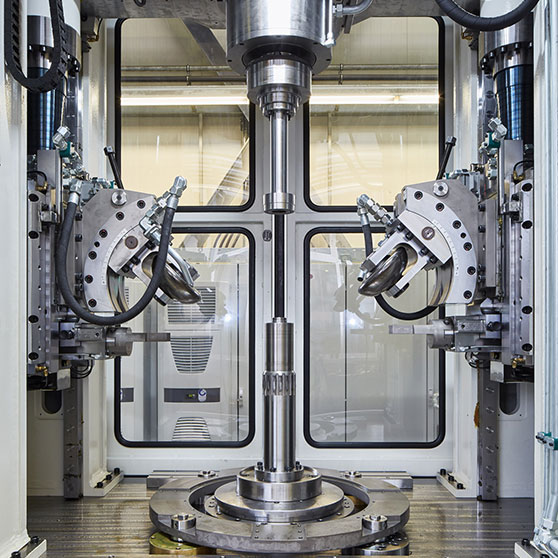
Flow Forming: Flow forming machines are advanced metal forming tools used to create high-precision, rotationally symmetrical components such as cylindrical parts, cones, and pressure vessels. The process is a variation of metal spinning and closely related to forging and extrusion, but it offers better material properties and precision.
What is Flow Forming?
Flow forming is a cold working process where a metal blank (usually a preform or tube) is clamped on a rotating mandrel. Rollers apply pressure to plastically deform the metal along the mandrel’s shape. Material flows axially and radially to form thin-walled components with high strength and tight tolerances.
Components of a Flow Forming Machine
- Mandrel – The internal support around which the material is shaped.
- Rollers (Forming tools) – Hardened rollers that press the workpiece against the mandrel.
- Main Spindle – Rotates the mandrel and the workpiece.
- Tailstock/Chuck – Holds the workpiece in place on the mandrel.
- Bed and Frame – Provide structural stability.
- Hydraulic/Servo Drive Systems – Control roller movement and pressure.
Types of Flow Forming
- Forward Flow Forming – Material flows in the same direction as the roller movement.
- Reverse Flow Forming – Material flows in the opposite direction of the rollers.
- Shear Forming (a variant) – Material thins while conforming to the mandrel’s shape.
Materials Commonly Used
- Aluminum alloys
- Stainless steel
- Titanium
- High-strength steel
- Nickel-based alloys
Applications
- Automotive (gear blanks, drive shafts)
- Aerospace (rocket motor cases, fuel tanks)
- Defense (artillery shell cases)
- Industrial (pressure vessels, hydraulic cylinders)
- Energy (wind turbine components)
Advantages
- High strength-to-weight ratio of final parts
- Excellent surface finish and dimensional accuracy
- Efficient material usage (low waste)
- Enhances mechanical properties due to cold working
- Allows forming of complex geometries
Limitations
- High equipment and tooling costs
- Limited to axisymmetric parts
- Requires skilled operators or CNC systems
- Not suitable for brittle materials
Flow forming machines are specialized metal forming systems used to produce high-precision, rotationally symmetrical components by plastically deforming a metal blank over a mandrel using one or more rollers. The process operates at room temperature (cold working) and relies on the simultaneous rotation of the workpiece and axial movement of the rollers to elongate and shape the material while reducing its wall thickness. The setup typically includes a mandrel (which defines the internal geometry), forming rollers (which apply pressure), a spindle (to rotate the mandrel and workpiece), and a machine frame with drive systems to control movement and force.
There are two main types of flow forming based on the direction of material flow relative to the roller movement: forward flow forming and reverse flow forming. In forward flow forming, the material flows in the same direction as the roller travel, whereas in reverse flow forming, the material is displaced in the opposite direction. A related technique, shear forming, involves thinning the wall of the part without significantly changing its axial length, and it’s often used for making cones or hemispherical shapes.
Flow forming is particularly effective for creating components that demand a high strength-to-weight ratio, precise wall thickness control, and excellent surface finish. The cold working of the material during forming improves its mechanical properties, including tensile strength and fatigue resistance. Materials suitable for flow forming include aluminum, stainless steel, titanium, high-strength steels, and nickel-based alloys. These attributes make the process ideal for manufacturing critical components in aerospace (such as rocket motor cases and satellite housings), automotive (like wheel rims and transmission parts), defense (ammunition shells), and energy sectors (such as turbine parts and gas cylinders).
The main advantages of flow forming include high material efficiency due to minimal waste, excellent dimensional accuracy, and the ability to form complex shapes with tight tolerances. However, it does have limitations: it’s best suited to axisymmetric parts, requires expensive equipment and tooling, and generally involves high setup times and operator expertise or CNC control for consistent quality. Despite these challenges, its ability to produce lightweight yet durable components makes flow forming an indispensable process in high-performance manufacturing.
Compared to other metal forming techniques, flow forming stands out due to its ability to combine material efficiency with enhanced mechanical properties. Unlike forging, which can require multiple heating and forming steps, or machining, which often involves high material waste, flow forming achieves near-net-shape parts with minimal waste and without the need for heating. This not only conserves material but also reduces secondary processing time. In contrast to metal spinning—which is more suited for forming shallower or less complex shapes—flow forming allows for precise control over wall thickness and elongation, making it more suitable for structural components that must endure internal pressure or stress.
Tooling in flow forming is both critical and costly. The mandrel must be precisely machined and heat-treated to endure high forces and repeated cycles, and forming rollers must be made from hardened tool steels or carbides to resist wear. Tool geometry, such as the roller nose radius and attack angle, significantly affects the material flow and surface finish. Toolpath programming and motion control, often handled by CNC systems, determine the final part’s geometry and quality. Any deviation in alignment, pressure, or feed rate can cause surface defects, cracks, or thickness variations, which are unacceptable in critical applications like aerospace.
Flow forming also enables the production of multi-diameter or stepped geometries in a single setup. By adjusting the roller position and path in successive passes, a single tube can be transformed into a component with varied diameters and wall thicknesses, such as a rocket nozzle with an integral neck and skirt. This capability eliminates the need for welding or joining multiple components, which would otherwise introduce weak points or require post-processing.
In terms of quality assurance, modern flow forming machines are equipped with force sensors, position encoders, and sometimes acoustic or thermal monitoring systems to detect anomalies in real-time. These integrations help ensure consistent forming conditions and reduce the risk of defects. Post-forming inspections typically involve ultrasonic thickness testing, surface roughness measurements, and sometimes destructive testing for validating microstructure and strength.
The flow forming industry continues to evolve with improvements in automation, simulation software, and materials science. Advanced finite element analysis (FEA) models are now used to simulate forming conditions and predict outcomes before physical trials, saving both time and cost. Meanwhile, developments in alloy design and lubrication have broadened the range of materials that can be flow formed reliably.
Overall, flow forming machines offer unmatched capability for producing strong, lightweight, and highly accurate cylindrical parts. While the capital investment and process complexity are significant, the resulting product performance and material savings justify their use in industries where quality, weight, and strength are paramount.
Vertical Flow Forming Machines
Vertical flow forming machines are a specific type of flow forming system where the forming axis is oriented vertically rather than horizontally. This configuration offers unique advantages in terms of gravity-assisted material control, reduced machine footprint, and ease of handling heavy or large-diameter preforms. These machines are particularly suited for forming long, tubular, or deep cylindrical parts that require high precision and structural integrity.
In a vertical flow forming setup, the mandrel is mounted vertically, and the workpiece (typically a cylindrical blank or tube) is loaded over it from the top or bottom. The forming rollers are arranged around the workpiece and move vertically to reduce wall thickness and elongate the material along the axis of the mandrel. As with horizontal machines, the workpiece rotates at controlled speeds, and the rollers apply radial pressure to deform the metal plastically while maintaining dimensional accuracy.
One of the key advantages of vertical orientation is the support offered by gravity. The part’s own weight helps maintain concentricity and alignment on the mandrel, especially during the initial forming stages. This is particularly beneficial when forming deep-drawn components like gas cylinders, pressure vessels, and aerospace liners. The vertical layout also simplifies the automation of material loading and unloading, often through the use of robotic arms or overhead cranes, which are easier to integrate in a vertical system.
In terms of design, vertical flow forming machines typically include a stationary or rotating mandrel (depending on the process requirements), a turret or carriage system to hold the forming rollers, and a robust vertical frame structure to withstand axial and radial forming forces. Multi-roller systems (usually 2 or 3 rollers) are common, allowing for balanced forming pressure and improved surface quality. The machine’s control system synchronizes spindle rotation, roller feed rates, and axial travel to ensure precise material flow.
Applications of vertical flow forming machines span across industries that require high-strength, lightweight, and seamless cylindrical components. Common products include aerospace engine liners, artillery shell casings, gas storage cylinders, automotive shock absorber tubes, and certain types of hydraulic components. The ability to form parts with varying wall thicknesses, stepped profiles, or integrated flanges makes vertical flow forming highly versatile.
Limitations of vertical flow forming machines are similar to those of their horizontal counterparts—primarily the restriction to axisymmetric shapes and the need for specialized tooling and setup. However, in environments where floor space is constrained, or where the parts being formed are long and heavy, the vertical design offers practical and ergonomic advantages that improve overall efficiency and process stability.
As with other advanced forming processes, vertical flow forming benefits from real-time process monitoring, closed-loop control systems, and simulation tools that help optimize roller paths, predict material behavior, and ensure part quality. In high-volume or high-specification industries, these machines offer an excellent combination of strength, precision, and material efficiency.
Vertical flow forming machines, by virtue of their upright orientation, are especially advantageous when forming deep or elongated components where gravity can assist in stabilizing the part on the mandrel. This is particularly useful during the initial forming passes when the material might otherwise shift or sag in a horizontal setup. The vertical configuration allows for more natural alignment of the workpiece, reducing the risk of runout or misalignment, which can lead to dimensional inaccuracies or surface defects. Because of this, vertical machines are often preferred for high-precision parts that require consistent wall thickness and roundness over long axial lengths.
The forming operation on a vertical flow forming machine typically begins with the loading of a cylindrical preform or tube onto a vertically oriented mandrel, either from the top or bottom, depending on the specific design. Once the workpiece is secured, the mandrel begins to rotate while the rollers gradually engage with the surface. Through carefully programmed axial movement, the rollers apply a controlled radial force, causing the metal to plastically deform and flow along the contour of the mandrel. The process is often performed in multiple passes, with the rollers progressively reducing the wall thickness and elongating the part without introducing defects like wrinkling or tearing.
Tooling and setup for vertical flow forming must be precisely engineered. The mandrel not only defines the internal geometry of the finished part but also must withstand significant radial forces and frictional heat generated during forming. In most high-performance applications, mandrels are made from hardened steel or tool steel alloys and are sometimes water-cooled to maintain thermal stability. Forming rollers, likewise, are made from wear-resistant materials and are designed with specific geometries that control the metal flow—parameters such as roller nose radius, attack angle, and surface finish all influence the forming result. Vertical machines may feature two, three, or even more rollers to distribute force evenly and achieve better surface finishes and tighter tolerances.
Automation plays a critical role in vertical flow forming, particularly in industrial settings where cycle time, consistency, and safety are key concerns. Robotic arms or gantry systems are often used for loading heavy preforms, while sensor systems monitor roller force, mandrel torque, and workpiece temperature. These real-time data streams are integrated into CNC control systems that can adjust roller feed rate, spindle speed, and position dynamically to compensate for material variations or thermal expansion. This level of process control ensures repeatability and helps meet the tight specifications demanded in sectors like aerospace and defense.
Material selection is important in flow forming, and vertical machines are often tasked with forming hard-to-work metals like titanium, Inconel, or ultra-high-strength steels. These materials benefit significantly from the cold working effect of flow forming, which improves mechanical properties such as yield strength, fatigue resistance, and structural integrity. Unlike casting or machining, which may leave internal defects or stress concentrations, flow forming produces a seamless, work-hardened structure with excellent grain orientation along the direction of material flow. This makes flow-formed parts not only stronger but also more reliable in pressure-bearing or fatigue-prone applications.
Despite the advantages, vertical flow forming machines do require substantial capital investment and specialized maintenance. The vertical arrangement demands a rigid, vibration-resistant structure capable of supporting heavy axial loads and precise roller movement. The height of the machine can also be a limiting factor in certain facilities, requiring high ceilings and special handling equipment. However, for operations focused on producing high-performance cylindrical components with minimal material waste and maximum strength, vertical flow forming offers a highly effective solution that often outperforms conventional machining or fabrication methods in terms of both cost-efficiency and part quality
Hydraulic Flow Forming Equipment
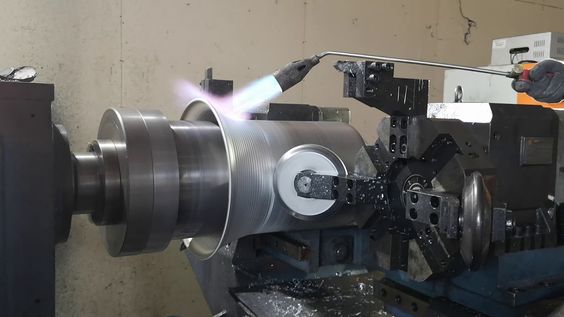
Hydraulic flow forming equipment utilizes hydraulic systems to drive the motion and force of the forming rollers, mandrel support, and auxiliary movements involved in the flow forming process. Unlike mechanically or electrically driven systems, hydraulic machines offer superior control over forming forces, smooth and consistent roller feed, and the ability to handle larger deformation loads. This makes hydraulic flow forming machines ideal for forming thick-walled components, high-strength alloys, and large-diameter parts that require high forming forces and precision.
In a typical hydraulic flow forming setup, the machine consists of a bed structure that supports a rotating spindle or mandrel, a set of hydraulic cylinders that actuate the rollers, and a hydraulic power unit (HPU) that supplies pressurized fluid to the system. The rollers are mounted on slideways or arms and are driven by hydraulic cylinders that allow precise control of radial position, feed rate, and forming pressure. The use of hydraulics enables a smooth, non-pulsing application of force, which is crucial when forming materials that are prone to cracking or require uniform metal flow.
Hydraulic control systems are often integrated with CNC or PLC-based controllers that regulate the position and velocity of the rollers, the rotational speed of the mandrel, and the axial feed during the forming passes. This integration allows for highly repeatable and programmable forming cycles, accommodating complex geometries such as stepped diameters or tapered wall thicknesses. Hydraulic machines are also well-suited for forming long or heavy components, as the system can be designed with large capacities and robust structural stiffness to handle significant forming loads without deformation or vibration.
One of the main advantages of hydraulic flow forming equipment is its ability to apply very high forming forces with fine resolution. This is especially important when working with hard metals such as Inconel, titanium, or hardened steel, which require substantial pressure to deform plastically. The hydraulic system can maintain consistent pressure even when the material’s resistance changes during forming, ensuring uniform wall thickness and surface finish. Additionally, hydraulic systems can be adjusted to provide different forming characteristics for various materials and part designs by tuning pressure levels, flow rates, and cylinder stroke speeds.
In terms of applications, hydraulic flow forming machines are widely used in industries that require large, strong, and high-precision cylindrical components. These include aerospace components like rocket motor cases and satellite housings, defense products such as artillery shells and missile bodies, industrial items like pressure vessels and gas cylinders, and automotive parts such as transmission sleeves or structural tubes. The cold working effect introduced by the hydraulic forming process significantly enhances the material’s mechanical properties, providing improved fatigue resistance and strength without adding weight.
However, hydraulic flow forming equipment also comes with some considerations. Hydraulic systems require regular maintenance, including checking for fluid leaks, monitoring filter conditions, and managing system pressure to prevent wear or failure. They can be less energy-efficient than servo or mechanical alternatives, particularly in low-force applications, and the hydraulic fluid itself introduces the need for environmental and safety precautions. Despite these factors, the power and precision offered by hydraulic systems make them a preferred choice for demanding forming operations where mechanical or servo drives might not provide the necessary force or smooth control.
Overall, hydraulic flow forming equipment combines high force capability with precise process control, making it a robust solution for forming advanced materials and complex cylindrical geometries in critical industrial sectors.
Hydraulic flow forming equipment offers a unique blend of high force capacity and smooth controllability that makes it particularly well-suited for forming tough materials and heavy-walled components. The use of hydraulic actuators to control the forming rollers allows for a consistent application of pressure throughout the forming process, which is essential when working with metals that have variable resistance or require gradual, progressive deformation to avoid cracking or thinning irregularities. The hydraulic system’s ability to precisely modulate roller force and feed rate ensures that the metal flows uniformly over the mandrel, resulting in a component with consistent wall thickness, excellent concentricity, and high surface integrity.
The machine’s hydraulic power unit typically includes a reservoir, pump, control valves, pressure sensors, and a cooling system. These components work together to maintain system pressure and ensure that each forming stroke operates within the predefined load parameters. The pressure can be continuously monitored and adjusted in real time, which gives operators the flexibility to fine-tune the forming process based on the behavior of the material, especially during the transition between passes or when forming stepped or contoured parts. This adaptability is critical when dealing with alloys that harden quickly under cold working, such as titanium or high-nickel superalloys, as it allows the forming force to be increased incrementally as the material becomes more resistant.
Hydraulic flow forming machines are often capable of both forward and reverse flow forming, depending on the motion direction of the rollers and the mandrel configuration. The ability to switch between these modes on a single machine adds versatility and expands the range of component geometries that can be manufactured without changing equipment. Moreover, the hydraulic drive can be configured to operate multiple rollers simultaneously, maintaining symmetrical force distribution and minimizing part distortion. This is especially beneficial when forming long parts that might otherwise deflect or vibrate under asymmetrical loads.
From a production standpoint, hydraulic flow forming systems can be automated to a high degree, reducing operator involvement and improving consistency. Components like servo-hydraulic valves, proportional pressure controllers, and position sensors can be integrated with CNC or PLC systems to create a closed-loop feedback environment. This not only allows for highly repeatable part quality but also enables real-time diagnostics, process optimization, and advanced features like adaptive force control. In some advanced systems, load cells are embedded into the roller arms to provide continuous feedback on forming pressure, while position encoders ensure accurate roller tracking along the workpiece’s axial length.
The structural design of hydraulic flow forming machines is another critical aspect. Because they must handle high radial and axial forces, their frames are built from reinforced steel or castings with vibration-damping properties to ensure dimensional stability. The bed must be rigid enough to resist deflection under full load, and all guideways and roller supports must be precisely machined and aligned to prevent roller tilt or skew. Mandrels are often modular and replaceable to allow quick changes between part designs or material sizes, which increases productivity and flexibility.
Despite their many strengths, hydraulic flow forming machines require careful consideration in terms of maintenance and operating environment. Hydraulic systems must be kept clean and leak-free, as contamination can cause valve failures, reduced response time, and inconsistent forming force. Temperature control of the hydraulic fluid is also important, as overheating can lead to changes in viscosity that affect system performance. Some systems incorporate heat exchangers or chillers to maintain fluid temperature within an optimal range, particularly during extended production runs where heat buildup is inevitable.
Overall, the key advantage of hydraulic flow forming equipment lies in its ability to deliver high, controllable forming forces in a smooth and predictable manner. This capability makes it an indispensable technology in industries where material strength, dimensional accuracy, and structural integrity are critical. As demand grows for stronger and lighter components—particularly in aerospace, defense, and energy—hydraulic flow forming remains a leading method for producing seamless, high-performance cylindrical parts that meet stringent engineering requirements.
As industries continue to push the boundaries of performance, efficiency, and reliability, the role of hydraulic flow forming equipment becomes even more crucial. With increasing demand for lightweight yet high-strength components, especially in aerospace and defense sectors, manufacturers are leaning heavily on flow forming processes to meet strict specifications for part integrity and material utilization. Hydraulic systems, with their high load capacity and precise control, allow for the consistent production of these components while minimizing material waste and post-processing requirements.
The forming process also produces a work-hardened structure, improving fatigue life and resistance to pressure-induced failures. In aerospace, this translates to lighter structures that retain strength under extreme conditions; in defense, it means safer, more durable components like gun barrels, missile casings, and pressure vessels. Automotive and energy sectors benefit too—driveshafts, suspension parts, hydrogen storage tanks, and even turbine casings can all be flow formed with reduced wall thickness and increased material strength, improving fuel efficiency and reliability.
Another area where hydraulic flow forming excels is in forming components with variable wall thicknesses or complex step-down geometries. These shapes are difficult to machine economically and would otherwise require welding or joining, which introduces stress points or inconsistencies. The precise roller control offered by hydraulic actuators allows smooth transitions along the workpiece’s length, maintaining structural continuity and avoiding microfractures or dimensional errors that would otherwise lead to failure in service. For example, forming a stepped motor case with different diameters and thickness zones in a single piece eliminates the need for assembly and strengthens the part overall.
Advances in control systems and digital integration are also enhancing the capabilities of hydraulic flow forming. Modern machines often come equipped with touchscreen interfaces, recipe management systems, and remote diagnostics. The ability to store and recall forming profiles means that complex parts can be produced with minimal manual input once the process has been qualified. This improves consistency and throughput while reducing operator error. Some manufacturers are integrating simulation tools and digital twins into their workflow, allowing the process to be modeled, tested, and optimized virtually before any material is processed—saving time, material, and wear on tooling.
In terms of sustainability, hydraulic flow forming aligns well with the growing focus on energy-efficient and low-waste manufacturing. Because the process works through plastic deformation without removing material, there is little to no scrap generated, especially when starting with forged or extruded blanks. The cold forming nature of the process means less energy is used compared to hot forming, and the improved mechanical properties of the final product can reduce the amount of material needed for a given performance requirement. Even in high-volume production, where cycle times and machine efficiency are critical, hydraulic systems have proven adaptable and durable enough to maintain high uptime with proper maintenance.
To support continuous improvement, manufacturers often incorporate process monitoring tools such as roller force sensors, torque monitoring on the mandrel, acoustic emission sensors, and thermal imaging to detect variations in real time. These inputs can trigger alarms or adjust parameters to compensate for unexpected conditions such as material hardness variation or roller slippage. Over time, data collected from these systems also supports predictive maintenance strategies, minimizing downtime and extending the life of critical components like rollers and mandrels.
Despite their robust capabilities, hydraulic flow forming machines are typically large, heavy-duty systems requiring reinforced floors, noise suppression measures, and proper safety systems. Operators must be trained not only in machine operation but also in hydraulic system management, including pressure regulation, oil cleanliness, and system diagnostics. Safety features like pressure relief valves, emergency shutoff systems, and guarding around the forming area are essential to ensure safe operation, especially when forming high-strength materials at high pressures.
In summary, hydraulic flow forming equipment stands as one of the most effective solutions for producing seamless, high-integrity cylindrical components with excellent dimensional control, material efficiency, and mechanical performance. Its capacity to form difficult materials into complex geometries under tightly controlled conditions makes it indispensable in advanced manufacturing, particularly where performance and reliability cannot be compromised. As control systems, materials, and forming technologies continue to evolve, hydraulic flow forming will remain a foundational process in the production of high-performance components across critical industries.
CNC Flow Forming Machines
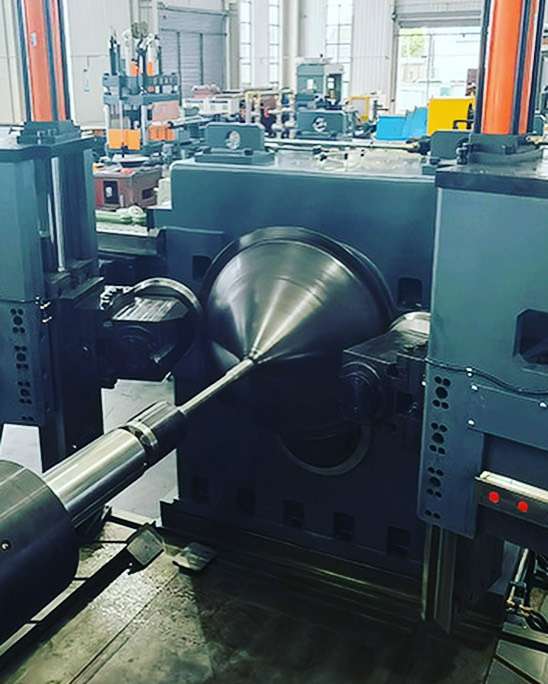
CNC flow forming machines integrate computer numerical control (CNC) systems with the traditional flow forming process to enable precise, automated, and repeatable metal forming of cylindrical parts. These machines use digitally programmed commands to control all aspects of the process—roller position, feed rate, mandrel rotation, spindle speed, and even real-time feedback from sensors. The result is a highly flexible and accurate system capable of producing complex geometries with consistent quality, minimal manual intervention, and reduced scrap.
At the core of a CNC flow forming machine is a multi-axis control system, typically managing 3 to 6 axes of motion. These axes include the rotation of the mandrel (spindle), the radial and axial movements of the rollers, and sometimes angular or tilting motions depending on the machine configuration. Each motion is coordinated precisely through a CNC controller, which executes a pre-programmed toolpath defined in G-code or a proprietary software format. This level of control allows manufacturers to produce parts with intricate features, such as varying wall thicknesses, stepped diameters, or smooth tapers, all while maintaining tight tolerances.
The roller movement is servo-driven, often using high-torque motors coupled with ball screws or linear actuators to ensure smooth and accurate positioning. The forming force applied by each roller can be monitored and adjusted in real-time based on sensor feedback, ensuring optimal forming pressure throughout the operation. CNC machines are especially effective for high-strength or sensitive materials, as they can fine-tune the deformation process to avoid issues like cracking, tearing, or surface imperfections.
