A circle cutting machine is a tool used to cut circular shapes out of various materials, such as metal, plastic, paper, or fabric. Here are some parts and features commonly found in circle-cutting machines:
- Cutting blade: The blade is the most important part of the circle cutting machine, and it can be made of different materials such as steel or tungsten carbide. The blade rotates as the machine is in use, cutting out the circular shape from the material.
- Cutting guide: The cutting guide holds the material in place and guides the blade along the cutting path. The guide is adjustable to accommodate different circle sizes.
- Cutting depth adjustment: The cutting depth adjustment allows the user to control the depth of the cut, which can be helpful when working with different materials.
- Base plate: The base plate is the surface on which the material is placed during cutting. It is typically made of a durable material like metal or plastic.
- Pivot arm: The pivot arm connects the cutting blade to the base plate and allows for precise control over the cutting angle and direction.
- Motor: The motor powers the blade and allows it to rotate quickly and efficiently.
- Safety features: Circle cutting machines may have safety features such as blade guards or automatic shut-off mechanisms to prevent injury or damage to the machine.
Circle cutting machines are used in a variety of industries, including metalworking, woodworking, and crafting. They are useful for creating circular shapes for products such as gears, wheels, and decorative items.
A circle cutting machine, also known as a circle cutter or disc cutter, is a specialized tool used to cut circular shapes from various materials, including paper, cardboard, plastic, fabric, and even thin metal sheets. These machines are commonly employed in industrial settings, craft workshops, and DIY projects.
Types of Circle Cutting Machines
Circle cutting machines come in various types, each with its own unique features and applications:
- Handheld Circle Cutters: These compact and portable machines are commonly used for cutting circles from paper, cardboard, and other lightweight materials. They typically feature a rotating blade or a punch-and-die mechanism to cut circles.
- Rotary Circle Cutters: These machines utilize a rotating blade mounted on a track or arm, allowing for precise cutting of circles in a variety of sizes. They are often used for cutting circles from fabric, leather, and other flexible materials.
- Hydraulic Circle Cutters: These powerful machines employ hydraulic pressure to cut circles from thicker materials, such as plastic sheets, thin metal sheets, and rubber. They offer high precision and cutting force for heavy-duty applications.
- CNC Circle Cutting Machines: These computer-numerically controlled (CNC) machines utilize computer programming to cut circles with exceptional precision and accuracy. They are commonly used in industrial settings for cutting circles from a wide range of materials.
Safety Precautions when using Circle Cutting Machines
When operating circle cutting machines, it is crucial to follow safety precautions to prevent injuries and ensure proper operation:
- Wear Personal Protective Equipment (PPE): Always wear safety glasses, gloves, and a cutting mat to protect yourself from sharp blades, flying debris, and potential cuts.
- Securely Clamp the Workpiece: Ensure the workpiece is firmly clamped to the machine’s work surface to prevent it from slipping or moving during cutting.
- Use the Correct Blade or Punch: Choose the appropriate blade or punch size and type for the material and desired circle size. Using the wrong blade or punch can damage the material or cause injury.
- Maintain Proper Blade or Punch Sharpness: Regularly sharpen or replace blades and punches to ensure they are sharp and maintain cutting efficiency. Dull blades can cause ragged edges and increase the risk of injury.
- Handle with Care: Always handle the machine with care, avoiding sudden movements or forceful actions that could lead to accidents.
- Never Touch the Moving Blade or Punch: Never touch the moving blade or punch while the machine is in operation.
- Turn Off the Machine Before Making Adjustments: Always turn off the machine before making any adjustments or changing the blade or punch.
- Store the Machine Properly: Store the machine in a safe, secure location when not in use, keeping it out of reach of children and unauthorized users.
By following these safety guidelines and operating the machine correctly, you can effectively utilize circle cutting machines to create precise circular shapes from various materials with minimal risk of injury.
Circle cutting machines are used in various industries, including:
- Metalworking: Circle cutting machines are commonly used in metalworking industries to cut circular shapes out of metals, such as steel, aluminum, and copper. They are used to make parts for machinery, automotive components, and other metal products.
- Woodworking: Circle cutting machines are also used in woodworking industries to cut circular shapes out of wood. They are used to make decorative wooden objects, such as clocks and signs, as well as to cut circular parts for furniture and other wooden products.
- Textile and apparel: Circle cutting machines are used in the textile and apparel industries to cut circular shapes out of fabric, leather, and other materials. They are used to make clothing, hats, bags, and other textile products.
