm forming is also being explored in the medical field, where devices and enclosures must meet tight tolerances, intricate geometries, and high cleanliness standards. Here, warm forming machines provide the precise control and smooth finishes required for parts like surgical instruments, orthopedic implants, or enclosures for sensitive equipment. The reduced risk of surface defects and the compatibility with materials like titanium or stainless steel make warm forming a compelling choice for critical applications where failure is not an option.
The electronics and consumer goods industries also benefit from warm forming by enabling the production of sleek, ultra-thin, and durable metal housings for smartphones, laptops, and other personal devices. These products demand both mechanical performance and visual appeal, which warm forming delivers through a combination of gentle forming pressure and uniform heating. The result is minimal deformation, tight edges, and smooth contours, even on ultra-thin materials.
There’s also a growing interest in using warm forming for post-processing in additive manufacturing. For metal parts created through 3D printing processes, warm forming can be used as a secondary step to refine shapes, enhance surface finish, or relieve internal stresses. This hybrid approach combines the geometric freedom of additive manufacturing with the material benefits of traditional metal forming, opening new doors in both prototyping and low-volume production.
Training and workforce development are evolving alongside this technology. As warm forming systems become more advanced and automated, operators and technicians must be equipped with a new skillset that includes understanding of material science, thermal behavior, and digital control systems. Many manufacturers are investing in training programs, simulation-based learning environments, and digital twins to upskill their teams. This knowledge shift ensures not only safer machine operation but also greater productivity and process optimization.
At the same time, machine builders are making warm forming equipment more user-friendly. Intuitive HMIs (Human Machine Interfaces), touchscreen controls, and AI-guided setup assistants reduce the learning curve and make it easier for operators to get up to speed. These interfaces often provide live feedback on cycle status, part conformity, and recommended adjustments, helping teams make data-informed decisions on the fly.
In terms of global trends, the growing demand for electric vehicles (EVs) is one of the most significant drivers for warm forming technology. EVs require a large number of lightweight yet strong components to offset the weight of battery packs. Warm forming makes it possible to produce these parts efficiently, especially using high-strength steels and lightweight alloys that are notoriously difficult to form cold. The process also supports the trend toward component integration—combining multiple parts into a single stamped form to reduce welds, fasteners, and assembly time.
Supply chain considerations are also shaping the evolution of warm forming. With disruptions affecting material availability and logistics, manufacturers need forming systems that are versatile and adaptable. Warm forming machines, with their ability to work across a wide range of materials and part geometries, offer a level of flexibility that helps companies respond quickly to changes in demand, material costs, or part design without the need for entirely new tooling or production lines.
Overall, the Sheet Metal Warm Forming Machine is more than just an intermediary between cold and hot forming—it’s a transformative technology enabling smarter, faster, and more capable manufacturing. As industries face increasing pressure to deliver higher-quality products with shorter lead times, tighter tolerances, and lower environmental impact, warm forming stands out as a powerful and evolving solution. Its blend of material science, process control, automation, and digital intelligence ensures it will continue to play a central role in the future of metal forming.
EMS Metalworking Machines
We design, manufacture and assembly metalworking machinery such as:
- Hydraulic transfer press
- Glass mosaic press
- Hydraulic deep drawing press
- Casting press
- Hydraulic cold forming press
- Hydroforming press
- Composite press
- Silicone rubber moulding press
- Brake pad press
- Melamine press
- SMC & BMC Press
- Labrotaroy press
- Edge cutting trimming machine
- Edge curling machine
- Trimming beading machine
- Trimming joggling machine
- Cookware production line
- Pipe bending machine
- Profile bending machine
- Bandsaw for metal
- Cylindrical welding machine
- Horizontal pres and cookware
- Kitchenware, hotelware
- Bakeware and cuttlery production machinery
as a complete line as well as an individual machine such as:
- Edge cutting trimming beading machines
- Polishing and grinding machines for pot and pans
- Hydraulic drawing presses
- Circle blanking machines
- Riveting machine
- Hole punching machines
- Press feeding machine
You can check our machinery at work at: EMS Metalworking Machinery – YouTube
Applications:
Flange-punching
Beading and ribbing
Flanging
Trimming
Curling
Lock-seaming
Ribbing