CNC flow forming is used to manufacture a wide range of components across industries, including rocket motor cases, missile bodies, aerospace liners, automotive shafts, energy storage tanks, and precision tubes. These parts benefit not only from the superior strength-to-weight ratios offered by the cold working process but also from the geometric consistency that CNC control provides. For high-volume production, CNC machines ensure that every part meets the exact same specification without deviation, eliminating variability and reducing inspection requirements.
Tooling for CNC flow forming machines includes programmable roller carriages, interchangeable mandrels, and sometimes live tooling that can be adjusted between forming cycles. The mandrels can be heated, cooled, or even vibrated to assist in material flow and improve final part quality. Machine designs may be horizontal or vertical, with vertical CNC machines often preferred for longer parts or when space efficiency is important. Modern systems may also include quick-change tooling features to speed up part transitions in a production setting.
One of the major advantages of CNC flow forming is its compatibility with simulation and design software. Engineers can model the forming process using finite element analysis (FEA) and then generate the CNC code directly from these simulations. This allows virtual testing and optimization before any physical material is processed, reducing development time and cost. Some advanced CNC systems include digital twin technology, where the physical and virtual machines are synchronized in real time to simulate, monitor, and optimize performance continuously.
The software ecosystem of CNC flow forming machines includes user-friendly HMIs (Human-Machine Interfaces), which allow operators to set parameters, monitor process data, and troubleshoot issues intuitively. These systems often include alarm handling, error logs, diagnostics, and even remote access for support and updates. The CNC controller ensures process repeatability by logging each operation, making it easy to validate part history for industries where traceability is crucial.
In terms of maintenance and reliability, CNC flow forming machines benefit from self-diagnostic features and modular designs that simplify service and component replacement. Regular calibration routines are built into the control software to ensure that positioning remains accurate even after extended use. Sensors and encoders continuously monitor positional feedback and roller pressure, enabling predictive maintenance strategies that prevent unexpected downtime.
As manufacturing continues to move toward digitalization and smart factories, CNC flow forming machines represent a critical step in combining precision metal forming with data-driven control. Their ability to form complex, high-performance parts with minimal waste and high repeatability makes them indispensable in modern production environments. With ongoing developments in CNC software, machine learning integration, and material science, CNC flow forming will continue to evolve as a cornerstone technology for advanced, high-specification cylindrical manufacturing.
CNC flow forming machines are particularly valued for their ability to seamlessly integrate precision with automation. In industries where part repeatability, traceability, and minimal deviation are non-negotiable, these machines provide a level of control that manual or semi-automated systems cannot match. The consistency they offer is critical not just for the integrity of the formed part, but also for maintaining downstream assembly tolerances and eliminating the need for extensive quality control intervention. Because the CNC controller regulates every aspect of the forming cycle, from the initial positioning of the preform to the final forming pass, the outcome is a dimensionally accurate part with optimized material distribution.
The programming flexibility of CNC flow forming also allows for easy adaptation to design changes. Instead of reconfiguring hardware or tooling, the operator can simply modify the digital toolpath or input a new forming recipe, which greatly reduces changeover time and enhances responsiveness to design iteration or customization. This is particularly advantageous in aerospace or defense sectors, where component design may evolve rapidly and production must keep pace with new specifications without costly delays. Even complex geometries such as variable thickness profiles, deep internal contours, or blended transitions can be executed by simply adjusting the CNC code and roller paths.
Thermal effects during forming, though less pronounced than in hot forming, are still monitored and compensated for by CNC systems. For example, sensors may track mandrel and workpiece temperatures to detect heat buildup that could affect dimensional accuracy or material flow behavior. The CNC system can then dynamically adjust roller feed rates, dwell times, or rotational speeds to maintain consistency. In many modern machines, these adjustments are part of a closed-loop control system that ensures the forming conditions remain within the desired envelope throughout the process, minimizing material springback and ensuring tight control over final dimensions.
In high-end CNC flow forming setups, data acquisition and analytics are deeply integrated into the forming process. Parameters such as forming forces, roller positions, motor torque, and vibration levels are continuously logged and can be analyzed to detect trends, predict wear on tooling, or refine the forming strategy. These systems may also include adaptive algorithms that learn from previous cycles to optimize performance in subsequent runs, reducing cycle time and improving surface quality. With this data-centric approach, operators can not only ensure quality but also improve process economics by extending tool life and reducing rework.
Another strength of CNC flow forming machines is their ability to minimize material waste. Since the process is fundamentally a plastic deformation operation that reshapes rather than removes metal, it avoids the inefficiencies of traditional subtractive machining. When CNC control is applied, this material efficiency is taken to an even higher level. The forming program can be finely tuned to apply only the necessary deformation to achieve the desired shape, ensuring that no excess material is used and no unnecessary thinning occurs. This is especially important in expensive materials like titanium or Inconel, where every gram saved directly reduces cost.
CNC systems also simplify the documentation and certification process. In regulated industries, where compliance with quality standards like AS9100 or ISO 9001 is mandatory, the ability to provide detailed process records—including timestamps, force logs, and machine status reports—is a major advantage. These digital records serve as traceable proof of conformance and can be easily archived or reviewed in case of audits or failure analysis. This traceability not only adds confidence to the end-user but also streamlines production qualification and product lifecycle management.
Operator training is simplified by the interface-driven nature of CNC systems. Instead of requiring deep mechanical knowledge, operators can be trained to manage the machine through visual dashboards, guided setup routines, and simulation previews. The risk of human error is reduced significantly because once a part program is qualified, the machine will consistently reproduce it without the need for manual intervention. Some systems even allow offline programming and simulation, so new parts can be prepared and verified without interrupting ongoing production.
CNC flow forming machines also provide scalability. A single platform can handle a wide variety of part types and sizes, simply by changing the mandrel and adjusting the program. Whether producing short prototype runs or scaling up to high-volume production, the same machine can adapt without major retooling. This versatility, combined with the repeatability and accuracy of CNC control, makes these machines central to lean manufacturing strategies and flexible production cells where uptime and changeover speed are critical metrics.
Ultimately, CNC flow forming represents the convergence of mechanical forming expertise with modern control and automation technologies. It preserves the inherent strengths of the flow forming process—namely high strength-to-weight ratios, seamless construction, and efficient material usage—while enhancing its precision, versatility, and integration into digital manufacturing ecosystems. As part complexity grows and tolerance demands tighten, CNC flow forming machines offer a future-ready solution capable of delivering exceptional performance with minimal waste and high operational efficiency.
Building further on their capabilities, CNC flow forming machines are increasingly being integrated into smart factory environments as part of Industry 4.0 initiatives. The connectivity of these machines through IoT (Internet of Things) protocols allows real-time data exchange with enterprise resource planning (ERP) systems, quality management software, and supply chain platforms. This integration facilitates seamless scheduling, predictive maintenance, and quality assurance workflows, thereby reducing downtime and improving overall equipment effectiveness (OEE). Operators and engineers can monitor machine health, production status, and quality metrics remotely, enabling faster response to issues and better decision-making.
Advanced CNC flow forming systems also benefit from enhanced sensor arrays that detect not only force and position but also surface finish and microstructural changes during forming. Optical and laser-based measurement systems can be incorporated to inspect parts in-process, identifying defects or deviations before they become costly. The feedback from these inline inspections can trigger automatic process adjustments or alert operators to intervene, further ensuring that parts meet stringent specifications without the need for extensive post-process inspection or rework.
Another trend in CNC flow forming is the adoption of hybrid manufacturing approaches, where flow forming is combined with additive or subtractive processes. For instance, a part may be flow formed to near-net shape and then finished by CNC machining or laser additive processes to achieve complex features or internal geometries that are difficult to form alone. CNC control systems facilitate these hybrid workflows by coordinating toolpaths and machine motions across different operations, enhancing overall manufacturing flexibility and enabling highly customized part designs.
In terms of material science, CNC flow forming machines are increasingly used with advanced alloys and composites that challenge traditional manufacturing methods. For example, forming titanium alloys or nickel-based superalloys requires extremely precise force control and gradual deformation steps to prevent cracking or residual stresses. The programmability of CNC machines enables tailored forming cycles that optimize these parameters for each material, extending the range of components that can be economically produced by flow forming. Similarly, CNC control helps in working with layered or clad materials, ensuring uniform deformation without delamination or defects.
Training and workforce development are evolving alongside CNC technology. Virtual reality (VR) and augmented reality (AR) tools are being developed to help operators simulate and visualize flow forming processes, understand machine dynamics, and practice troubleshooting without risk. These immersive training platforms complement traditional instruction and accelerate proficiency, particularly as machines grow more sophisticated and software-driven.
As sustainability becomes a key consideration, CNC flow forming machines also contribute by enabling more efficient use of raw materials and reducing energy consumption. The precise control of forming parameters reduces scrap rates and minimizes energy-intensive secondary operations. Moreover, advances in servo motors and energy recovery systems integrated with CNC controls improve overall machine efficiency, aligning manufacturing with environmental goals.
In summary, CNC flow forming machines represent a highly adaptable, precise, and intelligent manufacturing solution. Their combination of digital control, automation, and real-time feedback delivers parts with superior mechanical properties and dimensional accuracy, while also supporting modern production demands for flexibility, traceability, and sustainability. With ongoing advancements in control software, sensor integration, and material processing, CNC flow forming will continue to expand its role as a critical technology in producing next-generation cylindrical components across aerospace, defense, energy, automotive, and beyond.
Custom Flow Forming Machine Solutions
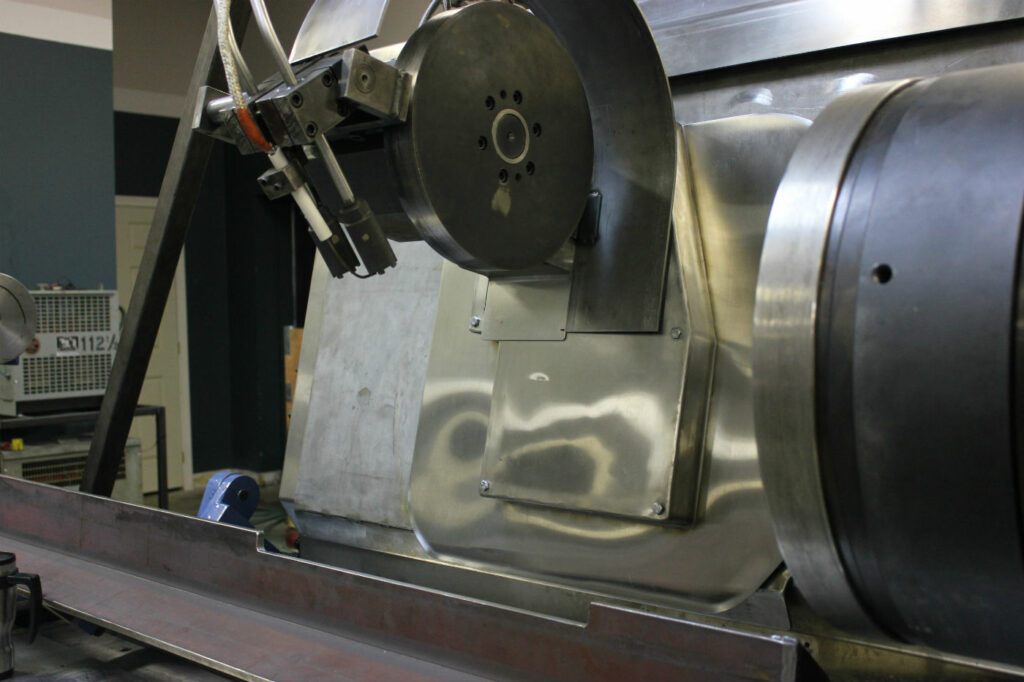
Custom flow forming machine solutions are specialized systems designed and built to meet the unique requirements of specific manufacturing applications or industries. Unlike standard, off-the-shelf flow forming machines, custom solutions focus on tailoring every aspect of the machine—from structural design, drive systems, control software, and tooling—to accommodate particular part geometries, material types, production volumes, and process complexities.
The need for customization arises when standard machines cannot efficiently handle unusual part dimensions, complex shapes, exotic materials, or demanding tolerances. For example, very large-diameter components, extremely long parts, or workpieces with multiple steps, grooves, or variable wall thicknesses often require machines with bespoke mandrels, roller configurations, and frame sizes. Custom machines can also integrate specialized features such as multiple roller stations, hybrid forming technologies, or combined processes like flow forming with spinning or forging, all designed to optimize the manufacturing workflow for specific products.
One of the key elements in custom flow forming machine design is the structural engineering of the frame and support systems. The machine must withstand significant forming forces without deformation or vibration, so materials selection, frame geometry, and reinforcement strategies are carefully calculated using finite element analysis (FEA) and other simulation tools. This ensures machine rigidity and precision, which are critical for achieving consistent part quality and maintaining tight tolerances during high-force forming operations.
Drive and actuation systems in custom machines are often designed for higher capacities or unique motion profiles. This might involve combining hydraulic, servo-electric, or hybrid drives to deliver the required torque, speed, and positional accuracy. For instance, a machine intended to work with very high-strength alloys might use a heavy-duty hydraulic system for roller actuation alongside CNC-controlled servo drives for precise mandrel rotation and axial feed. These combinations enable both power and fine control in demanding applications.
Control software in custom solutions is another area where flexibility is paramount. Since the machine may have non-standard axes or additional process steps, software is often developed or adapted to accommodate specific kinematics and process sequences. Integration with customer systems—such as enterprise manufacturing execution systems (MES), quality management platforms, or proprietary data analytics tools—is common, enabling seamless workflow integration and real-time process monitoring tailored to the customer’s operational environment.
Custom tooling is a critical part of the solution. Mandrels, rollers, and backup supports are designed for the exact shape and size of the parts, sometimes incorporating quick-change or modular designs to accommodate different product variants. Tool materials and surface treatments are selected to match the workpiece material and process conditions, reducing wear and extending tool life. In some cases, tooling may be designed to incorporate cooling channels, vibration dampening features, or embedded sensors to enhance process control and part quality.
For manufacturers with special needs—such as producing aerospace-grade rocket motor casings, defense components with complex geometries, or large-scale energy storage vessels—custom flow forming machines enable production that would otherwise be impossible or prohibitively expensive. These machines can also incorporate advanced features like automated loading and unloading systems, integrated inspection stations, or robotic material handling to support lean manufacturing and reduce cycle times.
Engineering support and collaboration are essential components of custom flow forming solutions. Machine builders typically work closely with customers from the initial concept through design, prototyping, testing, and commissioning. This partnership ensures that the final machine meets performance goals, safety requirements, and production targets. Additionally, training and documentation are tailored to the customer’s workforce to maximize machine utilization and minimize downtime.
Ultimately, custom flow forming machines provide manufacturers with the ability to push the boundaries of what can be produced economically and at scale. By optimizing machine design, control, and tooling for the unique demands of each application, these tailored solutions deliver superior part quality, material efficiency, and process reliability—key factors in maintaining competitive advantage in demanding industries.
Custom flow forming machine solutions often extend beyond the core forming process to include integrated systems that address the entire production workflow. This might involve automation for loading raw materials and unloading finished parts, robotic handling for precision positioning, and inline quality inspection systems such as laser scanners or ultrasonic testers. By incorporating these elements, manufacturers can create a highly efficient production cell that reduces human error, shortens cycle times, and ensures consistent part quality throughout long production runs.
The flexibility of custom machines also allows adaptation to evolving product requirements. As customers develop new designs or materials, the machine can be modified or upgraded with minimal disruption. Modular components such as interchangeable mandrels, adjustable roller arms, and configurable control software mean that the investment in a custom flow forming system remains relevant over a longer period. This adaptability is particularly valuable in industries like aerospace or defense, where product lifecycles and specifications frequently change.
From a materials standpoint, custom solutions are engineered to handle a wide range of metals, including challenging alloys like titanium, Inconel, stainless steel, and aluminum. Specialized forming strategies, combined with tailored tooling and force control, ensure that these materials are formed without compromising their mechanical properties or surface integrity. For instance, custom machines can include temperature-controlled mandrels or rollers, enabling cold or warm forming processes that optimize the metal’s ductility and reduce residual stresses.
Safety and ergonomics are also integral to custom machine design. Large-scale flow forming machines operate under high forces and require careful safeguarding of moving parts and hydraulic systems. Custom enclosures, interlocks, emergency stop systems, and noise reduction features are incorporated to protect operators and maintain compliance with industrial safety standards. Additionally, ergonomic considerations such as operator interfaces, lighting, and access points are tailored to facilitate easy operation and maintenance, improving productivity and reducing fatigue.
The collaboration between machine builders and end-users during the design and commissioning phases ensures that the custom flow forming system fits seamlessly into existing manufacturing environments. This includes considerations for floor space, power requirements, environmental controls, and integration with upstream and downstream equipment. Detailed planning minimizes installation time and ensures smooth ramp-up to full production capacity.
In terms of after-sales support, custom machine providers often offer comprehensive service packages including training, preventative maintenance, remote diagnostics, and rapid response for repairs or upgrades. Given the specialized nature of these machines, ongoing technical support is critical to maintaining optimal performance and minimizing downtime. Some providers also develop customized spare parts inventories and consumables management programs to streamline supply chain logistics for their customers.
The ROI on custom flow forming machines can be significant, particularly when they enable production of parts that were previously too costly, complex, or impossible to manufacture. By reducing the need for secondary machining, welding, or assembly, these machines not only lower manufacturing costs but also improve part reliability and performance. For companies competing in high-tech sectors, the ability to produce advanced components with superior mechanical properties and tight tolerances can be a decisive competitive advantage.
In summary, custom flow forming machine solutions provide tailored, end-to-end manufacturing platforms that address specific production challenges through advanced engineering, automation, and integration. Their design flexibility, material adaptability, and process precision enable manufacturers to innovate and scale efficiently while maintaining high quality and operational reliability. As product demands evolve and new materials emerge, these customized systems will continue to play a vital role in pushing the capabilities of flow forming technology across diverse industries.
Beyond the core benefits of precision and adaptability, custom flow forming machine solutions often incorporate advanced data management and digitalization features to align with modern manufacturing paradigms. Integration with Manufacturing Execution Systems (MES) and Enterprise Resource Planning (ERP) platforms enables seamless coordination of production schedules, inventory management, and quality control. This digital connectivity allows real-time monitoring of machine performance, process parameters, and production output, enabling manufacturers to optimize operations and respond swiftly to any deviations or issues.
Furthermore, the use of advanced analytics and machine learning algorithms on collected process data opens new avenues for process optimization and predictive maintenance. By analyzing trends in force feedback, temperature fluctuations, or vibration patterns, manufacturers can anticipate wear on critical components such as rollers or mandrels, schedule maintenance proactively, and avoid unexpected downtime. Over time, these insights help refine forming parameters, improve product quality, and reduce operating costs.
Custom flow forming machines are also increasingly designed with sustainability in mind. Energy-efficient drive systems, such as regenerative servo motors and variable frequency drives, reduce power consumption during forming cycles. Hydraulic systems may incorporate energy recovery and low-leakage technologies, lowering operational costs and environmental impact. Additionally, by maximizing material utilization and minimizing scrap through precise deformation control, these machines contribute to waste reduction and support circular economy initiatives.
As global supply chains grow more complex, custom flow forming solutions can include features that facilitate traceability and compliance with stringent industry standards. Embedded sensors and digital records create an unbroken chain of custody for each part, documenting material batch numbers, process parameters, and quality inspections. This traceability is vital in sectors like aerospace, defense, and medical devices, where regulatory compliance and certification are mandatory.
Innovation in tooling technology is another hallmark of custom systems. Advanced materials such as ceramics, carbides, or surface-coated steels extend tool life under high-pressure, high-temperature conditions. Tooling can be engineered with conformal cooling channels or vibration dampening layers to improve process stability and surface finish. Smart tooling embedded with sensors provides direct feedback on contact forces and wear, enabling real-time adjustments and proactive maintenance.
The human-machine interface (HMI) in custom flow forming machines is tailored for intuitive operation, with graphical displays, touchscreen controls, and multi-language support. Customizable dashboards show live process data, alarms, and diagnostics to operators and engineers alike, facilitating quick troubleshooting and reducing the learning curve. Some systems incorporate augmented reality (AR) guidance for maintenance or setup, enhancing operator efficiency and safety.
In addition, custom flow forming machines are often designed to accommodate future upgrades and expansions. Modular architectures allow the addition of extra roller stations, automated material handling systems, or integrated inspection modules without major redesign. This scalability ensures that the investment remains viable as production demands grow or evolve.
Ultimately, custom flow forming machine solutions embody a synthesis of mechanical engineering excellence, advanced control technology, and tailored automation. They empower manufacturers to push the limits of cylindrical component design and production, delivering parts that meet the most demanding specifications while optimizing cost, quality, and throughput. As industries continue to innovate, these custom machines will remain a cornerstone of precision metal forming technology, enabling the creation of lighter, stronger, and more complex components essential for modern engineering challenges.
High-Performance Flow Forming Systems
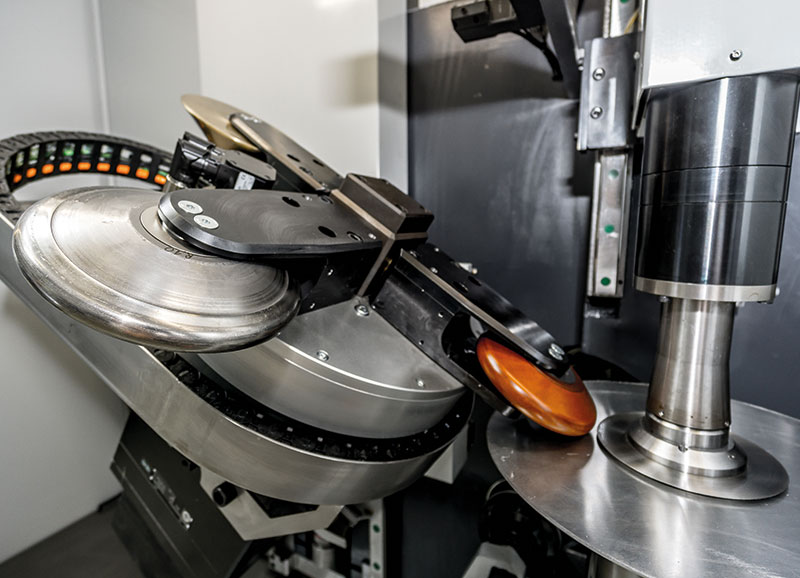
High-performance flow forming systems are advanced manufacturing platforms engineered to deliver exceptional precision, speed, and reliability for producing complex cylindrical components with demanding specifications. These systems leverage state-of-the-art mechanical design, robust drive technologies, and sophisticated control architectures to push the limits of flow forming capability, enabling manufacturers to meet stringent requirements in aerospace, automotive, defense, energy, and other high-tech industries.
At the core of high-performance flow forming systems is a rigid and vibration-damped machine structure designed to withstand intense forming forces without compromising dimensional accuracy or surface quality. The frames are typically constructed from high-strength steel or composite materials, reinforced and optimized using finite element analysis (FEA) to ensure stability during high-speed, high-force operations. This rigidity minimizes deflection and chatter, which are critical factors in achieving tight tolerances and superior surface finishes on formed parts.
Drive and actuation components in these systems are carefully selected for power, responsiveness, and precision. High-torque servo motors, hydraulic cylinders with fast response valves, or hybrid servo-hydraulic drives provide the necessary force and control to deform even the toughest alloys such as titanium, Inconel, and hardened steels. Advanced feedback devices, including high-resolution encoders and force sensors, feed data to the control system, enabling closed-loop control of roller position, feed rates, mandrel speed, and forming pressure in real time.
The control systems themselves are typically CNC-based with multi-axis coordination, allowing complex toolpaths and dynamic process adjustments. Sophisticated software algorithms manage deformation rates, compensate for material springback, and optimize roller engagement profiles to maximize material properties and minimize defects. High-performance systems may also incorporate adaptive controls that respond to sensor inputs during forming, adjusting parameters on-the-fly to maintain process stability and part quality.
Tooling in high-performance flow forming systems is engineered for durability, precision, and adaptability. Rollers and mandrels are made from premium materials and undergo advanced surface treatments such as nitriding, chrome plating, or diamond-like coatings to resist wear and reduce friction. Tool geometries are optimized using simulation tools to balance forming forces and material flow, often customized for specific part designs. Quick-change tooling systems enable fast transitions between different products, supporting flexible production schedules.
Thermal management is another key aspect. Because forming operations can generate heat that affects material properties and dimensional accuracy, high-performance systems incorporate cooling mechanisms such as water-cooled mandrels, thermoelectric cooling, or oil-based systems to maintain optimal temperatures. This thermal control preserves metallurgical integrity and reduces residual stresses, which is especially important when working with heat-sensitive or high-strength alloys.
In addition to the mechanical and control enhancements, high-performance flow forming systems often integrate advanced process monitoring and quality assurance technologies. Inline sensors measure forming forces, vibrations, temperature, and surface finish, while non-contact laser scanners or ultrasonic probes can inspect dimensions and detect defects in real time. These data streams enable immediate corrective actions or automatic process adjustments, drastically reducing scrap rates and enhancing first-pass yield.
Automation features complement these systems by incorporating robotic material handling, automated loading and unloading, and integration with factory-wide manufacturing execution systems (MES). Such automation not only increases throughput but also improves safety and reduces operator fatigue. The seamless flow of information across the production line facilitates predictive maintenance and continuous process improvement.
High-performance flow forming systems are well-suited for producing critical components such as aerospace engine casings, defense missile bodies, automotive drive shafts, energy storage vessels, and medical implants. Their ability to form complex geometries with superior mechanical properties and minimal material waste translates into lighter, stronger parts that meet or exceed industry standards.