- Craft and hobby: Circle cutting machines are popular among hobbyists and crafters, as they can be used to cut circular shapes out of a variety of materials. They are commonly used in scrapbooking, card making, and other paper crafts, as well as for creating decorative items like wreaths and ornaments.
- Manufacturing: Circle cutting machines are used in various manufacturing industries to cut circular shapes out of different materials. They are commonly used to make parts for machines and equipment, as well as for creating decorative and functional products.
In factories, where hydraulic presses manufacture deep drawn parts, as blank, sheet metal circles need to be prepared beforehand. These circles can either be manufactured by a mechanical press in serial production or needs to be cut as a square first, then the corners need to be trimmed by a machine to turn these square sheets into circles. This machine is called a circle cutting machine
A circle cutting machine is a machine that is used to cut a circle into a sheet of steel. Circle cutters are mainly used in metalworking to cut round steel sheets into circles. They come in two types: manual and automatic.
The first type of circle cutting machine is the manual type. This type of machine has large handles on both sides which are turned by hand to rotate the blade and produce the circular cut out from the metal sheet. The second type of circle cutting machine is automatic, which is much more efficient than its manual counterpart because it does not require any human input or labor to operate it.
For this purpose, we design and manufacture circle cutting machines, to cut the corners of sheet metals, which are later sent to hydraulic drawing presses for further operation
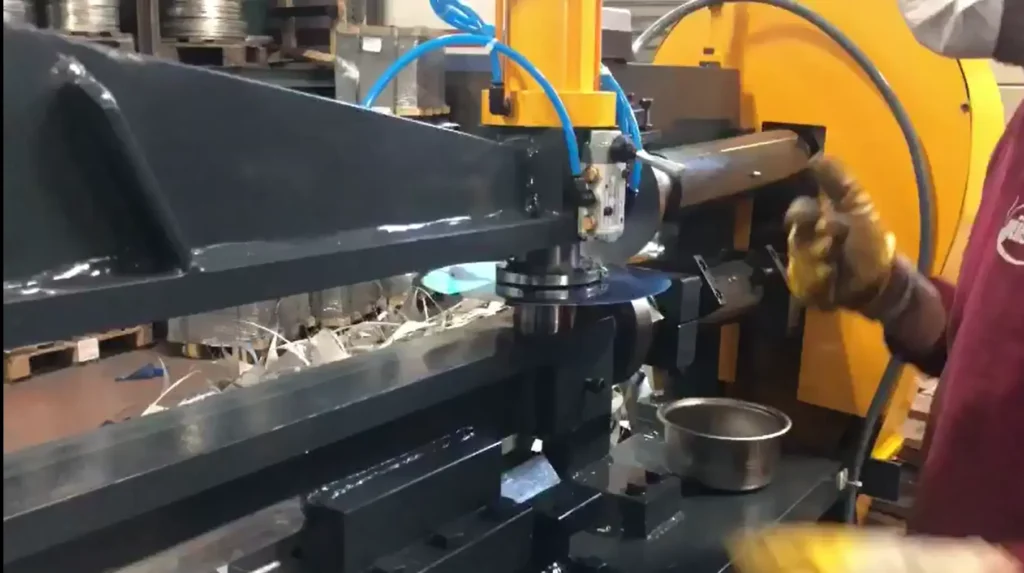
The capacity of our circle cutting machines is exceptionally high where an operator can get as high as 30 pcs per minute
The main body of the circle cutting machine is solid welded steel and knives to cut the sheet are made from high carbon high chromium steel
Circle cutting machine
Circle cutting machines can easily be used with stainless steel, aluminum, copper, and tin sheets for various industrial applications. The steel circles later are used to deep draw parts with a hydraulic press. For long-duration high-quality parts you can contact us as your supplier from our main page
For a better analysis of our circle blank cutting machine, you can check our video below
Our circle cutting machines are hand-operated. Hand-operated operations are safer as the operator needs to control the machine with his hands and he will need to keep his hands on the control board to operate the machine. Circle cutter machines are essential in production facilities where there is a deep drawing press. Deep drawing presses use round sheets to form vase forms by deep drawings.
With our round corner cutting machines or sheet metal circle cutting machines you can have a capacity as high as 1 piece in 4 seconds. With this capacity, you can feed 2 hydraulic presses with one 1 circle cutting machine easily
Circle cutting machines are versatile tools used in various industries and applications for precisely cutting circular shapes from a wide range of materials. They are commonly employed in industrial settings, craft workshops, and DIY projects.
Industrial Applications
- Manufacturing: Circle cutting machines are used in various manufacturing processes, such as cutting circular gaskets, seals, and components from rubber, plastic, and thin metal sheets. They are also used to cut circular patterns in fabrics and textiles for clothing and industrial applications.