Ultimately, these systems represent a fusion of cutting-edge mechanical engineering, advanced control technology, and integrated automation designed to maximize productivity, quality, and flexibility. As material science advances and design complexity grows, high-performance flow forming systems will continue to evolve, enabling manufacturers to tackle increasingly challenging applications with confidence and efficiency.
High-performance flow forming systems continue to advance through the integration of emerging technologies and innovative engineering approaches that further enhance their capabilities. One significant area of development is the incorporation of real-time digital twins—virtual replicas of the physical machine and process—that simulate and predict forming behavior under varying conditions. By continuously comparing the digital twin’s output with live sensor data, operators can detect anomalies early, optimize process parameters, and reduce trial-and-error adjustments. This predictive capability accelerates product development cycles and improves production consistency.
Material-specific forming strategies are another critical evolution. Advanced systems use detailed material models that consider factors like anisotropy, strain hardening, and temperature-dependent behavior to tailor the forming path for each alloy or composite. This level of customization ensures that the metal flows optimally, preserving structural integrity and achieving the desired microstructure and mechanical properties. For example, forming high-strength aerospace alloys may require slower feed rates and multiple passes, while aluminum alloys can be processed at higher speeds with different roller geometries.
The push towards sustainability also shapes high-performance flow forming system design. Energy-efficient components, such as servo drives with regenerative braking and low-friction linear guides, reduce power consumption. Systems are designed to minimize coolant use and maximize recycling, while precision forming reduces scrap and the need for secondary machining. Manufacturers increasingly prioritize machines that comply with environmental standards, helping organizations reduce their carbon footprint without sacrificing performance.
Customization remains a cornerstone, as many high-performance flow forming systems are purpose-built for specific applications. This customization extends beyond the mechanical and control aspects to include bespoke user interfaces that present process data in intuitive formats tailored to operator roles. For example, a machine operator might view simplified dashboards emphasizing immediate control functions, while engineers and quality managers access detailed analytics and trend reports. These interfaces facilitate better communication and decision-making across production teams.
The expansion of Industry 4.0 connectivity further enhances these systems’ role in smart manufacturing ecosystems. Integration with cloud platforms enables centralized monitoring and data analytics across multiple machines and sites. This connectivity supports remote diagnostics and troubleshooting, reducing downtime and service costs. In some cases, artificial intelligence algorithms analyze aggregated data to recommend preventive maintenance schedules or process improvements, pushing the boundaries of autonomous manufacturing.
In terms of hardware, the use of advanced materials in machine components, such as ceramic bearings or carbon fiber structural elements, improves longevity and reduces maintenance requirements. Innovations in sensor technology, including fiber optic strain gauges and high-speed vision systems, provide more accurate and comprehensive monitoring of the forming process. These sensors can detect minute variations in part geometry or machine behavior that traditional instruments might miss, enabling finer control and higher quality outcomes.
High-performance flow forming systems also increasingly support hybrid manufacturing processes. For example, some machines are equipped to combine flow forming with laser cladding or additive manufacturing techniques, allowing the creation of parts with enhanced surface properties or integrated features not achievable through forming alone. This hybrid approach expands design freedom and functional integration, meeting the complex demands of modern engineering components.
Operator safety and ergonomic design remain priorities. Modern systems incorporate advanced guarding, light curtains, and interlocks that comply with the latest safety standards. Ergonomic considerations include adjustable control consoles, improved access for maintenance, and features that reduce noise and vibration exposure. These improvements not only protect workers but also contribute to sustained productivity and job satisfaction.
Ultimately, high-performance flow forming systems embody a holistic approach to precision manufacturing. By combining robust mechanical design, intelligent control, advanced monitoring, and seamless integration into digital factory environments, these systems enable manufacturers to achieve unparalleled levels of efficiency, quality, and flexibility. As industries continue to demand lighter, stronger, and more complex components, high-performance flow forming will remain a vital technology—constantly evolving to meet the challenges of tomorrow’s manufacturing landscape.
Building on these advancements, the future trajectory of high-performance flow forming systems is shaped by continuous innovation aimed at further enhancing process efficiency, precision, and adaptability. One emerging trend is the development of fully autonomous flow forming cells, where machines operate with minimal human intervention through intelligent automation and AI-driven process control. Such cells integrate advanced robotics for material handling, real-time adaptive controls for process optimization, and comprehensive in-line inspection systems that collectively enable “lights-out” manufacturing. This shift not only increases throughput and reduces labor costs but also ensures consistent, repeatable quality essential for high-value applications.
Material innovation also plays a significant role in shaping next-generation flow forming capabilities. As novel alloys and metal matrix composites become more prevalent, flow forming systems will increasingly incorporate specialized tooling and process controls tailored to these new materials. For example, forming ultra-high-strength steels or titanium aluminides may require dynamic adjustment of roller forces and temperatures to prevent cracking or distortion. Advanced sensor fusion combining thermal, force, and acoustic emissions data will allow these systems to detect subtle signs of material stress and adapt in real time, preserving part integrity.
The rise of digital manufacturing ecosystems further enhances flow forming processes by linking machines, materials, and designs in an interconnected workflow. Digital threads that span from product conception through production and quality assurance enable unprecedented traceability and data-driven decision-making. High-performance flow forming systems integrated within such ecosystems can leverage historical process data to inform design for manufacturability, optimize toolpaths before physical trials, and ensure compliance with regulatory standards through comprehensive documentation.
Sustainability considerations will increasingly influence the design and operation of flow forming systems. Efforts to minimize energy consumption, water use, and waste generation are driving the adoption of green manufacturing technologies. This includes the use of alternative lubricants and coolants, energy recovery systems that capture and reuse heat generated during forming, and modular machine designs that facilitate easy upgrades and refurbishment rather than full replacement. These measures help manufacturers meet environmental goals without compromising production capability.
Customization and scalability will remain critical, with manufacturers demanding machines that can evolve alongside changing product portfolios and market demands. Modular architectures that allow quick reconfiguration of roller stations, mandrel sizes, and control systems enable manufacturers to switch rapidly between different product families or introduce new features without major capital investment. This flexibility is particularly valuable in industries like aerospace and defense, where low-volume, high-complexity components require adaptable manufacturing platforms.
Operator interfaces and training will continue to benefit from immersive technologies such as augmented reality (AR) and virtual reality (VR). These tools provide intuitive visualizations of machine operations, step-by-step maintenance guidance, and simulation-based training environments that improve workforce competency and reduce errors. Coupled with AI-driven virtual assistants, operators can receive real-time support and troubleshooting advice, enhancing machine uptime and process reliability.
Finally, the globalization of supply chains and increasing regulatory scrutiny are driving demand for integrated quality assurance and compliance features. High-performance flow forming systems will incorporate comprehensive inspection and validation technologies that capture detailed process data and link it to specific parts and batches. This capability ensures that each component meets exacting standards and supports certification requirements, particularly in safety-critical sectors like aerospace, medical, and energy.
In essence, the ongoing evolution of high-performance flow forming systems reflects a convergence of mechanical innovation, digital intelligence, and sustainable manufacturing principles. These systems are positioned to meet the growing complexity and precision demands of modern industries, delivering parts that are lighter, stronger, and more reliable while optimizing cost and environmental impact. As manufacturers continue to embrace these technologies, flow forming will remain a cornerstone process enabling the next generation of advanced engineered components.
Flow Forming Machines for Precision Metal Forming
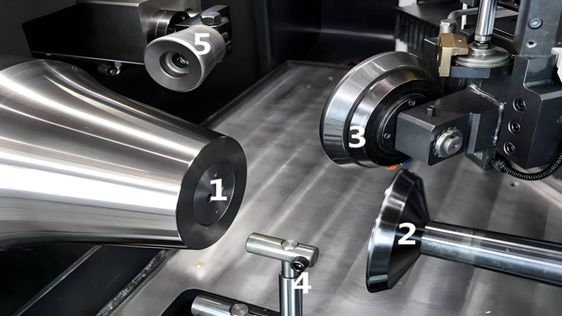
Flow forming machines for precision metal forming are specialized systems designed to produce thin-walled, high-strength cylindrical components with exceptional dimensional accuracy and surface finish. These machines use a combination of rotary motion and controlled deformation through rollers to reduce the thickness of a metal workpiece while extending its length and refining its geometry. The result is parts that meet tight tolerances and superior mechanical properties, making flow forming an ideal process for industries such as aerospace, automotive, defense, and medical device manufacturing.
At the heart of these machines is a robust, precisely engineered frame that provides the necessary rigidity and vibration damping to maintain stability during high-force forming operations. This structural integrity ensures that deformation forces are transmitted consistently, avoiding part distortion or surface defects. The machine typically includes a rotating mandrel around which the metal blank is mounted and a set of rollers that apply controlled pressure to the workpiece as it spins.
Precision control systems play a critical role in achieving the exacting standards required for these applications. Modern flow forming machines employ CNC technology to regulate mandrel rotation speed, axial feed, roller position, and pressure with high resolution and repeatability. Feedback from sensors measuring force, displacement, and vibration feeds into closed-loop control algorithms that adjust parameters in real time to compensate for material variations or process disturbances.
Tooling is carefully designed and manufactured to match the specific part geometry and material characteristics. Mandrels are precision-machined to provide a stable, accurately contoured surface against which the metal is formed, while rollers are shaped and positioned to ensure uniform deformation without inducing defects such as wrinkling or cracking. Advanced surface treatments and coatings extend tooling life and maintain consistent friction conditions during forming.
Thermal management is often integrated into precision flow forming machines to maintain optimal process temperatures and prevent adverse metallurgical changes. Cooling channels within the mandrel or rollers, or external temperature control systems, help dissipate heat generated during deformation, preserving material properties and dimensional stability.
To support quality assurance, many machines incorporate in-process monitoring and inspection technologies. Non-contact measurement systems such as laser scanners or ultrasonic probes can verify part dimensions and detect surface flaws during forming, enabling immediate corrective actions. This integration reduces scrap rates and ensures consistent part quality, vital for critical applications.
Automation features, including robotic loading and unloading, further enhance precision by minimizing manual handling and ensuring repeatable positioning of workpieces. When integrated with factory information systems, these machines contribute to streamlined production workflows and data-driven process optimization.
Flow forming machines for precision metal forming are capable of handling a wide range of materials, from aluminum and stainless steel to high-strength alloys like titanium and Inconel. Their ability to produce thin-walled components with improved mechanical properties—such as increased strength-to-weight ratios and enhanced fatigue resistance—makes them indispensable in manufacturing lightweight yet durable parts.
In summary, precision flow forming machines combine advanced mechanical design, sophisticated control systems, specialized tooling, and integrated quality monitoring to deliver superior metal forming performance. They enable manufacturers to produce complex, high-quality cylindrical components efficiently and consistently, meeting the stringent demands of today’s high-tech industries.
These precision flow forming machines are engineered to provide exceptional flexibility, accommodating a wide variety of part sizes and shapes while maintaining tight tolerances. The ability to adjust roller angles, pressures, and feed rates allows the forming process to be finely tuned for each specific material and design requirement. This adaptability is critical when producing parts with complex geometries or variable wall thicknesses, as it ensures uniform material flow and prevents defects such as thinning or cracking.
Material handling systems integrated into these machines contribute significantly to overall process accuracy and efficiency. Automated loading and unloading reduce the risk of human error and ensure consistent placement of blanks on the mandrel. Coupled with quick-change tooling systems, this facilitates rapid production changeovers, essential for meeting the demands of just-in-time manufacturing and small batch runs common in aerospace and medical industries.
The control systems in precision flow forming machines often feature advanced user interfaces that allow operators to monitor and adjust forming parameters easily. Real-time graphical displays of force curves, roller positions, and mandrel speed provide immediate feedback on process conditions. Additionally, programmable recipes enable the storage and recall of optimized settings for different parts or materials, promoting repeatability and reducing setup times.
To further enhance quality, many machines employ adaptive control technologies that respond dynamically to variations in material properties or external disturbances. Sensors measure forming forces and displacements continuously, and the control system adjusts roller pressure or feed rate in real time to maintain consistent deformation. This level of precision helps minimize scrap, improve yield, and extend tooling life by avoiding excessive loading or uncontrolled strain.
The design of tooling components, including mandrels and rollers, is critical to the success of the precision flow forming process. Mandrels are manufactured with extremely tight dimensional tolerances and finished to smooth surface finishes to support accurate metal flow and final part geometry. Rollers are engineered to apply consistent pressure over the workpiece surface and are often coated or treated to reduce friction and wear. Tooling can be customized to accommodate complex profiles such as stepped diameters, flanges, or tapered sections.
Thermal management in precision flow forming machines ensures that temperature fluctuations do not negatively impact material behavior or dimensional stability. Cooling channels within the mandrel and rollers, along with temperature sensors, allow the system to maintain an optimal thermal environment. This control is especially important when forming heat-sensitive alloys or when multiple passes are required, as uncontrolled heat buildup can lead to metallurgical defects or dimensional inaccuracies.
Quality assurance measures are tightly integrated into the forming process. Inline inspection tools, such as laser micrometers or ultrasonic thickness gauges, verify dimensions and detect surface imperfections in real time. Data from these systems feed into process control, enabling immediate corrective action and detailed traceability for each part. This capability is vital for applications with strict regulatory requirements, such as aerospace and medical device manufacturing.
Overall, flow forming machines for precision metal forming combine mechanical robustness, advanced automation, precise control, and integrated quality monitoring to deliver parts that meet demanding engineering specifications. Their versatility, efficiency, and accuracy make them indispensable for producing lightweight, high-strength components that drive innovation across numerous industries. As manufacturing technology continues to evolve, these machines will remain at the forefront of precision metal forming solutions.
In addition to their core capabilities, precision flow forming machines increasingly incorporate digital connectivity and data analytics to enhance process insight and control. By linking machine sensors, control systems, and inspection devices to centralized manufacturing execution systems (MES) or industrial IoT platforms, manufacturers gain real-time visibility into production status, machine health, and quality metrics. This connectivity supports predictive maintenance strategies that reduce unplanned downtime by forecasting wear or component failure before it occurs, based on trends in vibration, force, or temperature data.
Data-driven optimization is another key benefit. Historical forming data can be analyzed using machine learning algorithms to identify subtle correlations between process parameters and final part quality. These insights enable continuous improvement of forming recipes, reducing cycle times and scrap rates while ensuring parts consistently meet specifications. Over time, this creates a closed-loop manufacturing environment where process knowledge accumulates and evolves automatically.
The integration of advanced simulation tools also plays a vital role in precision flow forming. Finite element analysis (FEA) models simulate metal flow, stress distribution, and temperature effects during forming, allowing engineers to validate and refine tooling and process parameters before physical trials. This virtual prototyping reduces development costs and shortens lead times, accelerating time to market for new components.
Moreover, customization options for precision flow forming machines continue to expand. Manufacturers can specify modular designs that accommodate different roller configurations, mandrel sizes, or automation packages tailored to their unique production needs. Some systems offer multi-station setups enabling simultaneous forming operations or sequential processing steps within a single machine footprint, further boosting throughput and flexibility.
Sustainability is also gaining prominence in the design and operation of these machines. Energy-efficient drives and hydraulic systems minimize power consumption, while advanced lubrication and cooling solutions reduce waste and environmental impact. By maximizing material utilization and minimizing rework or scrap, flow forming contributes to lean manufacturing principles and supports corporate sustainability goals.
Training and support infrastructure are evolving alongside the machines themselves. Virtual and augmented reality tools provide immersive training experiences that help operators understand complex machine functions and maintenance procedures without the risks or costs associated with live equipment. Remote support capabilities enable experts to troubleshoot issues or guide repairs in real time, minimizing downtime and enhancing operational reliability.
In critical industries such as aerospace, medical devices, and defense, traceability and compliance are paramount. Precision flow forming machines support these requirements by embedding digital process documentation and quality data directly into production records. This comprehensive traceability ensures each component’s history is fully documented, facilitating certification, audits, and regulatory compliance.
In summary, precision flow forming machines represent a sophisticated convergence of mechanical engineering, digital technology, and process expertise. Their ability to consistently produce high-quality, complex cylindrical components with superior mechanical properties makes them indispensable in advanced manufacturing sectors. As technology continues to advance, these machines will further integrate intelligence, connectivity, and sustainability, driving innovation and efficiency across a broad spectrum of industrial applications.
Seamless Tube Production via Flow Forming
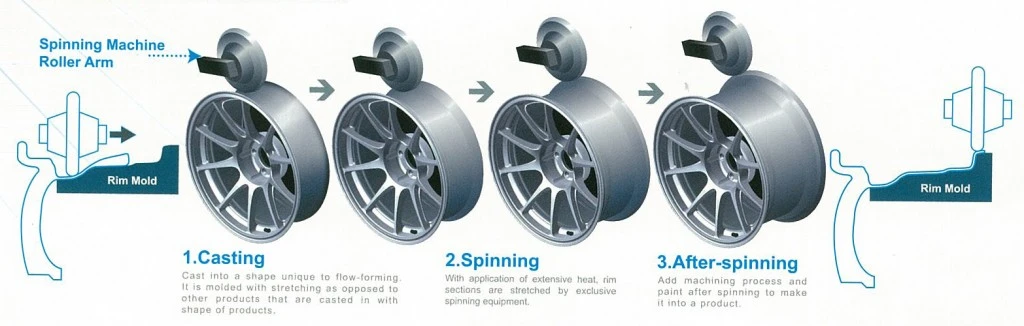
Seamless tube production via flow forming is a highly efficient and precise manufacturing process used to create thin-walled, high-strength cylindrical tubes without any welded seams. This method enhances the mechanical properties and surface quality of tubes compared to welded counterparts, making it ideal for demanding applications in industries such as aerospace, automotive, oil and gas, and medical devices.
The process begins with a solid metal blank, often a thick-walled cylindrical billet or preform, which is mounted onto a rotating mandrel inside the flow forming machine. As the mandrel spins at controlled speeds, one or more rollers apply pressure to the exterior surface of the billet, progressively reducing its wall thickness while extending its length. The metal is plastically deformed and flows over the mandrel’s contour, resulting in a seamless tube with precise dimensions and superior metallurgical properties.
One of the key advantages of flow forming for seamless tube production is the ability to produce tubes with varying wall thicknesses and complex profiles in a single continuous operation. By adjusting roller positions, pressures, and feed rates, manufacturers can create tubes with tapered sections, stepped diameters, or integrated features such as flanges and collars, reducing or eliminating the need for subsequent machining or assembly.
The seamless nature of the tubes produced by flow forming ensures excellent structural integrity and resistance to pressure, fatigue, and corrosion. The process refines the grain structure of the metal through controlled plastic deformation, improving mechanical strength and toughness. These enhanced properties make flow-formed seamless tubes particularly valuable in high-stress environments, such as hydraulic cylinders, aircraft landing gear, and medical implants.
Precision control systems are essential for maintaining consistent tube quality during flow forming. CNC controls regulate mandrel rotation speed, roller feed rate, and forming pressure to achieve tight tolerances on diameter, roundness, and wall thickness. Real-time feedback from force sensors and displacement encoders enables closed-loop control, compensating for material variability or external disturbances to ensure uniform deformation.
Material versatility is another strength of flow forming in seamless tube production. The process accommodates a wide range of metals, including aluminum alloys, stainless steel, titanium, and high-strength steels. This flexibility allows manufacturers to tailor tubes for specific performance requirements, balancing factors such as weight, corrosion resistance, and strength.
Thermal management during flow forming is critical, as the deformation generates heat that can affect material properties. Cooling systems integrated into the mandrel or roller assemblies maintain stable temperatures, preventing overheating and preserving metallurgical integrity. Controlled temperature conditions also reduce residual stresses and minimize dimensional distortions in the finished tubes.
Flow forming machines designed for seamless tube production often incorporate automated material handling and inline inspection systems. Robotic loaders and unloaders improve process efficiency and reduce handling errors, while laser measurement devices and ultrasonic testers monitor tube dimensions and surface quality in real time. This integration supports high production yields and stringent quality assurance standards.
Sustainability considerations also benefit from seamless tube flow forming. The process minimizes material waste by precisely controlling deformation and eliminating scrap associated with welding or joining. Energy-efficient drives and cooling technologies further reduce the environmental footprint, aligning with modern manufacturing’s focus on lean and green production.
In conclusion, seamless tube production via flow forming is a sophisticated manufacturing approach that delivers high-quality, durable tubes with complex geometries and enhanced mechanical properties. Its precision, flexibility, and efficiency make it a preferred method in industries where reliability and performance are paramount, driving continued innovation in seamless tube design and applications.
Flow forming’s ability to produce seamless tubes with exceptional dimensional accuracy and superior metallurgical characteristics stems from its precise control over material flow and deformation. Unlike other methods such as extrusion or welding, flow forming applies gradual, controlled pressure through rollers that work in concert with the mandrel’s shape, allowing the metal to stretch and thin uniformly without introducing seams or weak points. This controlled plastic deformation results in tubes with refined grain structures and enhanced mechanical properties, including improved tensile strength, fatigue resistance, and toughness.
The versatility of flow forming also enables the production of tubes with complex geometries that would be challenging or costly to achieve by traditional methods. For instance, tubes can be formed with integrated features like tapered ends, stepped diameters, or flanged sections, which are commonly required in aerospace hydraulic systems or automotive drive shafts. By incorporating these features directly during the flow forming process, manufacturers reduce the need for secondary machining or welding, thereby cutting production time, costs, and potential failure points.
Material selection plays a crucial role in seamless tube flow forming. The process accommodates a broad spectrum of metals, ranging from lightweight aluminum and copper alloys to high-strength steels, titanium, and nickel-based superalloys. Each material presents unique challenges, such as differing flow stress, strain hardening behavior, and thermal sensitivity, which must be carefully managed through process parameter adjustments. Advances in sensor technology and adaptive control systems have improved the ability to tailor forming conditions dynamically, optimizing the process for each material to maximize quality and tool life.
In addition to mechanical and metallurgical advantages, flow-formed seamless tubes benefit from excellent surface finishes, often eliminating the need for extensive post-processing. The roller contact imparts a smooth, work-hardened surface that enhances corrosion resistance and wear properties. This is particularly advantageous in industries like medical device manufacturing, where surface quality is critical for biocompatibility and sterilization.
Automation and integration with smart manufacturing technologies have further advanced seamless tube production via flow forming. Modern machines incorporate robotic handling for loading and unloading billets and finished tubes, minimizing human intervention and reducing the risk of damage or contamination. Inline inspection systems, employing laser micrometers, ultrasonic thickness gauges, and surface scanners, continuously monitor tube quality, enabling real-time corrections and minimizing scrap.
Energy efficiency and sustainability are increasingly prioritized in flow forming operations. The incremental deformation approach consumes less energy than bulk forming processes such as forging or extrusion. Coupled with energy-efficient servo drives, regenerative braking systems, and optimized cooling circuits, flow forming machines reduce operational costs and environmental impact. Additionally, the process generates minimal scrap and supports recycling of metal remnants, aligning with lean manufacturing and circular economy principles.
The growing demand for lightweight, high-performance components in aerospace, automotive, and medical sectors drives ongoing innovation in seamless tube flow forming. New developments focus on expanding size ranges, from microtubes used in medical implants to large-diameter tubes for oil and gas pipelines. Hybrid forming processes that combine flow forming with additive manufacturing or surface treatments are also emerging, enabling the production of tubes with integrated functions or enhanced surface properties.
Quality assurance and traceability are integral to seamless tube production, especially for critical applications. Flow forming systems often integrate digital record-keeping that logs process parameters, inspection data, and material batch information for each tube produced. This data-driven approach facilitates compliance with stringent industry standards and regulatory requirements, ensuring confidence in part performance and safety.
In summary, seamless tube production via flow forming represents a convergence of precise mechanical deformation, advanced process control, material science, and automation. This process enables manufacturers to deliver high-quality, complex tubes that meet the exacting demands of modern engineering applications while optimizing efficiency, sustainability, and cost-effectiveness. As technology and materials continue to evolve, flow forming will remain a key method for producing the next generation of seamless tubes across diverse industries.
Building on its established strengths, seamless tube production via flow forming continues to evolve through integration with advanced manufacturing technologies. The incorporation of artificial intelligence (AI) and machine learning (ML) algorithms is enabling predictive process optimization, where systems analyze vast datasets of forming parameters, material behaviors, and inspection results to anticipate and prevent defects before they occur. This proactive approach improves yield rates and reduces downtime by fine-tuning machine settings automatically based on real-time feedback.
Another significant advancement lies in multi-stage or hybrid flow forming systems that combine traditional flow forming with complementary techniques such as rotary swaging, spinning, or stretch forming. These hybrid setups allow manufacturers to achieve complex tube geometries and material properties that would be difficult or impossible with a single process alone. For example, a tube might undergo initial wall thinning through flow forming, followed by localized shaping or cold working to create flanges or other features, all within an integrated production line.
The scalability of flow forming machines has also improved, enabling the manufacture of tubes ranging from miniature medical-grade microtubes with diameters of a few millimeters to large-diameter industrial tubes used in energy infrastructure. This broad capability supports a wide variety of applications and allows manufacturers to serve diverse markets using a common technology platform, reducing capital investment and streamlining training and maintenance.
Advances in material science, including the development of new alloys and composite metal structures, are expanding the scope of flow-formed seamless tubes. Flow forming’s ability to precisely control deformation at relatively low temperatures helps preserve the unique properties of advanced materials, such as high-strength titanium alloys or nickel-based superalloys, which are critical in aerospace and medical fields. Additionally, techniques such as surface alloying or coating can be integrated post-forming to further enhance corrosion resistance or wear properties.
Sustainability remains a central focus in ongoing process development. Innovations aimed at reducing energy consumption, minimizing lubricants and coolants, and recycling scrap material contribute to greener manufacturing practices. Some manufacturers are experimenting with electrically driven, servo-controlled flow forming machines that offer higher efficiency and cleaner operation compared to hydraulic systems, further reducing environmental impact.
From a digital manufacturing perspective, the seamless integration of flow forming machines with enterprise resource planning (ERP) and supply chain management systems ensures better coordination between production planning, inventory control, and quality assurance. Real-time data exchange facilitates just-in-time manufacturing, reducing inventory costs and enhancing responsiveness to market demand.
Training and workforce development continue to leverage virtual and augmented reality platforms, enabling operators and engineers to simulate machine operation, troubleshoot issues, and practice maintenance procedures in safe, controlled environments. This approach accelerates skill acquisition and supports knowledge retention, critical as flow forming technology becomes more sophisticated.
In regulated industries, enhanced traceability features embedded within flow forming equipment help maintain compliance with international standards such as AS9100 for aerospace or ISO 13485 for medical devices. Detailed digital records capture each tube’s complete manufacturing history, from raw material batch through forming parameters and inspection data, supporting audit readiness and customer confidence.
Looking ahead, seamless tube production via flow forming is poised to integrate more deeply with Industry 4.0 initiatives, leveraging interconnected systems, AI-driven analytics, and autonomous operations to achieve unprecedented levels of quality, efficiency, and flexibility. These advancements will enable manufacturers to meet the increasingly complex demands of modern engineering challenges while maintaining competitive cost structures and sustainability goals.
In conclusion, flow forming remains a cornerstone technology for producing seamless tubes, combining precision mechanical forming, adaptable process control, and digital innovation to deliver superior products. Its ongoing evolution ensures it will continue to serve as a critical enabler of high-performance components across a wide array of industries well into the future.
Flow Forming Machines for High-Strength Alloys
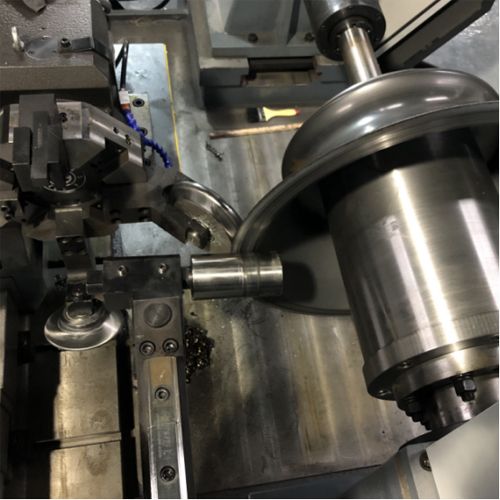
Flow forming machines designed for high-strength alloys are specialized systems engineered to handle the unique challenges posed by materials such as titanium, Inconel, stainless steel, and other advanced metal alloys. These materials are prized for their exceptional mechanical properties—high tensile strength, corrosion resistance, and heat tolerance—but they are also notoriously difficult to form due to their increased hardness, work-hardening behavior, and sensitivity to temperature.
To effectively process high-strength alloys, flow forming machines incorporate several key features that ensure precise control and robust performance. The machine frames are built with extra rigidity and vibration damping to withstand the elevated forming forces required without compromising dimensional accuracy or surface quality. Heavy-duty drive systems, often servo-controlled, deliver the high torque and stable speeds necessary for deforming tough materials consistently.
Tooling design is critical in handling high-strength alloys. Mandrels and rollers are fabricated from wear-resistant materials and may feature advanced coatings or surface treatments to reduce friction and tool wear. This prolongs tool life and maintains consistent forming conditions over extended production runs. Tool geometries are carefully optimized to apply uniform pressure while minimizing localized stresses that could cause cracking or surface defects in the alloy.
Thermal management systems play an especially vital role when working with high-strength alloys, which can be sensitive to temperature fluctuations. Flow forming machines for these materials often integrate cooling channels within mandrels and rollers, as well as external temperature control units, to dissipate heat generated by plastic deformation. Maintaining stable, controlled temperatures helps prevent adverse metallurgical changes such as work hardening beyond desirable levels, microstructural damage, or residual stresses that could weaken the final part.
Advanced control systems equipped with CNC and closed-loop feedback are essential for managing the complex forming dynamics of high-strength alloys. Sensors continuously monitor forces, displacements, temperatures, and vibrations, feeding data to the control unit, which dynamically adjusts roller pressures, feed rates, and mandrel speeds to optimize the process. This adaptability ensures uniform material flow, reduces the risk of defects, and maximizes process repeatability.
Given the premium cost and critical applications of components made from high-strength alloys—such as aerospace structural parts, medical implants, and power generation components—flow forming machines often include integrated quality assurance features. Inline inspection technologies such as laser micrometers, ultrasonic thickness gauging, and surface scanners verify dimensional accuracy and detect potential flaws during forming, enabling immediate corrective actions and minimizing scrap.
Material handling and automation systems further enhance the processing of high-strength alloys by reducing manual intervention, which can introduce contamination or handling damage. Robotic loading and unloading, coupled with quick-change tooling setups, improve throughput and enable efficient transitions between different parts or alloys.
The capability to form high-strength alloys with precision and efficiency allows manufacturers to produce lightweight, high-performance components that meet stringent regulatory and performance standards. The flow forming process enhances mechanical properties by refining grain structure and inducing beneficial residual stresses, resulting in parts with superior strength-to-weight ratios, fatigue resistance, and corrosion durability compared to those produced by conventional methods.
In summary, flow forming machines tailored for high-strength alloys combine robust mechanical design, advanced tooling, precise thermal and process control, and integrated quality monitoring to meet the demanding requirements of modern manufacturing. These machines enable the efficient production of critical components with complex geometries and superior material properties, supporting innovation and reliability across aerospace, medical, energy, and other high-tech industries.
Flow forming high-strength alloys demands meticulous attention to process parameters and machine capabilities due to the inherent material challenges. These alloys often exhibit significant work hardening, which means that as the metal is deformed, it becomes progressively harder and more resistant to further shaping. This behavior necessitates precise control over deformation rates and pressures to avoid excessive stress concentrations that could lead to cracking or premature tool wear. Flow forming machines achieve this through finely tuned servo drives and adaptive control systems that modulate roller force and feed speed in real time, ensuring smooth material flow and uniform wall thickness.
The thermal sensitivity of many high-strength alloys adds complexity to the forming process. Even slight variations in temperature can alter the alloy’s microstructure, affecting ductility and strength. Therefore, effective heat management is critical. Flow forming machines for these materials often incorporate active cooling systems with circulating fluids, temperature sensors, and automated regulation to maintain optimal thermal conditions throughout the operation. By stabilizing temperatures, the process minimizes residual stresses and distortion, which are common causes of part failure or dimensional inaccuracies.
Tooling design plays a pivotal role in extending tool life and maintaining quality when working with abrasive and tough alloys. Mandrels and rollers are crafted from ultra-hard tool steels or carbide materials, frequently enhanced with coatings such as titanium nitride (TiN) or diamond-like carbon (DLC) to reduce friction and resist wear. These coatings not only improve longevity but also prevent surface defects like galling or scoring on the workpiece, which are particularly detrimental when producing precision parts for aerospace or medical applications.
Automation integration in flow forming machines for high-strength alloys is often sophisticated, aiming to optimize both productivity and consistency. Robotic systems manage the handling of heavy billets and finished components, reducing the risk of damage and ensuring repeatable positioning. Quick-change tooling mechanisms minimize downtime between production runs, a vital factor when working with costly alloys where efficient use of machine time directly impacts profitability.
Advanced monitoring technologies embedded in these machines provide continuous feedback on process stability and part quality. Force sensors measure the load exerted by rollers, displacement sensors track material flow and thickness, and vibration sensors detect anomalies indicative of tool wear or material inconsistencies. This sensor data feeds into intelligent control algorithms capable of adjusting parameters on the fly, thereby reducing scrap rates and improving overall yield.
The capacity of flow forming to enhance mechanical properties of high-strength alloys through controlled plastic deformation is a key advantage over other fabrication methods. The process refines grain structure and introduces compressive residual stresses on the tube’s surface, which improve fatigue life and resistance to crack initiation and propagation. Such improvements are critical in safety-sensitive components like aircraft landing gear, surgical implants, or high-performance automotive parts.
Moreover, the process flexibility inherent in flow forming machines allows the production of complex geometries that incorporate varying wall thicknesses, stepped diameters, or integrated flanges and collars—all formed seamlessly without joints or welds. This capability is especially beneficial when manufacturing bespoke or low-volume parts, where conventional machining or welding could compromise structural integrity or significantly increase costs.
Environmental considerations also influence the design and operation of flow forming machines for high-strength alloys. Modern equipment emphasizes energy-efficient servo motors, regenerative braking, and optimized hydraulic systems to reduce power consumption. Cooling and lubrication systems are designed to minimize fluid use and enable recycling, aligning with sustainability goals increasingly demanded by industries such as aerospace and medical device manufacturing.
In the broader context of digital manufacturing, these machines often connect to factory-wide networks, providing real-time data for predictive maintenance and process optimization. By anticipating maintenance needs based on actual machine usage and performance trends, manufacturers can schedule interventions proactively, avoiding unexpected downtime that could disrupt production schedules, especially when dealing with expensive, high-strength alloys.
Training operators and maintenance personnel for these advanced flow forming machines involves specialized programs that blend hands-on experience with virtual simulations. Given the complexity and precision required when working with high-strength alloys, such training ensures that staff can efficiently manage the equipment and respond swiftly to any process deviations, thereby safeguarding product quality and operational efficiency.
Ultimately, flow forming machines tailored for high-strength alloys embody a synthesis of mechanical engineering, materials science, and digital technology. Their ability to deliver high-quality, complex components with enhanced performance characteristics makes them indispensable in sectors where safety, durability, and weight reduction are paramount. As materials evolve and manufacturing demands grow, these machines will continue to advance, integrating new technologies to push the boundaries of what’s possible in high-strength alloy forming.
Continuing the evolution of flow forming machines for high-strength alloys, ongoing innovations focus on further enhancing precision, efficiency, and adaptability to meet increasingly stringent industry requirements. One notable trend is the development of modular machine architectures that allow manufacturers to customize configurations quickly and economically. By swapping or adding modules—such as specialized roller assemblies, advanced cooling units, or enhanced control systems—these machines can be rapidly adapted to new alloy types, part geometries, or production volumes without requiring entirely new equipment.
Another key area of advancement lies in the integration of real-time, high-resolution sensing technologies. Innovations like digital image correlation (DIC) and acoustic emission sensors provide detailed insights into strain distribution and microcrack formation during the flow forming process. By detecting subtle signs of material fatigue or deformation anomalies as they develop, operators and automated systems can intervene immediately, adjusting parameters or halting production to prevent defective parts and minimize waste.
Artificial intelligence (AI) and machine learning (ML) play increasingly significant roles in optimizing flow forming for high-strength alloys. By analyzing vast amounts of historical and real-time process data, AI algorithms identify complex patterns and correlations that human operators might miss. This capability enables predictive adjustments to forming pressures, speeds, and cooling flows, dynamically optimizing the process for each unique batch of material or component design. Over time, the system “learns” and improves, continuously refining output quality and operational efficiency.
In terms of tooling innovations, researchers are exploring new materials and surface treatments that push the limits of durability and friction reduction. For example, the application of nanocomposite coatings or laser-textured surfaces on mandrels and rollers can dramatically improve lubricant retention and reduce metal-to-tool adhesion. This not only extends tool life but also ensures superior surface finishes on formed parts, a critical factor for components destined for aerospace or biomedical use.
Process simulation tools are also becoming more sophisticated, enabling manufacturers to virtually model the flow forming of high-strength alloys with unprecedented accuracy. Coupled with real-world sensor data, these simulations help in predicting material behavior under varying conditions, reducing trial-and-error and accelerating the development of new parts or alloys. This digital twin approach supports rapid innovation cycles and cost-effective scaling from prototype to full production.
Furthermore, collaborative robotics (cobots) are increasingly integrated alongside flow forming machines to handle material loading, unloading, and secondary operations safely and efficiently. These robots work in close proximity to human operators, enhancing flexibility while maintaining safety standards. This collaboration is particularly valuable in high-mix, low-volume production environments common in aerospace and medical sectors, where customization and responsiveness are critical.
Energy efficiency and environmental sustainability remain strong priorities. Flow forming machines now often include regenerative power systems that capture and reuse energy generated during deceleration phases, while advanced cooling techniques leverage eco-friendly fluids and closed-loop circuits to minimize consumption and emissions. These features not only reduce operating costs but also support corporate sustainability commitments and regulatory compliance.
As regulations around traceability tighten, particularly in safety-critical industries, flow forming machines for high-strength alloys increasingly incorporate blockchain or secure cloud-based data logging systems. These technologies ensure tamper-proof records of every process step, material batch, and inspection result, enhancing transparency and accountability throughout the supply chain.
Lastly, the human-machine interface (HMI) continues to evolve, with intuitive touchscreen controls, customizable dashboards, and augmented reality (AR) overlays that assist operators in setup, monitoring, and troubleshooting. AR applications can superimpose operational instructions, tool alignment guides, or real-time sensor data onto the physical machine view, dramatically reducing setup time and minimizing human error.
In conclusion, flow forming machines for high-strength alloys are becoming ever more advanced, blending mechanical robustness with cutting-edge digital technologies. This fusion empowers manufacturers to produce complex, high-performance components with exceptional precision and reliability, meeting the demanding needs of industries where material excellence and process control are non-negotiable. As these machines continue to evolve, they will play a pivotal role in driving innovation, sustainability, and competitiveness in high-strength alloy manufacturing worldwide.
Flow Forming Machine Capabilities for Thin-Wall Parts
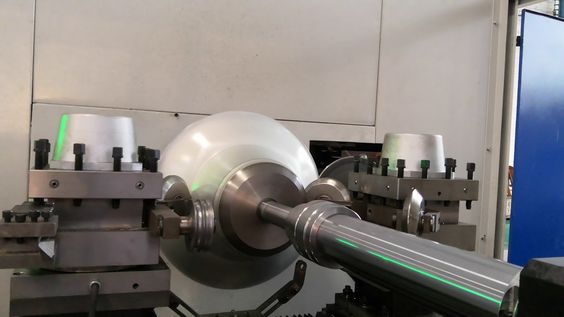
Flow forming machines are exceptionally well-suited for producing thin-wall parts, offering capabilities that address the unique challenges associated with manufacturing components that require precise wall thickness, high dimensional accuracy, and superior surface quality. Thin-wall parts—commonly used in aerospace, automotive, medical, and energy sectors—demand a manufacturing process that can achieve significant metal thinning without compromising structural integrity or introducing defects such as wrinkling, cracking, or uneven thickness distribution.
One of the core capabilities of flow forming machines in thin-wall production is their ability to apply controlled, incremental plastic deformation using multiple rollers that gradually reduce wall thickness while maintaining consistent material flow. This gradual forming approach minimizes the risk of defects and enables the production of parts with walls as thin as a fraction of a millimeter, depending on the material and geometry. The machine’s design emphasizes rigidity and precision to support the delicate balance between sufficient forming pressure and avoidance of excessive stress concentrations.
Precise control over forming parameters—such as roller feed rate, mandrel rotation speed, roller pressure, and axial positioning—is critical for thin-wall flow forming. Modern machines utilize CNC controls with closed-loop feedback systems that continuously monitor these parameters, adjusting them dynamically in response to real-time sensor data. This adaptability ensures uniform wall thickness and tight dimensional tolerances throughout the part, even in complex shapes or tapered sections.
Thermal management is also crucial when producing thin-wall components, as the metal’s reduced cross-section is more susceptible to temperature variations that can affect ductility and cause distortions. Flow forming machines incorporate active cooling mechanisms in mandrels and rollers to dissipate heat generated during deformation, stabilizing the process and preserving metallurgical properties critical for part performance.
The tooling design for thin-wall flow forming prioritizes minimizing friction and avoiding surface damage. Mandrels and rollers are manufactured from high-precision, wear-resistant materials with carefully engineered surface finishes to promote smooth metal flow and prevent galling or scoring. Lubrication systems are integrated to reduce friction further and enhance surface quality on the final part, which is especially important for thin walls where surface defects can compromise strength or aesthetic requirements.
Flow forming machines also excel in producing thin-wall parts with complex geometries, including variable wall thicknesses, tapered sections, and integrated features like flanges or collars. The flexibility of the process allows manufacturers to meet intricate design specifications without the need for secondary machining or assembly, thereby reducing production time and costs while improving structural integrity.
Automation and inline inspection systems are integral to maintaining quality in thin-wall flow forming. Laser micrometers, ultrasonic gauges, and surface profilometers provide continuous measurement of wall thickness, diameter, and surface finish, feeding data into the control system for immediate process corrections. This real-time quality assurance reduces scrap rates and ensures consistent production output.
Material versatility is another strength of flow forming machines for thin-wall parts. They can process a wide range of metals, from lightweight aluminum alloys used in aerospace and medical devices to high-strength steels and titanium alloys for automotive and energy applications. This adaptability enables manufacturers to select the optimal material for their specific performance needs while achieving challenging thin-wall requirements.
Finally, flow forming machines designed for thin-wall parts emphasize energy efficiency and sustainability. Incremental deformation consumes less energy than bulk forming processes, and the precise control reduces waste and scrap. Advanced cooling and lubrication systems minimize fluid consumption, supporting environmentally responsible manufacturing practices.
In summary, flow forming machines possess specialized capabilities that make them ideal for producing thin-wall parts with exceptional precision, quality, and efficiency. Their controlled deformation process, advanced control systems, and tooling innovations enable the creation of lightweight, high-performance components that meet demanding industry standards across a wide range of applications.
Flow forming machines continue to push the boundaries of thin-wall part manufacturing by integrating ever more advanced technologies and process innovations. One critical development is the increasing use of real-time adaptive control systems powered by sophisticated sensors and data analytics. These systems monitor variables such as roller force, mandrel position, wall thickness, and vibration patterns at high frequencies, allowing the machine to instantly adjust forming parameters to compensate for material inconsistencies, tool wear, or environmental changes. This dynamic adaptability is especially vital in thin-wall production, where even minor deviations can lead to part failure or excessive scrap.
The design of flow forming machines also incorporates enhanced structural stiffness and vibration damping to stabilize the forming process. Thin walls are inherently more sensitive to external disturbances and machine-induced vibrations, which can cause surface defects or dimensional inaccuracies. By employing heavy-duty frames constructed from advanced alloys, integrating isolators, and optimizing component layouts, manufacturers ensure a stable, repeatable forming environment that maintains part quality even during high-speed production runs.
Innovations in tooling are helping to further refine thin-wall forming capabilities. Tool surfaces are engineered at the micro and nano scale to optimize lubrication retention and minimize metal-to-tool friction. Some flow forming machines feature interchangeable roller sets with varying profiles and coatings, allowing quick adaptation to different thin-wall geometries or materials. These tooling advancements reduce wear, extend maintenance intervals, and enhance the finish and dimensional precision of delicate parts.
The ability to handle a wide spectrum of materials is continually enhanced by improved machine control and tooling design. Materials ranging from soft aluminum alloys to ultra-high-strength steels and exotic titanium alloys can be formed into thin-walled tubes, cylinders, or complex shapes with consistent quality. Each material presents unique challenges regarding ductility, strain hardening, and thermal sensitivity, but modern flow forming machines address these through tailored process recipes and specialized cooling or heating arrangements, ensuring optimal forming conditions.
Process integration is becoming a significant factor in maximizing thin-wall flow forming efficiency. Many machines are now equipped to interface seamlessly with upstream and downstream manufacturing steps such as tube cutting, heat treatment, surface finishing, and automated inspection. This integration supports just-in-time production models, reduces handling damage, and minimizes lead times, which are particularly critical when producing thin-walled components for high-value applications like aerospace or medical devices.
Sustainability considerations influence machine design and operation as well. The precise nature of flow forming means less raw material is wasted compared to machining or forging, contributing to more environmentally friendly production. Energy-efficient motors, regenerative braking systems, and closed-loop cooling circuits reduce the ecological footprint of the process. Additionally, improvements in lubricant formulations and delivery systems decrease chemical consumption and facilitate recycling, aligning manufacturing with increasingly stringent environmental regulations.
Operator interfaces have evolved to simplify complex thin-wall flow forming processes. Advanced graphical HMIs provide intuitive visualizations of real-time machine performance, process trends, and quality metrics, enabling operators to monitor and respond to process conditions effectively. Augmented reality and virtual reality tools are beginning to assist with training and maintenance, helping operators develop the skills required for precision thin-wall forming while reducing downtime caused by errors or misadjustments.
Quality assurance remains paramount, with inline, non-destructive testing methods embedded into flow forming machines ensuring that every thin-wall part meets strict standards. Technologies such as laser-based dimensional scanning, ultrasonic thickness measurements, and eddy current flaw detection provide comprehensive inspection without slowing production. Data collected during forming can be linked to digital traceability systems, which document each part’s manufacturing history for regulatory compliance and customer confidence.
As manufacturing demands evolve, the modularity and flexibility of flow forming machines allow quick adaptation to new product designs or material developments. This responsiveness is critical for industries requiring rapid innovation cycles or customized components, where thin-wall parts must meet unique performance and safety criteria. The ability to produce such parts efficiently without sacrificing quality gives flow forming a competitive edge over alternative forming methods.
Looking forward, emerging technologies such as artificial intelligence-driven process optimization, edge computing for low-latency control, and advanced materials with tailored microstructures will further enhance the capabilities of flow forming machines for thin-wall applications. These innovations promise higher throughput, improved part performance, and reduced costs, ensuring that flow forming remains a preferred method for manufacturing precision thin-walled components across diverse high-tech industries.
In conclusion, the capabilities of flow forming machines for thin-wall parts rest on their ability to combine precise, controlled deformation with advanced sensing, adaptive control, and tooling innovations. This synergy delivers high-quality, lightweight, and structurally sound components that meet the exacting demands of modern engineering applications, driving ongoing growth and innovation in thin-wall manufacturing.
Flow forming machines continue to evolve, offering ever-greater precision and efficiency in the production of thin-wall parts, responding to the growing demands for lighter, stronger, and more complex components across multiple industries. One of the most impactful advancements is the integration of smart manufacturing technologies, enabling the machines to operate within an Industry 4.0 framework. This allows seamless communication between flow forming equipment and other manufacturing systems, creating interconnected production lines that can optimize workflows, track materials in real time, and predict maintenance needs with unprecedented accuracy.
With these connected systems, manufacturers can implement predictive maintenance strategies that significantly reduce unplanned downtime. Sensors embedded within critical components monitor wear and stress levels, sending alerts when parameters approach limits. This preemptive approach ensures that machine servicing happens only when necessary, extending machine life and maintaining consistent quality in thin-wall production, which is particularly sensitive to process variability.
The continuous refinement of servo-driven actuation systems also enhances the responsiveness and smoothness of forming actions. These systems deliver precise, programmable force and speed profiles that can be adjusted on the fly, accommodating subtle changes in material behavior or design variations without interrupting production. This flexibility is essential when working with high-performance alloys or composite materials that exhibit complex deformation characteristics during thinning.
Additionally, the use of advanced simulation software has become an integral part of the flow forming process. Prior to physical trials, virtual models simulate material flow, stress distribution, and temperature effects throughout the forming cycle, allowing engineers to optimize tooling geometry, process parameters, and cooling strategies. This not only reduces development time but also minimizes costly trial-and-error runs, accelerating the path from design to production for thin-wall parts.
In the realm of materials science, ongoing research into nanostructured coatings and surface engineering is driving improvements in tooling durability and surface finish quality. Innovations such as diamond-like carbon (DLC) coatings or multilayer ceramic composites provide outstanding resistance to abrasion and corrosion, enabling flow forming machines to maintain tight tolerances and smooth finishes over extended production runs with minimal tool replacement.
Another promising development is hybrid flow forming processes, where traditional metal flow forming is combined with supplementary technologies like localized laser heating or ultrasonic vibration. These hybrid techniques can reduce forming forces, improve material ductility, and allow more extreme thinning or complex geometries without compromising integrity. Such capabilities open new possibilities for producing ultra-thin, high-strength components previously unattainable with conventional flow forming alone.
Environmental sustainability remains a strong driver of innovation. Efforts to reduce waste and energy consumption include optimizing lubrication systems to use biodegradable fluids and implementing closed-loop fluid recycling. Regenerative drives capture and reuse energy during deceleration phases, lowering overall power consumption. These green initiatives align flow forming with global trends toward more sustainable manufacturing practices.
Operator training and knowledge transfer also benefit from digital tools. Virtual and augmented reality platforms provide immersive environments where operators can practice machine setup, simulate troubleshooting, and learn maintenance procedures safely and efficiently. This reduces errors, enhances safety, and shortens the learning curve for handling increasingly sophisticated flow forming systems.
As additive manufacturing (3D printing) advances, its integration with flow forming is emerging as a complementary approach. For example, near-net-shape parts produced via additive methods can undergo flow forming to achieve final dimensional precision, surface finish, and mechanical properties, combining the strengths of both technologies. This hybrid manufacturing approach is especially valuable for complex thin-wall components requiring lightweight structures with superior performance.
In summary, the ongoing evolution of flow forming machines for thin-wall parts encompasses advances in smart automation, materials science, process integration, and sustainability. These developments empower manufacturers to meet the challenges of producing lightweight, high-strength, and intricately shaped components efficiently and reliably. By continuously pushing technological boundaries, flow forming remains a critical enabler in modern manufacturing, supporting innovation across aerospace, automotive, medical, and beyond.