- Packaging: Circle cutting machines are essential in the packaging industry for cutting circular packaging materials, such as cardboard discs, labels, and packaging inserts. They ensure precise dimensions and consistent quality for product packaging.
- Graphic Design and Printing: Circle cutting machines are used in graphic design and printing studios to cut circular shapes from paper, cardboard, and other materials for creating promotional materials, signage, and decorative elements.
Craft and DIY Applications
- Scrapbooking and Paper Crafts: Circle cutting machines are popular among scrapbookers and paper crafters for cutting circular embellishments, decorative shapes, and photo mats. They provide a precise and efficient way to create custom elements for scrapbooking projects.
- Jewelry Making: Circle cutting machines are used in jewelry making to cut circular shapes from leather, felt, and other materials for creating jewelry components, such as pendants, earrings, and bracelets. They ensure consistent shapes and sizes for jewelry designs.
- Home Décor and DIY Projects: Circle cutting machines are versatile tools for DIY projects, such as cutting circular patterns from fabrics for quilting, sewing, and home décor accents. They can also be used to cut circular shapes from materials like felt, foam, and cork for crafting projects.
- Model Making and Architectural Design: Circle cutting machines are used in model making and architectural design to cut circular shapes from various materials, such as cardboard, balsa wood, and foam, for creating models and prototypes. They provide precision and accuracy for detailed work.
General Safety Guidelines
When operating circle cutting machines, safety is paramount. Always follow these general guidelines:
- Wear Personal Protective Equipment (PPE): Always wear safety glasses, gloves, and a cutting mat to protect yourself from sharp blades, flying debris, and potential cuts.
- Securely Clamp the Workpiece: Ensure the workpiece is firmly clamped to the machine’s work surface to prevent it from slipping or moving during cutting.
- Use the Correct Blade or Punch: Choose the appropriate blade or punch size and type for the material and desired circle size. Using the wrong blade or punch can damage the material or cause injury.
- Maintain Proper Blade or Punch Sharpness: Regularly sharpen or replace blades and punches to ensure they are sharp and maintain cutting efficiency. Dull blades can cause ragged edges and increase the risk of injury.
- Handle with Care: Always handle the machine with care, avoiding sudden movements or forceful actions that could lead to accidents.
- Never Touch the Moving Blade or Punch: Never touch the moving blade or punch while the machine is in operation.
- Turn Off the Machine Before Making Adjustments: Always turn off the machine before making any adjustments or changing the blade or punch.
- Store the Machine Properly: Store the machine in a safe, secure location when not in use, keeping it out of reach of children and unauthorized users.
By adhering to these safety guidelines and operating the machine responsibly, you can effectively utilize circle cutting machines for various applications while minimizing the risk of injury.
Sheet Metal Circle Cutter Machine
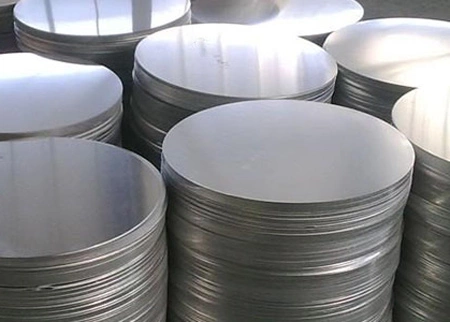
How do you cut a circle in sheet metal?
Our sheet metal circle cutter machine can cut from as small as 2″ of circle blanks on sheet metal surfaces up to 12″ in diameter. The circle diameter to be cut can be arranged on the machine. The cutting tool is made from high-carbon cutting steel specially produced for metal cutting operations. The frame of the machine is complete welded steel and all the machinery goes through stress-relieving after the manufacturing is finished. Circle cutting machines are widely used in the cookware industry.
How do you cut big circles in metal?
with our sheet metal circle cutting machine, you can cut circle blanks from square metal sheets with sheet thickness starting from 0.1 mm up to 5 mm. The diameter also can be arranged from 5 cm up to 30 cm.
The circle cutting machine is a type of machine that can cut circles out of metal sheets. It is also called an automatic circle cutter or a steel circle cutter.
It is used to cut circles out of metal sheets. The machine has a circular blade that rotates around the circumference of the sheet. The operator sets the size and radius of the desired hole and then presses start to begin cutting.
The circle cutting machine is an automatic machine that cuts circles from flat steel sheets.
The circle cutting machine is a type of metalworking equipment that is used to cut circles in sheet metal. This equipment can be used for a variety of purposes, including manufacturing and construction. The round steel sheet, which is the most common material used in the process, can be cut into circles using this machine.