Warm & Hot Flow Forming Technologies
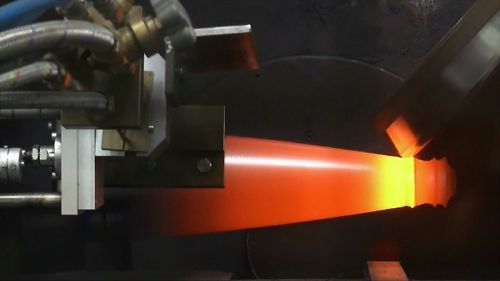
Warm and hot flow forming technologies are specialized variants of traditional flow forming processes that involve forming metal components at elevated temperatures to improve material ductility, reduce forming forces, and enable the shaping of materials that are otherwise difficult to deform at room temperature. These techniques are particularly valuable for high-strength alloys, titanium, nickel-based superalloys, and other advanced materials used in aerospace, automotive, energy, and medical industries where superior mechanical properties and complex geometries are required.
Warm flow forming typically operates at temperatures below the material’s recrystallization point, usually in the range of 200°C to 500°C depending on the alloy. At these intermediate temperatures, the metal softens sufficiently to reduce flow stress and enhance plasticity without causing significant microstructural changes or grain growth. This balance allows for improved formability, reduced roller loads, and extended tool life while maintaining the material’s desired mechanical properties. Warm forming is often preferred for alloys that are prone to strain hardening or cracking during cold forming but do not require the full benefits or complexities of hot forming.
Hot flow forming, in contrast, occurs at or above the recrystallization temperature of the metal, often exceeding 800°C for steels and even higher for titanium or nickel alloys. At these elevated temperatures, metals exhibit superplastic or near-superplastic behavior, allowing for extreme deformation with minimal risk of cracking. Hot flow forming enables the production of highly complex shapes with large reductions in wall thickness, which would be challenging or impossible with cold or warm forming methods. Additionally, the high temperatures promote dynamic recrystallization, which refines grain size and can improve mechanical properties such as toughness and fatigue resistance in the finished part.
Implementing warm and hot flow forming requires specialized equipment and process controls to manage the thermal environment precisely. Machines are equipped with induction heaters, resistance heating elements, or furnace chambers to uniformly raise the temperature of the billet or workpiece before and during forming. Temperature sensors embedded in the mandrel, rollers, or workpiece provide real-time feedback to maintain consistent thermal conditions, which is crucial to avoid thermal gradients that can cause distortion or uneven material properties.
Thermal insulation and controlled cooling systems are also integral components of these technologies. After forming, parts often require controlled cooling rates to achieve specific microstructures or mechanical properties, necessitating integrated quenching or tempering setups. Some systems include inert gas atmospheres or vacuum chambers to prevent oxidation and surface contamination at high temperatures, preserving the integrity and finish of the component.
Tooling for warm and hot flow forming must withstand the combined effects of high temperature, pressure, and friction. Mandrels and rollers are fabricated from high-temperature-resistant alloys or ceramics, often coated with advanced thermal barrier coatings to extend service life. Lubrication in these processes is more challenging, requiring specialized high-temperature lubricants or solid lubricants that can operate effectively without degrading or causing surface defects.
Process control in warm and hot flow forming is sophisticated, utilizing CNC systems integrated with thermal management and force feedback loops. This integration allows precise regulation of forming speed, roller pressure, and temperature profiles throughout the operation. The ability to adjust parameters dynamically is essential to compensate for changes in material behavior as it cools or deforms, ensuring consistent part quality and dimensional accuracy.
Warm and hot flow forming technologies offer several advantages. They enable the forming of materials that are too brittle or hard for cold forming, reduce the risk of defects like cracking or wrinkling, and often produce parts with improved metallurgical properties such as refined grain structure and enhanced fatigue resistance. Additionally, these methods can achieve greater thickness reductions and more intricate geometries in a single operation, improving production efficiency and reducing the need for secondary machining or joining.
Applications of warm and hot flow forming include aerospace components like jet engine casings, gas turbine parts, and structural airframe elements where high strength-to-weight ratios and complex shapes are critical. In the automotive sector, these technologies support the production of lightweight, high-performance driveline components and chassis parts. The medical field benefits from the ability to form biocompatible alloys into thin-walled implants and surgical instruments with precise dimensional control.
Despite their benefits, warm and hot flow forming processes pose challenges such as higher equipment costs due to heating and insulation requirements, increased energy consumption, and more complex process setup and maintenance. However, ongoing advances in thermal management, automation, and materials science continue to mitigate these drawbacks, making warm and hot flow forming increasingly accessible and cost-effective.
In summary, warm and hot flow forming technologies extend the capabilities of traditional flow forming by enabling the shaping of difficult-to-form materials at elevated temperatures. Through precise thermal control, robust tooling, and advanced process management, these methods produce high-quality, complex components with superior mechanical properties, meeting the stringent demands of modern engineering applications across a variety of high-performance industries.
Building on the foundation of warm and hot flow forming technologies, the continued evolution of these processes focuses on further refining temperature control, improving energy efficiency, and expanding the range of materials and geometries that can be effectively formed. Precise temperature uniformity throughout the workpiece remains a critical factor in achieving consistent microstructure and mechanical properties. To this end, advanced induction heating systems with multi-zone control have been developed, enabling targeted heating of specific areas on the workpiece to accommodate complex shapes and variable thicknesses. This localized heating reduces overall energy consumption and minimizes thermal distortion, ensuring dimensional accuracy and structural integrity.
Thermal simulation software plays an increasingly important role in designing and optimizing warm and hot flow forming operations. By modeling heat transfer, phase transformations, and material flow simultaneously, engineers can predict potential issues such as overheating, thermal gradients, or undesirable microstructural changes before production. These simulations aid in selecting optimal heating profiles, roller speeds, and cooling strategies, thus reducing costly trial-and-error and accelerating product development cycles.
Integration of sensor technologies has expanded beyond temperature and force monitoring to include acoustic emission, infrared thermography, and even in situ metallographic analysis using advanced optical sensors. These tools provide real-time insights into the forming process, detecting early signs of defects like cracking, delamination, or surface oxidation. The data collected feeds into machine learning algorithms that continuously improve process stability and part quality by suggesting corrective actions or parameter adjustments without operator intervention.
Tooling innovation continues to be a key area of focus. New materials such as ultra-high-temperature ceramics and refractory metal alloys extend the life of forming tools under extreme thermal and mechanical stresses. Innovative coatings combining low friction with high thermal resistance enhance performance, reducing downtime caused by tool wear or surface damage. Moreover, modular tooling systems allow for rapid changeovers and customization to suit different part geometries or materials, improving manufacturing flexibility.
The combination of warm or hot flow forming with post-forming heat treatments is another area seeing significant progress. Some advanced systems integrate induction or laser heat treatment modules that immediately follow the forming stage, enabling controlled tempering, aging, or solution treatment. This integrated approach refines microstructure and mechanical properties efficiently, reducing lead times and minimizing handling risks associated with transferring parts between separate processes.
From an environmental and economic perspective, efforts to improve the sustainability of warm and hot flow forming are gaining traction. Energy recovery systems capture waste heat generated during forming and use it to preheat incoming billets or power auxiliary systems, enhancing overall process efficiency. The adoption of greener lubricants and the implementation of closed-loop lubrication circuits reduce environmental impact and operating costs. Additionally, smart scheduling algorithms optimize machine utilization, balancing energy consumption with production demands.
The expanding capabilities of warm and hot flow forming technologies are opening doors to new industrial applications. In aerospace, the ability to shape nickel-based superalloys and titanium alloys with complex features and high structural integrity supports the development of next-generation engines and airframes with improved fuel efficiency and durability. The automotive sector benefits from lightweight, high-strength components that contribute to electric vehicle range extension and crash safety improvements. In energy, flow-formed components are critical in manufacturing turbine blades, pressure vessels, and high-performance piping systems that operate under extreme thermal and mechanical conditions.
Training and workforce development have adapted alongside these technological advances. Virtual reality (VR) and augmented reality (AR) platforms provide immersive environments where operators and engineers can gain hands-on experience with warm and hot flow forming machines in simulated conditions. These tools improve understanding of thermal dynamics, process controls, and safety protocols, fostering a skilled workforce capable of maximizing the potential of these sophisticated systems.
Looking to the future, research is underway to combine warm and hot flow forming with emerging technologies such as additive manufacturing and advanced composite materials. Hybrid processes that incorporate printed metal preforms followed by flow forming can create complex, near-net-shape components with tailored properties, reducing waste and production time. Similarly, efforts to develop flow forming techniques compatible with metal matrix composites or layered materials promise new possibilities for high-performance, multifunctional components.
In conclusion, warm and hot flow forming technologies continue to mature and expand, driven by innovations in thermal management, tooling, process control, and integration with digital manufacturing systems. These advances empower manufacturers to produce high-quality, complex components from challenging materials efficiently and sustainably, addressing the rigorous demands of modern engineering fields and paving the way for future breakthroughs in metal forming.
Advancing beyond current capabilities, warm and hot flow forming technologies are poised to benefit significantly from the integration of artificial intelligence (AI) and machine learning (ML) in process optimization and predictive quality control. AI algorithms analyze vast datasets generated during forming—encompassing temperature profiles, force measurements, material properties, and sensor feedback—to identify subtle patterns and correlations that human operators might miss. This enables the development of adaptive control strategies that dynamically adjust process parameters in real time, optimizing forming conditions to consistently produce defect-free thin-wall components with complex geometries and demanding performance criteria.
Furthermore, the incorporation of digital twins—virtual replicas of the physical flow forming system—allows engineers to simulate and experiment with different process scenarios without interrupting production. These digital twins, continuously updated with live sensor data, provide predictive insights into machine behavior, tool wear, and potential failure modes. This capability enhances maintenance scheduling, reduces downtime, and ensures that quality standards are maintained across extended production runs, which is particularly valuable when working with expensive materials and tight tolerances typical of warm and hot flow forming applications.
Material innovations also continue to drive enhancements in warm and hot flow forming. Research into novel alloys with engineered microstructures—such as gradient or hierarchical grain structures—improves formability at elevated temperatures while maintaining or enhancing mechanical performance. These materials allow for more aggressive thinning and complex shaping, expanding the design envelope for components in aerospace, medical, and high-performance automotive sectors. Additionally, smart materials with self-healing or adaptive properties are being explored, potentially enabling components that can respond to stress or damage over their service life.
Sustainability efforts are deepening with the integration of lifecycle assessment (LCA) tools directly into manufacturing planning. These tools quantify the environmental impact of flow forming processes, including energy use, emissions, and material waste, guiding decisions to optimize resource efficiency. Coupled with renewable energy sources and closed-loop recycling systems, warm and hot flow forming can become a key contributor to circular economy models in metal manufacturing.
On the industrial scale, the scalability and modularity of flow forming equipment are improving, allowing manufacturers to tailor production capacity to demand while maintaining process quality. This flexibility supports both mass production of standardized components and small-batch manufacturing of highly customized parts without extensive retooling. Such adaptability is crucial for industries like aerospace and medical devices, where rapid innovation and frequent design updates are common.
Safety and ergonomics are also enhanced in modern warm and hot flow forming environments. Automated material handling, enclosed heating zones, and advanced monitoring systems minimize operator exposure to high temperatures and mechanical hazards. Collaborative robots (cobots) assist with machine setup, loading, and inspection tasks, reducing physical strain and improving productivity.
In sum, the trajectory of warm and hot flow forming technologies is shaped by a convergence of digitalization, materials science, sustainability, and automation. These advances collectively enable the manufacturing of ever more complex, high-performance thin-wall components with greater efficiency, reliability, and environmental responsibility. As research and industrial adoption continue, warm and hot flow forming will remain at the forefront of precision metal forming, meeting the evolving challenges of cutting-edge engineering applications worldwide.
Cold Flow Forming Techniques
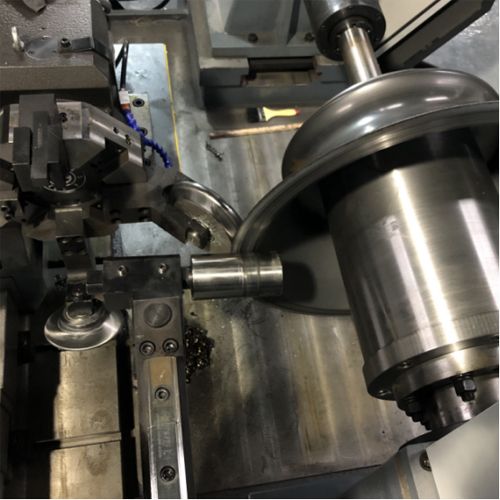
Cold flow forming techniques refer to the process of shaping metal components at or near room temperature, without the application of external heating to the workpiece. Unlike warm or hot flow forming, cold flow forming relies on the metal’s inherent ductility and plastic deformation behavior at ambient temperatures to achieve thinning, elongation, and shaping of tubular or cylindrical parts. This technique is widely used in manufacturing industries where dimensional precision, surface finish, and mechanical properties are critical, such as automotive, aerospace, defense, and general engineering.
Cold flow forming involves feeding a metal blank or preform onto a rotating mandrel, where one or more rollers apply high pressure to plastically deform the material. The rollers progressively reduce the wall thickness and extend the length of the part, often in multiple passes. Because the process occurs without heating, the metal undergoes strain hardening, which increases strength and hardness but can reduce ductility. Careful control of process parameters is essential to prevent defects like cracking, wrinkling, or excessive residual stresses.
One of the main advantages of cold flow forming is the ability to produce parts with excellent dimensional accuracy and surface finish, often eliminating or reducing the need for subsequent machining or finishing operations. The process also imparts beneficial mechanical properties due to strain hardening, improving fatigue resistance and tensile strength. Cold flow forming is especially suited for producing lightweight, high-strength components such as precision shafts, aerospace structural members, and high-performance automotive parts.
To accommodate the challenges of forming metals at ambient temperatures, cold flow forming machines are engineered with robust frames and precision control systems. CNC technology enables precise manipulation of roller forces, mandrel rotation speeds, and feed rates to optimize material flow and minimize defects. Advanced monitoring systems track parameters such as forming force, torque, and displacement to ensure consistent quality throughout production.
The selection of materials for cold flow forming is influenced by their formability at room temperature. Common materials include low and medium carbon steels, stainless steels, aluminum alloys, and some copper alloys. Metals with high ductility and good work-hardening characteristics are preferred, as they tolerate large plastic strains without cracking. However, very hard or brittle materials may require preheating or alternative forming methods to avoid damage.
Tooling design is critical for successful cold flow forming. Rollers and mandrels must provide uniform pressure distribution to control wall thickness and surface quality. Tool surfaces are typically hardened and precision ground to withstand the stresses of cold forming and maintain tight tolerances. Lubrication plays an important role in reducing friction and wear, enabling smoother material flow and longer tool life.
Cold flow forming is often combined with other manufacturing processes to achieve complex geometries or enhanced properties. For instance, parts may be cold flow formed to near-final dimensions and then heat-treated to relieve residual stresses or modify microstructure. Additional machining or joining operations can then complete the component assembly.
In terms of applications, cold flow forming is extensively used to produce components such as seamless tubes, gun barrels, drive shafts, aerospace structural members, and pressure vessels. Its ability to create thin-walled, high-strength parts with excellent surface finish and dimensional control makes it indispensable in industries where performance and reliability are paramount.
Despite its benefits, cold flow forming has limitations. The strain hardening effect increases forming forces and can limit the achievable thickness reduction in a single pass. Complex shapes or very large thickness changes may require multiple stages or alternative processes. Additionally, the process can introduce residual stresses that may necessitate post-forming stress relief treatments.
In summary, cold flow forming techniques offer an efficient, cost-effective method to manufacture high-precision, thin-walled components from ductile metals without the need for heating. By leveraging advanced machine control, tooling design, and process monitoring, manufacturers achieve parts with superior mechanical properties and surface quality suitable for demanding applications across various industries.
Cold flow forming continues to be refined through improvements in machine design, process control, and material science, enhancing its capabilities and expanding its range of applications. One significant trend is the increased use of servo-driven CNC systems, which provide highly precise and repeatable control over roller pressure, mandrel rotation speed, and feed rate. This precision allows manufacturers to optimize forming parameters for different materials and geometries, minimizing defects such as wrinkling, cracking, or ovality while maximizing material utilization and part consistency.
The development of real-time monitoring and feedback systems plays a crucial role in modern cold flow forming. Sensors integrated into the machine detect variables like forming force, torque, vibration, and surface temperature, feeding data to control algorithms that adjust process parameters on the fly. This closed-loop control helps maintain optimal forming conditions, compensate for variations in material properties or tooling wear, and ensure consistent part quality across large production runs. Machine learning techniques are increasingly being applied to analyze these datasets, enabling predictive maintenance and further process optimization.
Material advancements also contribute to the evolution of cold flow forming. The increasing use of advanced alloys, including high-strength aluminum, titanium, and dual-phase steels, demands careful balancing of process parameters to avoid cracking while achieving desired strength and dimensional accuracy. Research into metal microstructure and work-hardening behavior informs the development of forming schedules tailored to these materials, sometimes incorporating intermediate annealing or partial heating to enhance ductility without sacrificing the benefits of cold forming.
Tooling innovations focus on enhancing durability and reducing friction. Roller and mandrel surfaces are treated with advanced coatings such as diamond-like carbon (DLC) or ceramic composites to resist wear and maintain surface finish quality over extended runs. Tool geometries are optimized through finite element analysis to distribute forming stresses evenly, minimizing localized deformation and prolonging tool life. Additionally, modular tooling systems facilitate rapid changeovers between part designs, improving production flexibility and reducing downtime.
Cold flow forming is also increasingly integrated into multi-process manufacturing lines. For example, seamless tubes or cylinders produced by cold flow forming may proceed directly to machining, heat treatment, or assembly without intermediate storage, streamlining production workflows. Hybrid approaches combining cold flow forming with additive manufacturing or precision forging enable the production of near-net-shape components with complex features and superior mechanical properties.
Environmental and economic factors drive improvements in cold flow forming efficiency and sustainability. Optimized lubrication systems use environmentally friendly, biodegradable fluids that reduce friction and tool wear while minimizing environmental impact. Energy-efficient servo motors and regenerative drives capture and reuse energy during deceleration phases, lowering overall power consumption. Process optimization reduces scrap rates and material waste, contributing to more sustainable manufacturing operations.
Applications of cold flow forming remain broad and diverse, reflecting its versatility. In the automotive industry, it produces lightweight drive shafts, steering components, and structural tubes that improve vehicle performance and fuel efficiency. Aerospace uses cold flow forming for precision engine components, landing gear parts, and airframe structures requiring tight tolerances and high fatigue resistance. The defense sector benefits from cold flow formed gun barrels and missile casings that combine strength with dimensional accuracy. Additionally, cold flow forming is employed in manufacturing medical devices, sporting goods, and industrial equipment where precise, thin-walled metal parts are essential.
Challenges associated with cold flow forming include managing the strain hardening effect, which increases forming forces and can limit thickness reductions per pass. Engineers often design multi-pass processes or incorporate intermediate stress relief annealing to address this. Controlling residual stresses and distortion in finished parts remains critical, especially for applications demanding high dimensional stability. Ongoing research focuses on advanced process modeling and simulation to better predict material behavior and optimize forming sequences.
Looking forward, cold flow forming is expected to benefit from further digitalization and automation. The integration of AI-driven process control, enhanced sensor networks, and cloud-based data analytics will enable smarter, more adaptable manufacturing systems capable of producing complex parts with minimal human intervention. Coupled with continued materials innovation and tooling development, these advances will extend the applicability of cold flow forming to new materials, more complex geometries, and tighter tolerances.
In essence, cold flow forming techniques offer a mature yet continually evolving metal forming solution that balances precision, mechanical performance, and cost-efficiency. As technology advances, these processes will remain indispensable for producing high-quality, thin-walled components across a wide array of demanding industrial applications.
Building on these advancements, cold flow forming is increasingly being integrated with Industry 4.0 principles to create smart manufacturing environments. This integration involves the use of interconnected machines, real-time data exchange, and advanced analytics to optimize production workflows. Digital twins of cold flow forming machines and processes allow manufacturers to simulate and predict outcomes before physical production, reducing setup times and minimizing material waste. Such digital integration enables rapid adaptation to new part designs or material changes while maintaining strict quality control.
Automation in cold flow forming operations is also expanding. Robotics and automated material handling systems reduce manual intervention, improving workplace safety and productivity. Automated inspection systems, including laser scanning and machine vision, verify dimensional accuracy and surface quality immediately after forming, allowing for early detection of defects and reducing scrap rates. These automated quality assurance measures are vital in industries like aerospace and medical devices, where traceability and certification standards are stringent.
The scope of cold flow forming applications continues to grow as new materials and complex component designs emerge. Lightweight metals such as magnesium alloys and high-entropy alloys are being explored for cold flow forming, though their unique deformation characteristics require tailored process strategies. Multi-material components, combining layers or inserts of different metals, challenge traditional forming but open pathways to parts with customized mechanical and thermal properties. Cold flow forming’s precision and control make it a promising candidate for fabricating such advanced structures.
Research into hybrid manufacturing processes combines cold flow forming with other techniques to leverage their complementary advantages. For example, pairing cold flow forming with incremental sheet forming or laser-assisted forming can enable the creation of intricate geometries that were previously unattainable. These hybrid methods also allow localized control of material properties and thickness, enhancing component performance while reducing weight.
Sustainability considerations drive innovation in tooling and consumables. Longer-lasting tool coatings reduce waste and downtime, while environmentally friendly lubricants and recycling of metal swarf contribute to greener operations. Energy recovery systems and smarter scheduling reduce the carbon footprint of cold flow forming plants, aligning manufacturing with global sustainability goals.
Workforce development evolves alongside technological progress. Virtual and augmented reality training tools offer immersive, hands-on learning experiences without the risks or costs of live machine operation. These platforms help new operators understand the nuances of cold flow forming, such as the impact of roller positioning and feed rate on material behavior. Continuous education ensures that personnel stay current with evolving process capabilities and digital tools.
In conclusion, cold flow forming techniques stand at the intersection of precision engineering, materials science, and digital innovation. Their adaptability, efficiency, and ability to produce high-quality, thin-walled components make them essential in modern manufacturing landscapes. As technologies converge and advance, cold flow forming will continue to expand its role in producing next-generation components for aerospace, automotive, medical, defense, and beyond—meeting increasingly complex demands while supporting sustainable, smart manufacturing ecosystems.
Flow Forming vs. Metal Spinning
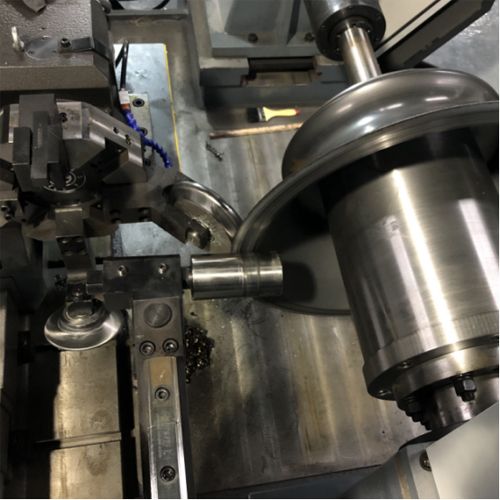
Flow forming and metal spinning are both metal forming processes used to shape rotationally symmetric parts, but they differ significantly in technique, applications, capabilities, and outcomes.
Flow Forming is a high-precision, incremental metal forming process that involves plastically deforming a preform (usually a thick-walled cylindrical blank) over a mandrel by applying controlled pressure with rollers while the workpiece rotates. The process reduces wall thickness and elongates the part axially, producing thin-walled, high-strength components with excellent dimensional accuracy and surface finish. Flow forming typically requires specialized machinery with CNC control to precisely manage roller forces, feed rates, and rotation speeds. It is widely used in aerospace, automotive, and defense industries to produce complex, thin-walled cylinders such as rocket motor casings, seamless tubes, pressure vessels, and high-performance shafts.
Metal Spinning, also known as spin forming, is a simpler, more manual or semi-automated process where a flat metal blank is rotated at high speed on a lathe-like machine and shaped over a mandrel using one or more handheld or mechanized tools. The metal is plastically deformed by pressing or “spinning” it against the mandrel, creating hollow, axisymmetric shapes such as cones, domes, or shallow cylinders. Metal spinning is typically suited for lower-volume production or prototyping because of its flexibility and relatively low tooling costs. It is commonly used for manufacturing lighting fixtures, musical instrument parts, cookware, and decorative elements.
Key Differences:
- Material Thickness and Strength: Flow forming starts with thicker blanks and produces parts with significantly reduced wall thickness while maintaining or enhancing mechanical properties through strain hardening. Metal spinning generally works with thinner sheet metal and produces parts with less thickness reduction and strength improvement.
- Dimensional Precision and Surface Finish: Flow forming offers superior control over dimensions and surface finish due to CNC control and gradual, uniform deformation. Metal spinning often yields parts with less dimensional accuracy and rougher surface finish, requiring more post-processing.
- Automation and Production Volume: Flow forming is highly automated and suited for medium to high-volume production with repeatable quality. Metal spinning can be manual or semi-automated, making it flexible for low to medium volumes and custom parts.
- Complexity of Shapes: Flow forming excels at producing cylindrical or tubular parts with thin walls and complex internal geometries, while metal spinning is better suited to simpler shapes like cones, domes, and shallow cylinders.
- Tooling Costs: Flow forming requires specialized, often expensive tooling and mandrels designed for specific parts, while metal spinning needs minimal tooling investment, often just a mandrel and basic forming tools.
In summary, flow forming is a precision, high-performance metal forming technique ideal for producing thin-walled, structurally demanding components at scale, while metal spinning is a versatile, cost-effective method suited for simpler, smaller-scale production or prototyping of rotationally symmetric metal parts. The choice between them depends on the desired part geometry, production volume, material properties, and dimensional tolerances.
Both flow forming and metal spinning rely on the rotational symmetry of the workpiece and mandrel to shape metal efficiently, but their operational philosophies diverge sharply in how they manipulate the material. Flow forming emphasizes controlled, incremental deformation using rollers that apply precise pressure to gradually reduce thickness and extend the length of a thicker blank. This controlled plastic flow, combined with CNC automation, allows the production of parts with consistent mechanical properties and tight dimensional tolerances across large batches.
In contrast, metal spinning typically involves pressing or shaping a thinner metal blank over a mandrel using handheld or semi-automated tools, relying heavily on the operator’s skill for quality and precision. The process allows quick adaptation to new shapes with minimal tooling changes, making it highly flexible and cost-effective for prototyping or custom, low-volume parts. However, because it is less controlled and often manual, metal spinning usually results in parts with less uniform wall thickness, higher variability in dimensions, and a rougher surface finish compared to flow formed components.
Material behavior during the processes also differs. Flow forming induces strain hardening and improved mechanical strength due to cold working, while metal spinning primarily reshapes the material without significant thinning or strengthening. This makes flow formed parts especially suited for applications requiring high strength-to-weight ratios, such as aerospace or defense components, where structural integrity and fatigue resistance are critical. Metal spun parts, meanwhile, are commonly used in applications where aesthetics, moderate strength, and rapid turnaround are prioritized.
Tooling complexity and cost reflect these operational differences. Flow forming demands specialized mandrels and rollers precisely engineered for each part design, which translates to higher upfront investment but consistent, repeatable output suitable for mass production. Metal spinning uses relatively simple tooling—mainly mandrels shaped to the desired profile and forming tools—which facilitates quick changeovers and lower tooling expenses, but at the cost of reduced automation and consistency.
The surface finish and post-processing requirements also vary. Flow formed parts often emerge with fine surface quality, sometimes eliminating the need for further machining or finishing. Metal spinning can leave tool marks or slight irregularities that typically require additional finishing steps like polishing or machining, particularly for applications where appearance or tight tolerances are essential.
In modern manufacturing environments, these differences influence how each process fits into production strategies. Flow forming is typically integrated into automated, high-throughput lines producing critical, high-value components. Its precision and repeatability make it ideal for parts where performance and compliance with stringent standards are non-negotiable. Metal spinning retains a valuable niche in rapid prototyping, custom fabrication, and lower-volume production where design flexibility and lower cost outweigh the need for extreme precision or high strength.
Both processes can also complement each other within a manufacturing workflow. For example, a part might be initially shaped by metal spinning to create a near-net shape quickly and inexpensively, followed by flow forming or other precision processes to achieve final dimensions, wall thickness, or mechanical properties. This hybrid approach leverages the strengths of each technique to optimize cost, quality, and production speed.
In conclusion, understanding the fundamental differences between flow forming and metal spinning helps manufacturers select the appropriate method based on the required material properties, production volume, part complexity, and budget constraints. While flow forming offers unparalleled control and strength for demanding applications, metal spinning provides unmatched flexibility and cost-effectiveness for simpler shapes and lower volumes. Together, they represent complementary solutions in the toolbox of metal forming technologies.
Looking ahead, advancements in both flow forming and metal spinning continue to blur the lines between these processes, driven by innovations in automation, materials, and process control. For flow forming, the integration of advanced CNC systems, real-time sensors, and AI-driven process optimization enables even tighter tolerances, reduced cycle times, and enhanced repeatability. These improvements expand the range of materials and geometries that can be formed, pushing flow forming into applications traditionally reserved for other high-precision manufacturing methods.
Similarly, metal spinning is benefiting from greater mechanization and computer numerical control, evolving from a largely manual craft to a more automated, repeatable process. CNC spinning machines allow for consistent control of tool paths, forces, and speeds, reducing variability and improving surface finish and dimensional accuracy. This makes metal spinning more viable for moderate volume production runs and more complex shapes, bridging the gap toward precision applications.
Material innovations also impact both processes. New alloys with improved ductility, strength, and heat resistance enable more aggressive forming at room temperature, expanding the scope of parts that can be cold formed without heating. Additionally, composite materials and hybrid metal structures challenge conventional forming techniques but open new design possibilities for lightweight, high-performance components in aerospace, automotive, and medical sectors.
Environmental and economic pressures drive both processes toward greater sustainability. Improvements in lubrication technology, energy-efficient machinery, and scrap reduction techniques reduce environmental footprints. Recycling of offcuts and swarf, along with process optimization to minimize waste, help manufacturers meet stricter regulations and corporate responsibility goals without compromising productivity or quality.
Furthermore, digital manufacturing ecosystems that connect flow forming and metal spinning operations to enterprise resource planning (ERP), quality management systems, and supply chain logistics enhance traceability and responsiveness. This integration supports just-in-time manufacturing, rapid prototyping, and mass customization, which are increasingly important in today’s fast-paced markets.
Training and workforce development evolve accordingly, with virtual and augmented reality tools providing immersive learning environments for operators and engineers. These platforms accelerate skill acquisition and enable simulation of complex scenarios, fostering expertise in both flow forming and metal spinning techniques while reducing the risks and costs associated with traditional training methods.
In summary, while flow forming and metal spinning remain distinct in their core methods and typical applications, ongoing technological, material, and digital advancements are enabling both processes to become more versatile, precise, and sustainable. Manufacturers increasingly leverage the strengths of each technique individually or in combination to meet the demands of modern engineering challenges, delivering components that balance performance, cost, and environmental considerations. This dynamic evolution ensures that both flow forming and metal spinning will continue to play vital roles in the future of metal shaping and precision manufacturing.
The Flow Forming Process Explained
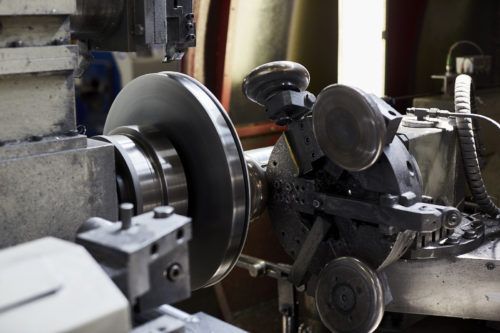
The flow forming process is a precise metal forming technique used to produce thin-walled, seamless, rotationally symmetric components, such as cylinders, tubes, or discs. It involves the controlled plastic deformation of a metal blank, typically a thick-walled cylindrical preform, over a rotating mandrel using one or more rollers that apply pressure. The primary goals are to reduce the wall thickness, increase the length, and shape the metal while maintaining or enhancing its mechanical properties.
The process begins by mounting the metal blank onto a mandrel, which serves as the internal support and defines the final inner geometry of the part. The mandrel rotates at a controlled speed while the rollers, positioned around the outside of the blank, exert force radially and axially. As the mandrel spins, the rollers press against the outer surface of the blank and move gradually along its length, causing the material to plastically flow, thin out, and elongate.
This incremental deformation transforms the original thick blank into a thin-walled, high-precision component with a smooth surface finish. The metal undergoes strain hardening during the process, which strengthens the part by increasing its yield and tensile strength. The amount of thickness reduction and elongation per pass is carefully controlled to prevent defects like cracking, wrinkling, or uneven wall thickness.
Flow forming machines are typically CNC-controlled, allowing precise adjustments to roller pressure, feed rate, and mandrel speed to optimize material flow and part geometry. The tooling consists of hardened rollers designed to conform to the desired shape and a mandrel that may be fixed or adjustable, depending on the application.
The process offers several advantages, including excellent dimensional accuracy, superior surface finish, improved mechanical properties, and efficient material usage. It is well-suited for producing aerospace components, automotive parts, pressure vessels, seamless tubes, and other precision metal parts requiring thin walls and high strength.
In summary, flow forming is a highly controlled metal forming process that transforms a thick, solid blank into a thin, seamless, and strong part by applying pressure through rollers against a rotating mandrel, combining dimensional precision with enhanced material performance.
During the flow forming process, the material experiences a gradual and controlled plastic deformation, which allows the metal to elongate and thin without compromising its structural integrity. As the rollers press and move along the rotating blank, the metal is effectively “flowed” along the mandrel surface, hence the name. This flow of metal aligns the grain structure in the direction of deformation, which often results in improved mechanical properties such as increased tensile strength and fatigue resistance compared to parts produced by casting or machining from solid stock.
The control of process parameters is critical to the success of flow forming. Variables such as roller pressure, feed rate, mandrel speed, and the number of passes must be carefully balanced. Excessive roller pressure or overly aggressive feed rates can cause defects such as cracks, wrinkles, or out-of-round sections. Conversely, too light a pressure may fail to properly form the part or result in uneven wall thickness. Operators rely on sophisticated CNC controls and sometimes real-time sensors to monitor forces and adjust parameters dynamically, ensuring consistent quality throughout production.
The tooling setup involves the mandrel, which provides internal support and defines the internal dimensions of the part, and the rollers, which apply the forming pressure externally. Mandrels may be fixed in shape for producing simple cylindrical parts or engineered with adjustable segments for forming more complex geometries like stepped diameters or tapered sections. Rollers are typically designed with hardened surfaces and specific contours to gradually shape the metal, minimizing stress concentrations and ensuring a smooth surface finish.
Because the process typically occurs at room temperature, the metal undergoes strain hardening, which increases its strength. However, this also means that the material becomes less ductile with each pass, which limits the total amount of deformation that can be achieved without intermediate annealing. In some cases, manufacturers perform multiple forming passes with controlled intermediate heat treatments to relieve stresses and restore ductility before continuing.
Flow forming is especially advantageous when producing parts that require tight tolerances, thin walls, and excellent surface finishes, making it a preferred method in high-performance industries such as aerospace, automotive, and defense. Compared to alternative methods like deep drawing or machining from solid billets, flow forming reduces material waste and lowers production costs while achieving superior part properties.
In essence, the flow forming process combines mechanical precision, material science, and advanced control technologies to convert simple metal blanks into complex, high-strength, seamless components efficiently and reliably, making it a cornerstone technique in modern manufacturing of precision tubular and cylindrical parts.
Flow forming machines vary in design depending on the specific application and part requirements. Some machines feature a single roller, while others may use multiple rollers arranged symmetrically around the workpiece to apply even pressure and reduce deformation inconsistencies. The choice of roller number and configuration impacts the achievable part geometry, surface finish, and process stability.
In addition to cylindrical parts, flow forming can produce stepped, tapered, and contoured shapes by carefully programming the mandrel geometry and roller paths. This flexibility allows manufacturers to create near-net-shape components that require minimal secondary machining, thus reducing production time and cost. Moreover, the ability to precisely control wall thickness distribution helps optimize the strength-to-weight ratio of parts, which is especially important in industries focused on lightweight design such as aerospace and automotive.
The material selection for flow forming is broad, encompassing aluminum, steel, titanium, copper, and various alloys. Each material presents unique challenges in terms of ductility, strain hardening behavior, and required forming forces. Advanced flow forming processes incorporate real-time monitoring systems that adjust parameters dynamically to accommodate these material differences, ensuring consistent quality regardless of batch or alloy variations.
While flow forming excels at producing high-quality thin-walled components, it is not without limitations. The initial cost of specialized machinery and tooling can be significant, making the process more economical for medium to high-volume production runs. Additionally, the maximum achievable wall thickness reduction per pass is constrained by material properties and machine capabilities, sometimes necessitating multiple forming passes with careful process planning.
The integration of flow forming into modern manufacturing systems often involves upstream and downstream processes such as blank preparation, heat treatment, machining, and inspection. By coordinating these steps, manufacturers create streamlined production lines that enhance throughput and traceability while maintaining high quality standards.
Overall, flow forming stands out as a sophisticated metal forming process that merges precise mechanical deformation with advanced control technologies to produce seamless, high-performance parts efficiently. Its ongoing evolution in machine design, process monitoring, and materials compatibility continues to broaden its applicability across demanding industrial sectors.
What Is Flow Forming?
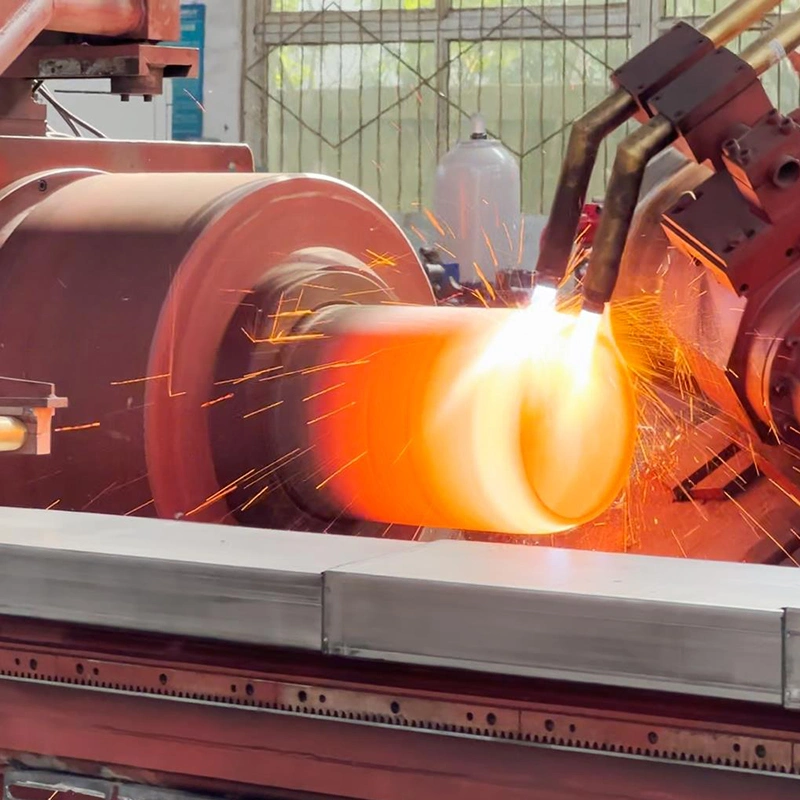
Flow forming is a specialized metal forming process used to create thin-walled, seamless, rotationally symmetrical components by plastically deforming a thicker metal blank over a rotating mandrel. In this process, one or more rollers apply controlled pressure to the outside surface of the rotating blank, causing the material to “flow” and elongate while reducing its wall thickness. The mandrel supports the inside of the workpiece, defining its internal shape and ensuring dimensional accuracy.
The key characteristic of flow forming is its ability to produce parts with uniform wall thickness, excellent surface finish, and improved mechanical properties due to strain hardening from cold working. This process is highly precise, often CNC-controlled, and is commonly used in manufacturing components like aerospace cylinders, automotive drive shafts, seamless tubes, pressure vessels, and other high-performance thin-walled parts.
Unlike other forming methods, flow forming combines gradual plastic deformation with continuous rotation, allowing efficient use of material and reducing waste compared to machining or casting. Its precision and strength enhancement make it ideal for applications requiring lightweight, durable, and seamless metal parts.
Flow forming begins with a thick metal blank, usually a cylindrical piece, mounted on a mandrel that rotates at a controlled speed. As the mandrel spins, rollers positioned around the outside of the blank press inward and move along its length, gradually reshaping the material. This controlled pressure causes the metal to plastically deform and flow along the mandrel’s surface, thinning the walls and elongating the part while maintaining its seamless integrity.
The gradual nature of the deformation is essential because it helps maintain material continuity without cracks or fractures. The metal’s grain structure aligns along the direction of deformation, which enhances the mechanical properties such as tensile strength, fatigue resistance, and toughness. These improvements are especially important in demanding applications like aerospace and automotive industries, where parts must withstand high stresses while being as lightweight as possible.
Precision control over variables like roller pressure, feed rate, and mandrel rotation speed is crucial. Too much pressure or an overly aggressive feed can cause defects such as wrinkles, uneven thickness, or even cracking. Conversely, insufficient pressure may result in incomplete forming or poor dimensional accuracy. To manage this, modern flow forming machines employ CNC controls and sometimes incorporate sensors to monitor force and displacement in real-time, enabling dynamic adjustments that maintain consistent quality throughout production.
The tooling for flow forming consists primarily of the mandrel and the rollers. The mandrel acts as the internal shaping surface, often machined to exact specifications to ensure the finished part meets tight dimensional tolerances. Some mandrels are adjustable or segmented to accommodate complex shapes, including tapered or stepped geometries. Rollers are designed to apply uniform pressure and may have specialized contours to support specific part profiles, helping to minimize stress concentrations and produce smooth surfaces.
Flow forming is typically performed at room temperature, meaning it’s a cold working process. As the metal is deformed, it hardens, which improves strength but also reduces ductility. Because of this, the total amount of deformation possible in a single pass is limited. For parts requiring significant thickness reduction, multiple passes with intermediate annealing to restore ductility may be necessary.
This process is highly efficient in terms of material usage compared to traditional machining from solid billets or casting, as it starts with a near-net-shape blank and forms it precisely to the desired dimensions. This minimizes scrap and machining time, making it cost-effective for medium to high volume production runs.
Overall, flow forming combines mechanical precision, material enhancement, and process efficiency to create high-quality, seamless thin-walled components with superior strength and finish, meeting the demanding requirements of modern engineering applications.
The advantages of flow forming extend beyond just mechanical strength and precision. Because the process is inherently material-efficient, it supports sustainability goals by reducing waste and the need for extensive post-processing. Components often come off the machine close to final shape and dimensions, requiring minimal finishing operations such as machining or polishing. This reduces not only material costs but also labor and energy consumption associated with secondary operations.
Another major benefit is the ability to tightly control wall thickness along the entire length of the part. This is critical in applications like aerospace, where weight optimization is vital and overbuilt or inconsistent sections can negatively impact performance. Engineers can design components with variable wall thickness tailored to stress profiles, optimizing strength where it’s needed while keeping the part as light as possible. This capability also contributes to more efficient use of raw materials.
In terms of flexibility, flow forming can accommodate a range of part geometries—beyond simple cylindrical forms. By customizing the mandrel shape and programming the roller path, manufacturers can produce parts with complex contours, stepped diameters, and even shallow tapers. This versatility makes flow forming suitable for an array of components like rocket motor cases, missile bodies, drive shafts, pressure vessels, gas cylinders, and specialized tubing for nuclear and oil industries.
Flow forming can be applied to a broad spectrum of metals and alloys, including aluminum, steel, titanium, and high-nickel superalloys. Each material responds differently to the forming process, requiring specific machine setups and process parameters to account for differences in strength, ductility, and strain hardening behavior. Harder or less ductile materials may require more forming passes or elevated forming temperatures, though flow forming is most commonly done as a cold working process.
Quality control in flow forming is increasingly integrated with digital monitoring and inspection tools. Sensors can track forming forces, roller positions, and workpiece temperature in real time, allowing the machine to detect deviations early and adjust automatically or alert operators before problems arise. Finished parts are often inspected with non-destructive techniques such as ultrasonic testing or laser scanning to confirm internal and external dimensions, surface finish, and material integrity.
While the initial setup cost for flow forming—especially for CNC machines and custom tooling—can be high, the long-term cost benefits become evident in mass production scenarios. The process produces consistent parts with tight tolerances and minimal rework, leading to lower rejection rates and higher throughput. Moreover, the mechanical advantages gained through cold working reduce the need for subsequent heat treatment in many cases, saving additional time and energy.
Ultimately, flow forming is a highly engineered process that bridges the gap between performance and efficiency in metal manufacturing. Its ability to create high-strength, seamless, precision components with minimal waste positions it as a valuable technique in modern industrial production, especially in sectors where structural integrity, weight, and dimensional accuracy are paramount.
Defense and Ballistics Flow Forming Equipment
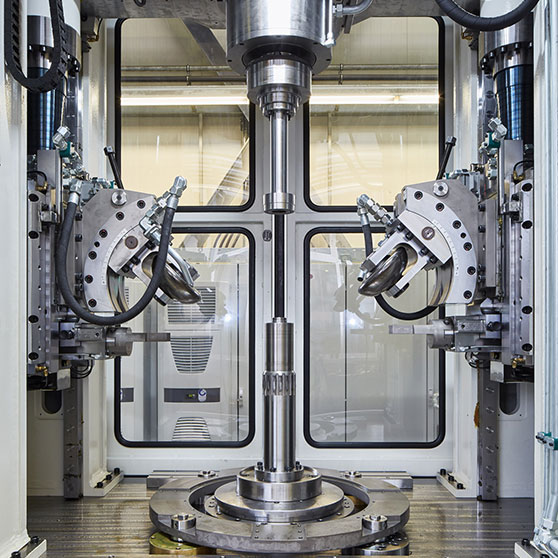
Defense and ballistics flow forming equipment is engineered to meet the demanding requirements of military and aerospace applications where strength, precision, and reliability are critical. These machines are specifically designed to manufacture components such as rocket motor cases, missile bodies, gun barrels, projectile casings, and pressure vessels used in advanced weaponry and defense systems. The process ensures that these components have seamless construction, uniform wall thickness, and enhanced mechanical properties — all vital for withstanding high pressures, dynamic stresses, and extreme environmental conditions.
Flow forming used in this context typically involves high-strength alloys such as maraging steel, titanium, and specialized aluminum alloys, chosen for their ability to maintain integrity under high-stress scenarios. Defense-grade flow forming machines often incorporate high-tonnage hydraulic or servo-controlled systems to apply the necessary forming forces while maintaining precise control over material deformation. These machines are usually fully CNC-controlled, allowing for programmable control over roller paths, forming speeds, and pressure settings to ensure consistent, repeatable quality even across complex or multi-stage components.
A key advantage in ballistics applications is the ability of flow forming to produce elongate, cylindrical structures with exceptional strength-to-weight ratios and a seamless grain structure that follows the part’s geometry. This grain alignment, a result of controlled plastic deformation, significantly improves fatigue resistance, burst strength, and impact performance — all crucial characteristics for defense equipment exposed to explosive forces or high-velocity operation.
Many defense contractors rely on custom flow forming solutions that integrate specialized tooling, adaptive control systems, and real-time process monitoring. These advanced features are critical to maintaining tight tolerances and meeting the rigorous standards imposed by defense agencies and quality certifications such as AS9100 and ITAR compliance. Integration with non-destructive testing equipment, such as ultrasonic or eddy current inspection, is also common to verify material integrity without compromising the part.
Overall, flow forming equipment for defense and ballistics serves as a cornerstone technology in the production of mission-critical hardware, combining metallurgical performance, structural precision, and process efficiency to deliver components capable of operating under the most extreme conditions.
These machines are often customized to meet specific military production needs, whether for large rocket motor housings that must endure intense combustion pressures or for smaller, high-velocity projectile bodies where uniformity and balance are critical for trajectory and accuracy. The flexibility of flow forming allows for the fabrication of components with varying wall thicknesses, integrated reinforcement features, and complex geometries — all while preserving a seamless structure that would be difficult or impossible to replicate with welding or casting.
Because flow forming is a cold-working process, the resulting strain-hardened material properties can exceed those of the base material. In defense applications, this means parts can withstand higher operational loads with improved resistance to fatigue and fracture. The absence of weld seams also eliminates common failure points, further enhancing the reliability and safety of the final components. This is particularly important for applications like gun barrels and rocket cases, where any structural weakness could lead to catastrophic failure.
In terms of production capabilities, defense and ballistics flow forming equipment must handle a wide range of diameters, lengths, and materials. Machines may be equipped with multiple forming stations, heavy-duty spindle drives, and automated loading systems to maximize throughput while maintaining quality. Precision feedback systems monitor variables such as roller force, part temperature, and rotational speed, allowing for real-time adjustments to ensure consistent material flow and dimensional accuracy. This high level of control is essential for meeting strict tolerances, especially in components that must function flawlessly under high-speed or explosive conditions.
Another key advantage of flow forming in the defense sector is its adaptability to prototyping and rapid iteration. Since the process is tool- and die-efficient, it allows engineers to modify mandrels or roller paths relatively quickly when adapting to new designs or evolving specifications. This makes it ideal for the fast-paced development cycles typical in modern defense programs, where changes in threat profiles or technology may necessitate new configurations of munitions or delivery systems.
The integration of flow forming equipment into defense manufacturing lines is often supported by strict documentation, traceability systems, and compliance frameworks. Equipment manufacturers provide extensive validation services, ensuring that each machine meets the unique specifications of the end-user and that every part formed can be traced back through the production process. This level of accountability is essential in military supply chains, where parts may be in service for years and must consistently perform to original specifications.
Flow forming’s role in defense and ballistics manufacturing is likely to grow even further as advanced materials and hybrid metal designs become more prevalent. As mission requirements demand lighter, stronger, and more efficient weapon systems, flow forming offers an unmatched combination of strength, precision, and efficiency. Its seamless results, adaptability to difficult geometries, and ability to enhance base material performance make it a cornerstone process for the next generation of defense and aerospace technology.
The continual evolution of flow forming equipment for defense applications also includes advancements in automation, data acquisition, and digital integration. Modern machines are not just mechanical systems—they function as intelligent platforms capable of capturing detailed process data for each component produced. This digital footprint allows for predictive maintenance, performance analytics, and integration into broader smart manufacturing environments, all of which are increasingly demanded in high-spec military manufacturing facilities.