EMS Metalworking Machinery: Your Trusted Partner in Precision Metalworking
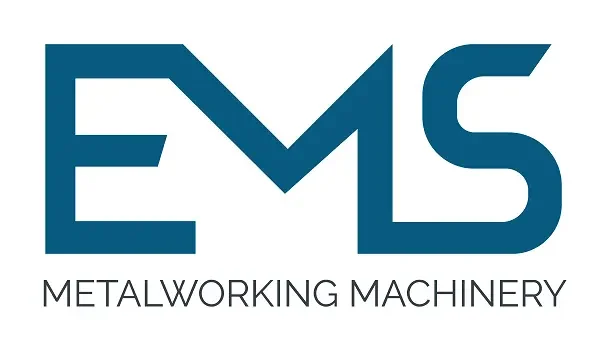
EMS Metalworking Machinery is a leading manufacturer of high-quality metalworking equipment, dedicated to providing innovative solutions that meet the evolving needs of industries worldwide. With a rich history of excellence and a commitment to technological advancement, we have earned a reputation for delivering cutting-edge machinery that ensures precision, efficiency, and durability.
Our Product Range:
- CNC Spinning Lathes: From precision bench lathes to heavy-duty industrial models, our lathes offer unmatched accuracy and performance for a wide range of applications, including machining shafts, gears, and other cylindrical components.
- Trimming Beading Machine: Our trimming beading machines are designed to provide exceptional cutting capabilities and versatility, enabling you to create complex shapes and intricate details with ease. Whether you need a horizontal or vertical trimming machine, we have the perfect solution for your needs.
- Hydraulic Deep Drawing Press Machines: Our hydraulic deep drawing press machines are built to deliver precise and powerful drawing operations, ensuring clean holes and exceptional surface finishes. We offer a comprehensive range to suit various applications.
- Grinding Machines: Our grinding machines are engineered for precision and efficiency, allowing you to achieve the highest levels of surface finish and dimensional accuracy. Whether you need a surface grinder, cylindrical grinder, or tool grinder, we have the equipment to meet your specific requirements.
- Sawing Machines: Our sawing machines are designed for fast and accurate cutting of metals, providing clean cuts and minimal burrs. From band saws to circular saws, we offer a variety of options to suit different materials and cutting needs.
- Custom Machinery: In addition to our standard product line, we also specialize in custom machinery fabrication. Our experienced engineers can work with you to design and build tailored solutions that meet your unique requirements and optimize your production processes.
Why Choose EMS Metalworking Machinery:
- Quality: Our machines are crafted with the highest quality materials and components, ensuring long-lasting performance and reliability.
- Precision: We are committed to delivering machinery that meets the most stringent tolerances and standards, ensuring exceptional accuracy in your metalworking operations.
- Innovation: We continuously invest in research and development to stay at the forefront of technological advancements, offering innovative solutions that enhance your productivity and efficiency.
- Customer Support: Our dedicated team of experts is always available to provide comprehensive support, from machine selection and installation to maintenance and troubleshooting.
- Customization: We understand that every business has unique needs, and we offer flexible customization options to tailor our machines to your specific requirements.
At EMS Metalworking Machinery, we are more than just a supplier of equipment; we are your trusted partner in metalworking success. By choosing EMS, you can be confident in the quality, reliability, and performance of your machinery, enabling you to achieve your business goals and stay ahead of the competition.
EMS Metalworking Machinery
We design, manufacture and assembly metalworking machinery such as:
- Hydraulic transfer press
- Glass mosaic press
- Hydraulic deep drawing press
- Casting press
- Hydraulic cold forming press
- Hydroforming press
- Composite press
- Silicone rubber moulding press
- Brake pad press
- Melamine press
- SMC & BMC Press
- Labrotaroy press
- Edge cutting trimming machine
- Edge curling machine
- Trimming beading machine
- Trimming joggling machine
- Cookware production line
- Pipe bending machine
- Profile bending machine
- Bandsaw for metal
- Cylindrical welding machine
- Horizontal pres and cookware
- Kitchenware, hotelware
- Bakeware and cuttlery production machinery
as a complete line as well as an individual machine such as:
- Edge cutting trimming beading machines
- Polishing and grinding machines for pot and pans
- Hydraulic drawing presses
- Circle blanking machines
- Riveting machine
- Hole punching machines
- Press feeding machine
You can check our machinery at work at: EMS Metalworking Machinery – YouTube
Applications:
- Beading and ribbing
- Flanging
- Trimming
- Curling
- Lock-seaming
- Ribbing
- Flange-punching