Another important development is the move toward hybrid flow forming processes that combine traditional cold forming with localized heating for particularly tough or thick materials. While pure cold flow forming is preferred for its strengthening effects, warm or hot flow forming can be used strategically in areas where ductility is limited or where deeper draws are required. These hybrid methods maintain most of the mechanical benefits of cold forming while expanding the range of materials and geometries that can be successfully formed. This is particularly useful for forming large-diameter rocket motor casings, penetrator rods, or other specialized hardware that must resist extreme thermal and mechanical stresses.
Flow forming also plays a role in life-extension programs for military systems. By reproducing high-fidelity replacement parts for aging equipment, flow forming can help maintain older systems that are still strategically valuable but lack original manufacturing support. This is especially important in the context of global defense readiness, where parts availability and logistical continuity are vital.
Security and confidentiality are also key considerations in the design and use of defense-related flow forming equipment. Machines destined for military use are often subject to export controls and require compliance with strict international regulations. Manufacturers working in this space are typically experienced in handling sensitive projects and offer secure design, installation, and training services tailored to classified or proprietary programs.
Looking ahead, flow forming is expected to integrate even further with additive manufacturing and composite technologies. As metal matrix composites and other advanced materials become more common in defense systems, flow forming may be used in conjunction with additive preforms or inserts to create hybrid parts that combine the best properties of different materials. The ability to apply localized flow forming to enhance or encapsulate high-performance inserts without compromising integrity is a promising area of research.
In conclusion, flow forming equipment tailored for defense and ballistics is more than a fabrication tool—it is a strategic enabler of mission-critical hardware. Its ability to produce seamless, high-strength, precision components from advanced materials makes it indispensable in a sector where reliability, performance, and adaptability are non-negotiable. As threats evolve and systems become more complex, flow forming continues to provide the structural backbone for some of the most advanced military technologies in the world.
Flow Forming Machine for Electric Motor Housings
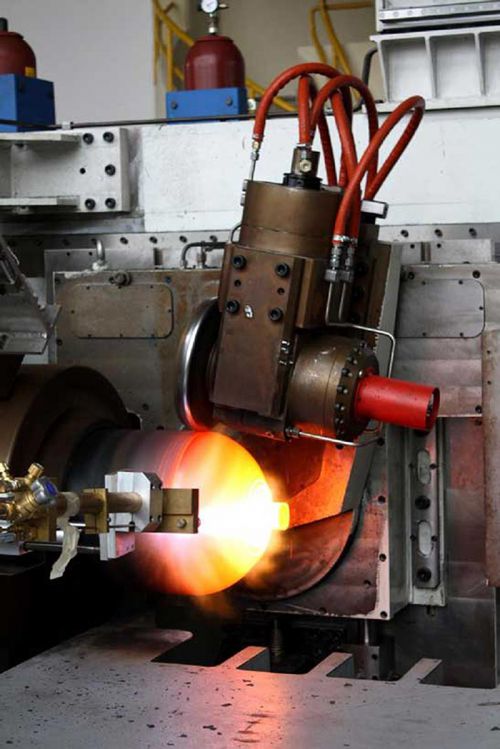
Flow forming machines for electric motor housings are engineered to produce high-precision, thin-walled, cylindrical components that meet the stringent requirements of electric mobility, industrial automation, and energy-efficient applications. These machines enable the production of seamless motor housings with exceptional dimensional accuracy, concentricity, and surface finish, all of which are critical for the performance and efficiency of electric motors. By starting with a preform or blank and plastically deforming it over a rotating mandrel using controlled roller pressure, the process ensures a uniform wall thickness and superior structural integrity, reducing the need for additional machining.
Electric motor housings made through flow forming benefit from the cold working process, which enhances the mechanical properties of the material, particularly strength and fatigue resistance. This is especially important for electric motors that operate at high rotational speeds, where any imbalance or structural inconsistency can result in vibration, noise, or premature wear. Flow forming also allows for tight tolerance control and high repeatability, making it ideal for high-volume production of motor housings across the automotive, aerospace, and industrial sectors.
Aluminum and its alloys are commonly used materials for electric motor housings due to their lightweight nature and excellent thermal conductivity. Flow forming enables efficient shaping of these materials into complex geometries, including housings with integrated cooling channels or reinforced sections for mounting components. The ability to vary wall thickness along the axial length of the housing gives engineers the freedom to reinforce stress-critical areas without adding unnecessary weight.
Modern CNC-controlled flow forming machines offer advanced features such as real-time monitoring, programmable roller paths, and automated handling systems to optimize production speed and quality. These systems can be integrated directly into manufacturing lines, supporting lean and automated motor production workflows. Additionally, flow forming minimizes material waste compared to machining from solid billets or casting, offering a more sustainable and cost-effective solution.
As electric mobility and motor-driven systems continue to grow, the demand for precision-engineered, lightweight, and thermally efficient motor housings is rising sharply. Flow forming provides a manufacturing method that not only meets these needs but also allows for design flexibility and scalable production, making it a strategic technology for the future of electric motor development.
This process is particularly well-suited for electric motor applications where balance and dimensional accuracy are critical to performance and energy efficiency. The seamless construction of flow-formed housings ensures uniform stress distribution and eliminates the weaknesses associated with welded or cast joints. This structural uniformity is essential for minimizing vibration and noise during high-speed motor operation, which is especially important in electric vehicles, aerospace actuators, robotics, and precision industrial machinery.
Another key benefit is thermal management. The tight grain structure and high surface quality achieved through flow forming improve the thermal conductivity of aluminum housings, allowing heat generated by the motor to dissipate more effectively. This contributes to longer component life and supports the integration of more compact, high-power-density motor designs. Some designs also incorporate features like integrated cooling fins or channels, which can be more easily achieved or refined with flow forming than with casting or machining.
From a production standpoint, flow forming offers high repeatability and consistency. CNC-controlled systems can produce thousands of identical motor housings with minimal variation, making it ideal for mass production in the electric vehicle industry or in large-scale automation systems. Machines can be programmed for quick changeovers between different sizes or geometries, supporting flexible manufacturing environments where multiple motor types are produced on the same line. Automation options such as robotic part loading, inline gauging, and process feedback loops further enhance productivity and quality assurance.
Tooling costs are generally lower than those of casting or forging for similar applications, particularly in short to medium production runs. Since flow forming is a near-net-shape process, the need for secondary machining is drastically reduced, which not only saves time and cost but also preserves material integrity. This is especially valuable when working with higher-cost materials like high-performance aluminum or specialty steels, where minimizing scrap is a financial advantage.
Flow forming also enables rapid prototyping and iterative design. Engineers developing new motor housings can test multiple wall thickness profiles, reinforcement patterns, or cooling features by modifying mandrels or roller paths without the long lead times associated with casting die changes. This accelerates development cycles and allows manufacturers to refine motor housing performance without sacrificing production agility.
In electric drivetrains where compact, lightweight, and robust components are key, flow-formed housings support the broader goals of improved power-to-weight ratio and system efficiency. The ability to manufacture housings that are not only lighter but also stronger and more dimensionally stable enhances overall drivetrain performance, battery life, and thermal efficiency. These advantages are increasingly important as OEMs and Tier 1 suppliers seek to differentiate their products through innovation in motor and powertrain design.
Overall, flow forming provides an advanced manufacturing pathway that aligns with the evolving requirements of electric motor technology. It combines structural precision, material efficiency, and scalable automation into a process capable of delivering superior motor housings for next-generation electric and hybrid systems. As demand increases for high-speed, high-efficiency motors across industries, the role of flow forming in enabling lightweight, high-performance housing solutions will continue to grow.
In addition to performance and production benefits, flow forming also contributes to regulatory and sustainability goals increasingly relevant in modern manufacturing. Because the process inherently reduces material waste and energy consumption—particularly when compared to subtractive machining—it aligns with global efforts to lower carbon emissions and implement greener industrial practices. The elimination of welding or extensive post-processing not only cuts down on labor and energy costs but also simplifies the supply chain, reducing the number of steps and potential points of failure in the production process.
Electric motor housings produced through flow forming are also better suited for design standardization and modularity. Manufacturers can create common base geometries across different motor models while adjusting specific features like length, mounting interfaces, or cooling elements without completely reengineering the part. This modularity enables product lines to scale efficiently and reduces inventory complexity, which is valuable for manufacturers serving multiple vehicle platforms or industrial product lines.
Another emerging trend is the integration of flow forming with complementary technologies such as additive manufacturing and precision joining. For example, a flow-formed aluminum housing might be combined with additively manufactured internal structures or cooling features that would be impossible to form through traditional means. Similarly, the precise tolerances achieved with flow forming make it easier to mate housings with stator assemblies, bearing seats, or electronic components with minimal additional alignment work. This leads to higher assembly efficiency and lower defect rates in motor production lines.
From a design standpoint, engineers are increasingly leveraging the capabilities of flow forming to push boundaries in electric motor performance. By optimizing the wall thickness and material distribution in a housing, they can tailor stiffness, weight, and thermal behavior to match specific performance targets. In high-speed applications like drone motors or advanced robotics, even minor improvements in concentricity or balance can have measurable effects on performance, making the precision of flow forming a competitive advantage.
Furthermore, flow forming allows for the creation of housings that serve multiple functions within the motor assembly. A single, flow-formed part can integrate structural, thermal, and sometimes electromagnetic shielding roles, replacing what would otherwise require multiple separate components. This simplification not only reduces weight and cost but also improves overall system reliability by eliminating interfaces and potential failure points.
As electric mobility continues to mature and as industries adopt higher levels of automation and electrification, the demand for high-performance, cost-effective, and environmentally friendly production methods like flow forming will only intensify. Manufacturers investing in flow forming equipment for electric motor housings position themselves at the forefront of this shift—able to meet tight tolerances, aggressive cost targets, and advanced engineering requirements without compromise. The adaptability, repeatability, and structural quality of flow forming will remain a cornerstone of innovation in electric motor manufacturing for years to come.
Industrial Flow Forming for Large-Scale Manufacturing
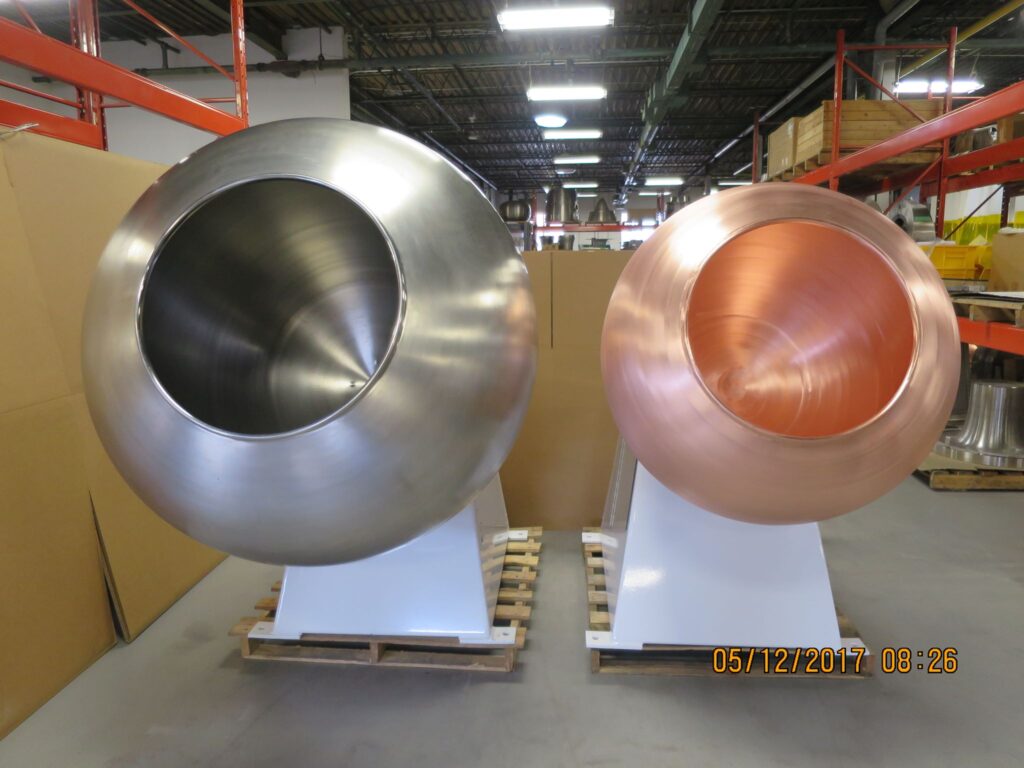
Industrial flow forming for large-scale manufacturing is a critical technology for producing high-strength, dimensionally precise, and lightweight cylindrical components across a broad range of sectors, including automotive, aerospace, energy, and heavy machinery. The process offers an ideal combination of scalability, repeatability, and material efficiency, making it especially suited for high-volume production environments where component consistency and cost control are paramount. By plastically deforming a preform or blank over a rotating mandrel using controlled rollers, flow forming achieves seamless structures with tightly controlled wall thicknesses and superior mechanical properties.
In large-scale industrial applications, the parts produced through flow forming are often subjected to high mechanical and thermal stresses. Examples include transmission parts, hydraulic cylinders, gas turbine components, pressure vessels, and structural tubes. These applications demand not only geometric accuracy but also high levels of fatigue resistance, tensile strength, and dimensional stability—requirements that flow forming meets through the inherent benefits of cold or warm plastic deformation. Unlike casting or welding, flow forming creates grain structures aligned with the part’s geometry, resulting in parts with enhanced durability and a better strength-to-weight ratio.
To support large-volume production, industrial flow forming machines are built for robustness and high duty cycles. They often feature heavy-duty hydraulic systems or servo-controlled drives capable of applying immense forming forces across long lengths and large diameters. Multi-station flow forming setups allow for simultaneous operations, reducing cycle time while maintaining precision. CNC control systems manage roller pressure, feed rates, spindle speed, and temperature conditions with real-time feedback, ensuring consistent product quality across thousands of parts.
Material efficiency is another key benefit in industrial-scale flow forming. By shaping near-net parts from tubular or cup-shaped preforms, the process minimizes scrap and reduces raw material usage compared to machining from solid billets. This not only results in lower material costs but also aligns with sustainability objectives, reducing environmental impact and supporting more resource-conscious manufacturing practices. The minimal need for post-processing and finishing also streamlines operations and reduces energy consumption across the production line.
Industrial flow forming is also highly adaptable. Machines can be configured to form parts in a wide range of materials—from mild steel and aluminum to titanium, Inconel, and maraging steels—making the process suitable for diverse application demands. Tooling changes and program adjustments allow rapid transitions between different product types, which is especially valuable in facilities producing for multiple industries or contract manufacturing environments.
Integration with modern factory automation is another advantage. Flow forming systems can be equipped with robotic part handling, inline inspection stations, and automated data logging systems for process monitoring and quality assurance. These capabilities are vital in industries with strict traceability and certification requirements, such as aerospace and automotive sectors, where each part must meet exacting standards over the course of extended production runs.
Ultimately, industrial flow forming empowers manufacturers to produce high-performance components at scale without compromising on quality, efficiency, or material performance. As manufacturing trends continue to emphasize lightweighting, durability, and process sustainability, flow forming remains a cornerstone process capable of meeting the evolving demands of large-scale production in today’s competitive industrial landscape.
In high-throughput manufacturing environments, the ability of flow forming to deliver consistent dimensional precision over long production runs is particularly valuable. Components such as drive shafts, gas bottles, suspension parts, and industrial rollers must meet exacting specifications, and even minor deviations in wall thickness or roundness can affect performance, longevity, or safety. Flow forming eliminates many of the variables associated with traditional forming or machining processes, enabling a high level of uniformity and control even when working with large, complex geometries. This precision is achieved through advanced CNC controls that monitor and adjust every aspect of the forming process, from roller positioning to rotational speeds and forming pressures.
The machines themselves are engineered for industrial reliability. They often operate in harsh conditions and are built with reinforced frames, high-torque spindles, and heavy-duty hydraulic or servo systems that can sustain 24/7 operations. Maintenance cycles are optimized to reduce downtime, with modular tooling systems allowing for quick changeovers between product types. This kind of flexibility is essential in large-scale manufacturing settings where responsiveness to shifting market demand or design updates is critical.
Industrial flow forming is also compatible with a wide range of upstream and downstream processes. Parts can be preheated, coated, or treated before forming, and the resulting components can move directly into secondary processes like CNC machining, heat treatment, or surface finishing. Because flow forming significantly reduces the amount of finishing work required, overall production time and cost are decreased. The smooth, high-integrity surfaces produced during forming are ideal for applications requiring high corrosion resistance or tight seals, further reducing the need for complex post-forming treatments.
Scalability is one of the most powerful aspects of flow forming for industrial use. A process proven at a small production scale can be expanded relatively easily by increasing machine size, adding forming stations, or integrating additional automation. As companies scale up from prototype to full production, flow forming enables a smooth transition without the need for completely new tooling or process redesigns. This scalability reduces financial risk and helps companies bring new products to market faster while maintaining quality and compliance with international manufacturing standards.
The process also supports continuous innovation. Engineers can experiment with varying wall thicknesses, complex inner and outer contours, or hybrid materials to improve part performance or reduce cost. With flow forming, these adjustments are possible without drastically increasing tooling complexity or production times. For sectors like aerospace and energy, where materials like titanium and nickel alloys are common, the ability to form parts efficiently from such tough materials provides a strategic manufacturing advantage.
Moreover, flow forming contributes to a lean manufacturing philosophy by minimizing waste, reducing energy consumption, and supporting just-in-time production strategies. In large-scale manufacturing operations, where cost efficiency, reliability, and environmental impact must be balanced, this alignment with lean principles enhances the overall competitiveness of the operation. The reduced need for inventories of finished parts, simplified logistics due to part standardization, and minimized defect rates all contribute to a more efficient and resilient production model.
As global industries continue to push toward more advanced, lightweight, and high-strength designs, industrial flow forming stands out as a proven, scalable, and efficient method for producing components that meet the highest standards. Whether producing tens of thousands of identical parts or a wide variety of customized components, flow forming delivers the performance, precision, and productivity required for success in modern manufacturing.
The strategic adoption of flow forming in large-scale industrial manufacturing also plays a role in addressing workforce challenges by reducing reliance on highly specialized manual labor. With increasingly automated and CNC-controlled systems, the process becomes less dependent on operator expertise and more on digital programming and system integration, allowing for easier training, better consistency, and reduced human error. This shift supports modern workforce dynamics, where skilled labor shortages are common and manufacturers must do more with fewer specialized technicians.
Moreover, flow forming’s compatibility with digital manufacturing ecosystems allows it to be fully integrated into smart factory setups. Real-time data collection from sensors embedded in rollers, spindles, and drives enables predictive maintenance and continuous process optimization. Production metrics such as wall thickness, concentricity, material temperature, and roller force can be monitored and adjusted automatically, reducing scrap rates and improving overall equipment effectiveness (OEE). These capabilities make flow forming a key technology in Industry 4.0 environments where traceability, efficiency, and data-driven quality control are standard expectations.
From a supply chain perspective, the reliability and repeatability of flow forming reduce the risk of quality deviations that might otherwise cause production stoppages or customer rejections. This process stability enhances the ability of manufacturers to meet strict delivery schedules and maintain long-term supplier agreements. In industries like automotive, where just-in-time manufacturing is critical and tolerances are tight, the ability to repeatedly produce thousands of identical parts with minimal variation becomes a decisive advantage. It also opens up opportunities for strategic partnerships and preferred supplier status in high-volume contracts.
Environmental sustainability is becoming an increasingly decisive factor in manufacturing decisions, and flow forming supports these goals through its inherent efficiency. The process generates significantly less waste than subtractive methods, particularly when using expensive or difficult-to-machine alloys. Energy usage is lower because the deformation is localized and continuous rather than interrupted, as in forging or stamping. The ability to form at room or near-room temperatures also cuts down on the emissions and energy costs associated with heat-intensive processes, especially when forming steel or aluminum.
Looking to the future, the role of industrial flow forming is set to expand further as new materials and product applications emerge. The shift to electrification in transportation, increased investment in renewable energy infrastructure, and the continued miniaturization and performance enhancement of mechanical systems all demand lightweight, high-strength components at scale. Flow forming is uniquely positioned to meet these needs, not just because of its technical capabilities, but because of its adaptability to evolving design and manufacturing requirements. It is a method that bridges traditional industrial craftsmanship and next-generation production, enabling companies to scale intelligently without sacrificing quality or innovation.
With its unmatched combination of strength, precision, efficiency, and scalability, flow forming continues to redefine what is possible in large-scale industrial manufacturing. It empowers manufacturers to meet growing demand with tighter tolerances, higher performance materials, and reduced environmental impact—all while lowering costs and shortening lead times. As industries evolve, flow forming remains an essential technology for companies seeking to stay competitive in a landscape defined by complexity, speed, and precision.
Flow Forming for Medical Device Production
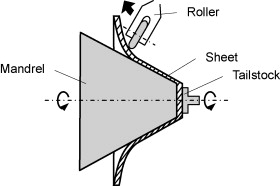
Flow forming for medical device production is an advanced manufacturing process that enables the creation of precise, seamless, and thin-walled components essential to many medical applications. The medical industry demands components with exceptional accuracy, surface finish, biocompatibility, and mechanical integrity, all of which flow forming can provide by plastically deforming metal blanks over mandrels with controlled rollers. This process is particularly well-suited for producing parts such as surgical instrument shafts, implant casings, orthopedic device components, and housings for diagnostic equipment.
The seamless nature of flow-formed parts eliminates welds or joints that could harbor bacteria or weaken the structure, which is critical in maintaining sterility and durability in medical devices. Additionally, the process can produce complex geometries with tight tolerances and consistent wall thicknesses, allowing medical device manufacturers to meet stringent regulatory standards imposed by bodies such as the FDA or ISO. The ability to maintain dimensional stability throughout production also supports the high repeatability required for batch manufacturing of disposable or implantable components.
Materials commonly used in medical flow forming include stainless steel, titanium, cobalt-chromium alloys, and specialized biocompatible materials. Flow forming enhances the mechanical properties of these metals, such as strength and fatigue resistance, through cold working, which is vital for devices that undergo repetitive stress, such as orthopedic implants or minimally invasive surgical tools. The improved surface finish reduces the need for extensive polishing or coating, streamlining the production process while ensuring patient safety.
Modern CNC flow forming machines equipped with precise control systems allow for the manufacturing of micro-scale or miniature components used in catheter shafts, endoscopic tools, or needle housings. The process’s adaptability to small diameters and thin walls makes it ideal for these delicate applications where flexibility, strength, and accuracy are paramount. Additionally, flow forming supports multi-stage forming sequences to create components with variable wall thickness or integrated features, reducing the number of assembly steps and improving device reliability.
Flow forming also contributes to cost efficiency and sustainability in medical device production. Near-net-shape forming reduces material waste compared to machining from solid billets, which is particularly important when working with expensive or rare metals. The process is energy efficient and can be integrated into automated production lines, supporting the high-volume manufacturing demands of disposable medical products or implantable devices.
In summary, flow forming offers medical device manufacturers a high-precision, cost-effective, and reliable method for producing complex, biocompatible components. Its combination of material integrity, dimensional accuracy, and scalability makes it an increasingly important technology in the development and production of next-generation medical devices that require both performance and safety.
Flow forming’s ability to produce seamless, thin-walled components with exceptional dimensional accuracy directly addresses the critical needs of the medical industry, where device reliability and patient safety are paramount. Because the process eliminates welds or joints, it reduces potential points of failure or contamination, which is crucial in applications such as implantable devices or surgical instruments that must withstand harsh sterilization environments and rigorous mechanical stresses. The uniform wall thickness achieved through flow forming ensures consistent mechanical properties throughout the component, enhancing fatigue resistance and longevity—key factors in devices implanted long-term or used repeatedly.
The precision control offered by modern CNC flow forming equipment allows manufacturers to meet the tight tolerances required for complex medical device geometries. This is particularly important for minimally invasive surgical tools or catheters, where even slight dimensional deviations can affect functionality or patient comfort. Flow forming can also accommodate features like variable wall thickness, integrated mounting points, or internal channels within a single part, reducing assembly complexity and improving overall device performance. This integration capability helps streamline production workflows and minimizes the risk of assembly errors or component mismatch.
Material selection is critical in medical applications, and flow forming supports a wide range of biocompatible metals, including titanium alloys favored for their strength-to-weight ratio and corrosion resistance, as well as stainless steel and cobalt-chromium alloys known for their durability and bioinert properties. The cold working nature of flow forming improves these materials’ mechanical properties without introducing thermal stresses or microstructural defects that can occur in other forming processes. This enhances implant performance, particularly in load-bearing applications such as orthopedic joint replacements or spinal implants, where strength and reliability are essential.
In addition to mechanical and material benefits, flow forming supports the increasingly stringent regulatory requirements governing medical device manufacturing. The process’s repeatability and ability to produce consistent parts facilitate validation, documentation, and traceability, which are essential for compliance with FDA, ISO, and other international standards. Automated CNC flow forming lines can incorporate real-time monitoring and data capture, enabling comprehensive quality control and rapid identification of deviations, thereby reducing waste and ensuring that only components meeting exact specifications proceed through production.
Cost efficiency and sustainability are also enhanced through flow forming in medical device production. By forming parts near net shape, the process drastically reduces the amount of expensive raw material wasted during machining or grinding operations. This is particularly valuable when working with high-cost biocompatible metals, which can represent a significant portion of device production costs. Additionally, the reduced need for post-processing and finishing lowers labor, tooling wear, and energy consumption, supporting both economic and environmental goals.
The flexibility of flow forming enables rapid prototyping and iterative design changes, which is beneficial in a medical device development environment where product specifications often evolve through clinical feedback or technological advances. Changes to part dimensions, wall thickness profiles, or integrated features can be implemented quickly by adjusting CNC programs or tooling, accelerating time-to-market and enabling manufacturers to respond effectively to emerging clinical needs or regulatory updates.
Overall, flow forming stands as a vital manufacturing technology in the medical field, delivering the precision, durability, and efficiency necessary to produce advanced medical devices. Its capacity to combine complex geometries with high-quality material properties while maintaining regulatory compliance makes it an indispensable tool for manufacturers aiming to innovate and excel in this demanding sector.
Beyond its core manufacturing advantages, flow forming also plays a role in advancing emerging medical technologies that demand increasingly sophisticated component designs. For example, as implantable devices become smaller, more complex, and multifunctional—such as drug delivery systems, neurostimulation leads, or implantable sensors—the ability to produce parts with intricate internal features, ultra-thin walls, and precise dimensional control becomes essential. Flow forming’s adaptability allows manufacturers to meet these exacting design challenges while maintaining structural integrity and biocompatibility.
Furthermore, the seamless and smooth surfaces produced by flow forming reduce the need for additional polishing or surface treatments, which can be critical in preventing bacterial adhesion and biofilm formation on surgical instruments or implants. This surface quality not only enhances patient safety but also reduces manufacturing time and costs associated with finishing operations. When combined with advanced surface coatings, such as antimicrobial or wear-resistant layers, flow-formed components provide a strong foundation for next-generation medical device performance.
Flow forming’s compatibility with automation and integration into smart manufacturing environments also supports the trend toward personalized medicine and small-batch production. Custom or patient-specific implants, tailored surgical tools, or bespoke diagnostic device housings can be efficiently produced with minimal tooling changes or setup time, helping to bridge the gap between high precision and customization. The digital nature of CNC control enables rapid adaptation of production parameters to meet unique design requirements, enhancing responsiveness to individual patient needs.
In addition, flow forming supports rigorous quality assurance practices through its repeatability and process control. Inline inspection systems, such as laser scanners or ultrasonic sensors, can be incorporated to monitor each part as it is formed, ensuring that defects are detected immediately and quality standards are maintained without slowing production. This real-time feedback loop enhances yield and minimizes scrap, which is particularly important when working with expensive biocompatible materials.
The medical device industry’s push toward sustainability and resource efficiency also benefits from flow forming technology. By maximizing material utilization and minimizing waste, manufacturers reduce their environmental footprint. The process’s lower energy consumption compared to forging or extensive machining further contributes to greener manufacturing practices, aligning with broader industry goals to reduce carbon emissions and promote circular economy principles.
As medical devices continue to evolve in complexity and function, the versatility and precision of flow forming will remain key enablers of innovation. Whether producing high-strength orthopedic implants, delicate endoscopic instruments, or custom diagnostic housings, flow forming offers manufacturers a reliable, efficient, and scalable method to meet the challenges of modern healthcare technology. Its combination of material enhancement, dimensional accuracy, and process efficiency ensures that medical devices can be produced safely, cost-effectively, and in compliance with stringent regulatory standards—ultimately supporting improved patient outcomes and advancing the future of medicine.
Oil & Gas Flow Forming Solutions
Flow forming solutions for the oil and gas industry address the demanding requirements for high-strength, corrosion-resistant, and dimensionally precise components used in exploration, extraction, processing, and transportation of hydrocarbons. Components such as tubular parts, pressure vessels, pipeline fittings, valve bodies, and compressor shells must withstand extreme pressures, harsh environments, and cyclic loads. Flow forming’s ability to produce seamless, uniform, and high-integrity parts with optimized wall thickness and excellent mechanical properties makes it an ideal manufacturing process for these critical oil and gas applications.
In the oil and gas sector, flow forming enhances the structural integrity of components by refining the grain structure and inducing beneficial residual stresses through cold or warm plastic deformation. This results in improved fatigue resistance, tensile strength, and impact toughness—properties essential for parts subjected to high-pressure service and cyclic mechanical stress in subsea environments or drilling operations. Unlike welded or cast components, flow-formed parts have no joints or seams that can act as initiation points for cracks or corrosion, greatly improving reliability and service life.
Flow forming equipment designed for oil and gas applications is capable of handling large diameters and long lengths, reflecting the scale of components required in pipelines, risers, or pressure vessels. Heavy-duty CNC-controlled machines apply precise roller forces and controlled feed rates to form materials such as carbon steel, stainless steel, duplex alloys, and nickel-based superalloys, all common in oil and gas infrastructure. This versatility allows manufacturers to produce a wide range of parts, from thick-walled tubing to thin-walled shells, optimized for specific pressure ratings and environmental conditions.
Material efficiency is particularly important given the high cost of specialty alloys used in harsh oilfield environments. Flow forming enables near-net-shape production, minimizing material waste compared to machining from solid billets or extensive welding fabrication. This not only reduces raw material costs but also lowers energy consumption and production time, contributing to more sustainable and cost-effective manufacturing practices in a highly competitive industry.
The process’s repeatability and precision are critical for meeting the stringent standards and certifications required in oil and gas manufacturing, such as API, ASME, and ISO standards. Components produced through flow forming can achieve tight dimensional tolerances and superior surface finishes, reducing the need for extensive machining or finishing. This consistency ensures proper fit, sealing, and performance in complex assemblies like valve bodies or compressor housings, where even minor deviations could compromise safety or efficiency.
Flow forming’s ability to integrate complex features into a single seamless part—such as tapered sections, flanges, or reinforced areas—simplifies assembly and improves component performance. This capability reduces the number of welds or joints, which are potential failure points under corrosive or high-pressure conditions. The enhanced fatigue resistance and corrosion performance of flow-formed parts are especially valuable in offshore and subsea applications, where maintenance or replacement is costly and difficult.
In addition, flow forming equipment used in oil and gas can be configured for both cold and warm forming processes, allowing manufacturers to optimize forming parameters based on material properties and component design. Warm flow forming, for instance, enables easier deformation of tough alloys without compromising mechanical properties, facilitating the production of high-performance components with intricate geometries or tight tolerances.
Automation and real-time process monitoring are increasingly integrated into industrial flow forming lines for oil and gas manufacturing. Inline sensors and CNC controls enable precise adjustments to forming pressure, speed, and temperature, ensuring consistent quality and early detection of defects. This is crucial in meeting the rigorous quality assurance protocols demanded by the industry and helps reduce scrap and rework costs.
Ultimately, flow forming solutions for the oil and gas industry provide a robust, efficient, and flexible manufacturing method for producing high-performance components capable of withstanding the demanding conditions encountered throughout the hydrocarbon supply chain. By combining material efficiency, mechanical strength, and process precision, flow forming supports safer, more reliable, and cost-effective operations in one of the world’s most challenging industrial sectors.
Flow forming’s contribution to the oil and gas sector extends beyond component quality and efficiency to include significant improvements in supply chain responsiveness and operational flexibility. Given the cyclical nature of oil markets and the often urgent demand for replacement parts or upgrades to infrastructure, the ability to rapidly produce customized or small-batch components without extensive retooling is invaluable. Flow forming machines, especially those equipped with CNC controls, allow manufacturers to quickly adjust forming parameters, tooling, and part dimensions, enabling faster turnaround times that help operators minimize downtime and maintain continuous production.
The seamless construction of flow-formed components also reduces inspection and certification complexities, which are critical in an industry where safety and regulatory compliance are paramount. Parts made with fewer joints and welds require less non-destructive testing and have a lower likelihood of failure during service, thus enhancing overall system reliability and reducing the risk of costly accidents or environmental damage. This reliability is especially important in subsea and deepwater applications, where repair or replacement involves significant logistical challenges and expense.
In upstream operations such as drilling and well completion, flow-formed tubular components provide superior strength-to-weight ratios, improving handling and installation efficiency while maintaining resistance to the corrosive fluids and high pressures encountered underground. Similarly, flow-formed parts used in midstream and downstream equipment, such as compressors, separators, and heat exchangers, benefit from enhanced dimensional control and surface quality, which contribute to improved sealing and operational efficiency.
The adaptability of flow forming also supports the ongoing transition toward cleaner energy and more sustainable practices within the oil and gas industry. For example, the production of parts for equipment used in natural gas processing, carbon capture, and renewable energy hybrids can benefit from the same precision and material efficiency offered by flow forming. This positions flow forming as a forward-looking manufacturing technology that not only meets current demands but also aligns with the sector’s evolving environmental and technological goals.
By integrating flow forming technology into their production capabilities, oil and gas manufacturers and service providers can achieve a competitive edge through reduced lead times, improved product performance, and lower manufacturing costs. The combination of high-strength, seamless components with flexible and efficient production processes supports safer, more durable equipment that withstands the harshest environments and extends operational life cycles.
Overall, flow forming represents a critical enabler of innovation and reliability within the oil and gas industry, providing manufacturing solutions that address the sector’s unique challenges while supporting its drive toward enhanced safety, sustainability, and economic efficiency. As demands grow for more advanced materials and complex part designs, flow forming’s precision, scalability, and material optimization will continue to play a vital role in meeting the future needs of this essential global industry.
Flow forming also plays a key role in supporting the evolving demands of enhanced oil recovery (EOR) techniques and the development of advanced drilling technologies. Components such as downhole tools, pump housings, and casings must endure aggressive chemical exposure, high pressures, and extreme temperatures. Flow forming allows manufacturers to produce parts with tailored mechanical properties and precise dimensional control, improving their ability to withstand these conditions while maintaining integrity over extended operational periods.
In the realm of offshore drilling, where environmental and safety standards are among the strictest, flow forming contributes to reducing the risk of component failure. The seamless nature of flow-formed parts eliminates stress concentrators associated with welds and joints, which are potential sites for fatigue cracks or corrosion-induced damage. This reliability is critical for equipment such as blowout preventers, risers, and subsea connectors, where failure could have catastrophic consequences both in terms of safety and environmental impact.
The use of high-performance alloys formed through flow forming also supports the industry’s move toward deeper and more challenging wells. Materials like Inconel, Hastelloy, and duplex stainless steels, which offer superior corrosion resistance and strength, can be difficult to machine or weld without compromising their properties. Flow forming’s cold or warm deformation process preserves or enhances these material characteristics, enabling the production of robust components capable of performing reliably under extreme service conditions.
In addition to component manufacturing, flow forming technologies facilitate the production of repair parts and retrofits for aging infrastructure, extending the lifespan of critical assets and delaying costly replacements. This is especially relevant in mature oil and gas fields where maintenance and refurbishment play a significant role in sustaining production. The process’s flexibility to create parts with precise dimensions and mechanical properties ensures that repairs can restore or even improve original equipment performance.
Flow forming’s integration with advanced digital manufacturing platforms and Industry 4.0 concepts is increasingly important for the oil and gas sector. Real-time monitoring, data analytics, and predictive maintenance enable manufacturers to optimize forming processes, reduce waste, and improve product consistency. This digital transformation supports tighter control over quality and traceability, both of which are vital for compliance with evolving regulatory frameworks and for building trust with clients and stakeholders.
Ultimately, the continued advancement of flow forming technology, including improvements in machine capabilities, tooling materials, and process control, will expand its applications within the oil and gas industry. The combination of superior material properties, precision manufacturing, and operational efficiency positions flow forming as a cornerstone technology that addresses both current industry challenges and future innovation needs. By enabling the production of stronger, more reliable, and cost-effective components, flow forming helps ensure that oil and gas operations can continue to meet global energy demands safely and sustainably.
Flow Forming in Military Manufacturing
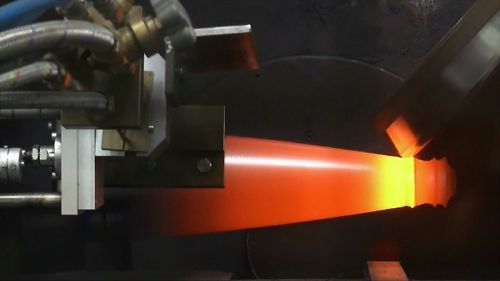
Flow forming in military manufacturing plays a crucial role in producing high-performance, durable, and precise components essential for defense applications. Military equipment and weaponry demand parts that can withstand extreme conditions, including high stresses, rapid temperature changes, and exposure to harsh environments. Flow forming’s ability to produce seamless, thin-walled components with excellent mechanical properties makes it an ideal process for manufacturing critical defense components such as artillery shells, missile casings, armored vehicle parts, and aerospace components.
The seamless construction achieved through flow forming eliminates welds or joints that can serve as weak points under high-impact or explosive forces, enhancing the structural integrity and reliability of military parts. Components produced via flow forming exhibit superior fatigue resistance and strength due to the work-hardening effect imparted by the plastic deformation of the metal during forming. This durability is vital for applications like projectile bodies, where failure could have catastrophic consequences.
Flow forming machines used in military manufacturing are capable of working with advanced alloys such as high-strength steels, titanium, and aluminum alloys that balance weight, strength, and corrosion resistance. These materials are often difficult to machine or weld without compromising their properties, but flow forming preserves and enhances their mechanical characteristics through controlled deformation. This makes it possible to create lightweight yet robust components, which is essential for improving mobility and performance in military vehicles and aircraft.
Precision is another significant advantage of flow forming in defense production. CNC-controlled flow forming machines can achieve tight tolerances and complex geometries required for sophisticated military hardware, including guidance system housings, gun barrels, and structural frames. The high repeatability of the process supports consistent quality, which is critical for the safety and effectiveness of military equipment.
In addition to component manufacturing, flow forming supports rapid prototyping and small-batch production, enabling defense contractors to quickly develop and test new designs or customized parts. This agility is important in responding to evolving defense requirements and integrating emerging technologies such as advanced sensors, communications equipment, or lightweight armor systems.
Flow forming also contributes to cost efficiency and material optimization in military manufacturing. Near-net-shape forming reduces material waste and machining time, which is especially beneficial when working with expensive or strategic materials. The process’s energy efficiency and reduced tooling wear further lower production costs, helping defense programs manage budgets while maintaining high standards.
Moreover, the robust nature of flow-formed components enhances their survivability in extreme battlefield conditions, including shocks, vibrations, and corrosive environments. This reliability reduces maintenance needs and extends service life, providing operational advantages in the field.
Overall, flow forming is a vital technology in military manufacturing, delivering components that combine strength, precision, and durability. Its ability to handle advanced materials, produce complex shapes, and maintain consistent quality supports the development of cutting-edge defense systems that meet stringent performance and safety standards essential for national security.
Flow forming’s impact on military manufacturing extends into the production of critical aerospace components used in defense aircraft and unmanned aerial vehicles (UAVs). Lightweight, high-strength parts such as structural frames, engine casings, and fuel tanks benefit from flow forming’s ability to reduce weight without sacrificing mechanical integrity. This weight reduction improves fuel efficiency, maneuverability, and payload capacity, which are vital factors in modern military aviation. Additionally, flow forming enables the creation of complex geometries that integrate multiple functions into single components, reducing assembly complexity and enhancing overall system reliability.
The process also supports the manufacture of precision components for missile and rocket systems, where exact dimensions and flawless surface finishes are mandatory to ensure aerodynamic stability and optimal performance. Flow forming minimizes material inconsistencies and surface defects, which can affect trajectory or structural strength, thus increasing the reliability and effectiveness of these high-stakes defense technologies.
In armored vehicle manufacturing, flow-formed parts contribute to improved protection and mobility. Seamless armor components and chassis elements produced through flow forming combine toughness and reduced weight, enabling vehicles to better withstand ballistic impacts while maintaining speed and agility on the battlefield. The process also allows for the production of large, durable parts that would otherwise require multiple welds or complex assemblies, thereby reducing potential failure points and simplifying logistics.
Flow forming’s flexibility in production volume is particularly advantageous for military supply chains, which often require rapid response capabilities and adaptability to changing demands. Whether producing large batches of standard parts or specialized components for limited-run projects, flow forming machines can be quickly reprogrammed and retooled, facilitating efficient transitions between different product lines or mission-specific equipment.
The technology’s role in sustainability is gaining attention in military manufacturing as well. By minimizing material waste and energy consumption, flow forming aligns with defense agencies’ increasing focus on reducing environmental impact and operational costs. The process’s precision reduces the need for secondary machining and finishing, further decreasing resource use and production timelines.
Quality assurance and traceability are paramount in defense applications, and flow forming integrates well with modern inspection and monitoring technologies. Inline sensors, automated measurements, and digital documentation enable stringent control over each component’s dimensional accuracy and mechanical properties. This level of oversight ensures compliance with military specifications and standards, while facilitating certification processes and lifecycle management.
As defense technologies continue to advance, the demand for components with enhanced performance characteristics—such as improved fatigue resistance, corrosion protection, and thermal stability—will grow. Flow forming is well-positioned to meet these challenges through ongoing innovations in tooling, materials science, and process automation. The ability to form increasingly complex, high-performance parts with consistency and efficiency supports the military’s pursuit of cutting-edge capabilities and mission readiness.
In summary, flow forming is integral to military manufacturing because it delivers high-strength, lightweight, and precisely engineered components that enhance the performance, durability, and reliability of defense systems. Its adaptability, cost-effectiveness, and compatibility with advanced materials make it an essential technology for producing the sophisticated equipment necessary to meet modern defense challenges and ensure national security.
Flow forming also plays a pivotal role in advancing the development and production of emerging military technologies, such as directed energy weapons, hypersonic vehicles, and next-generation radar systems. These technologies require components with exceptional dimensional precision, thermal stability, and structural integrity—requirements that flow forming is uniquely capable of meeting. For example, the process can create lightweight, high-strength housings and cooling channels that manage the intense heat loads generated by laser or microwave-based weaponry, ensuring consistent performance in extreme operational conditions.
In the rapidly growing field of unmanned systems and autonomous military platforms, flow forming supports the manufacture of compact, durable parts that enable longer mission endurance and enhanced payload capacity. UAV fuselages, sensor mounts, and propulsion system components benefit from the strength-to-weight advantages and tight tolerances that flow forming delivers, contributing to improved flight stability, stealth characteristics, and mission flexibility.
Flow forming’s ability to produce complex, seamless shapes with integrated features also facilitates miniaturization and multifunctionality—key trends in military hardware design. This capability reduces the number of individual parts and assembly steps, which not only decreases overall system weight and size but also improves reliability by reducing potential failure points. These advantages are crucial for portable or wearable defense equipment, where space and weight savings directly enhance soldier effectiveness and mobility.
From a logistical and operational standpoint, the durability and consistency of flow-formed parts reduce maintenance frequency and extend equipment service life, leading to lower lifecycle costs and greater readiness. The process also supports the rapid production of replacement parts in the field or at forward operating bases, enabling quicker repairs and minimizing downtime during critical missions.
Collaboration between flow forming technology providers, materials scientists, and defense engineers continues to push the boundaries of what is possible, exploring new alloys, hybrid materials, and process parameters to tailor components for specific military applications. Innovations such as additive manufacturing-assisted tooling, advanced lubrication techniques, and real-time adaptive control systems enhance flow forming’s precision and expand its application scope.
Furthermore, flow forming aligns with the defense sector’s increasing emphasis on digital manufacturing ecosystems, where data-driven production and integrated supply chains improve transparency, quality control, and responsiveness. This integration supports secure, traceable manufacturing processes vital for safeguarding sensitive military technologies and complying with stringent regulatory requirements.
In essence, flow forming is more than just a manufacturing method—it is a strategic enabler that underpins the evolution of military capabilities by delivering components that meet the rigorous demands of modern defense systems. Its combination of strength, precision, efficiency, and adaptability ensures that military manufacturers can respond effectively to emerging threats and technological advancements, maintaining superiority on the battlefield while managing costs and resources prudently.
Automotive Applications of Flow Forming
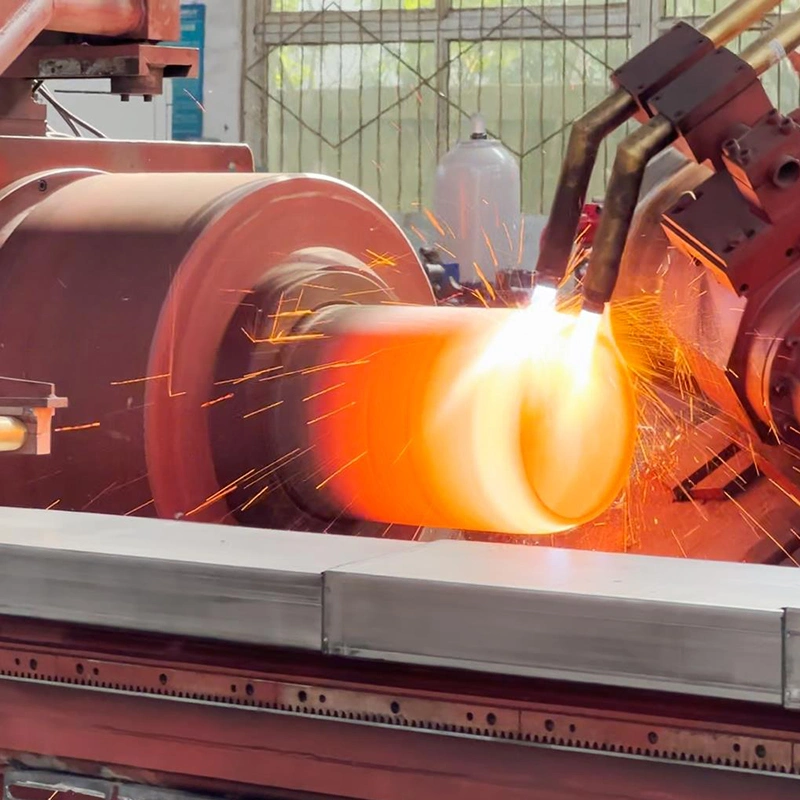
Flow forming has become an important manufacturing process in the automotive industry, prized for its ability to produce high-quality, lightweight, and precise components that meet the performance and efficiency demands of modern vehicles. This metal forming technique is especially valued for parts requiring enhanced strength-to-weight ratios, tight tolerances, and seamless construction, which contribute to improved vehicle safety, fuel efficiency, and durability.
One of the most common automotive applications of flow forming is the production of wheels, especially alloy and performance wheels. Flow forming allows manufacturers to create wheels with thinner walls and lighter weight compared to traditional cast or forged wheels, without compromising strength or structural integrity. The process refines the grain structure of the metal through plastic deformation, resulting in enhanced fatigue resistance and impact toughness—qualities critical for wheels subjected to constant stresses from road conditions and driving maneuvers.
Beyond wheels, flow forming is widely used in manufacturing drivetrain components such as gears, clutch plates, and transmission parts. These components benefit from the precise dimensional control and improved mechanical properties that flow forming imparts, enabling smoother operation, greater load capacity, and longer service life. The seamless nature of flow-formed parts reduces the risk of stress concentrations and failure points, enhancing reliability under high torque and dynamic loading conditions.
Flow forming also plays a significant role in producing structural components like drive shafts, axle tubes, and suspension parts. The ability to form thin-walled, hollow components with uniform wall thickness enables weight reduction without sacrificing strength, contributing directly to overall vehicle weight savings and improved fuel economy. Additionally, flow forming’s tight tolerances help ensure proper fit and alignment within complex assemblies, supporting vehicle stability and handling performance.
In the realm of engine and exhaust systems, flow forming is utilized to manufacture parts such as turbocharger housings, compressor wheels, and exhaust tubing. The process allows for the creation of complex shapes with smooth surfaces that improve airflow and thermal performance, enhancing engine efficiency and emissions control. Materials commonly used include aluminum, stainless steel, and high-temperature alloys, all of which retain or improve their mechanical properties through the flow forming process.
Flow forming’s near-net-shape capability reduces material waste and machining requirements, which is especially beneficial when working with costly or lightweight materials like aluminum and titanium. This efficiency not only lowers production costs but also supports sustainability initiatives within the automotive sector by minimizing raw material usage and energy consumption.
With the automotive industry’s growing focus on electric vehicles (EVs), flow forming is becoming increasingly important for producing lightweight, high-strength components such as battery housings, motor casings, and cooling system parts. These components must meet stringent requirements for thermal management, structural rigidity, and electromagnetic shielding—all achievable through the precision and material optimization offered by flow forming.
The flexibility of CNC-controlled flow forming machines allows manufacturers to adapt quickly to evolving vehicle designs and production volumes. This agility supports both mass production of standard parts and limited runs of specialty or performance components, enabling automakers to respond to market demands efficiently.
In summary, flow forming is a vital technology in automotive manufacturing, enabling the production of lightweight, strong, and precise components that enhance vehicle performance, safety, and efficiency. Its ability to work with advanced materials, reduce waste, and produce complex geometries makes it indispensable as the automotive industry continues to innovate toward cleaner, lighter, and more reliable vehicles.
Flow forming further contributes to the automotive industry by enabling the production of components with superior surface finishes and consistent wall thicknesses, which are essential for both aesthetic appeal and functional performance. For example, in the manufacturing of wheels, the smooth surface finish achievable through flow forming reduces aerodynamic drag and improves the visual quality of the final product. This is especially important for high-end and performance vehicles where both form and function are critical.
The process also supports the production of safety-critical parts such as roll cage tubing and crash management components. Flow-formed tubing offers high strength and ductility, allowing it to absorb and dissipate energy effectively during impacts. This enhances occupant protection and helps vehicles meet increasingly stringent safety regulations without adding unnecessary weight.
Flow forming is highly adaptable to various metals commonly used in automotive manufacturing, including aluminum alloys for lightweight parts, high-strength steels for structural components, and specialty alloys designed for heat resistance or corrosion protection. This material versatility enables automakers to optimize each component according to its specific function, balancing cost, weight, and durability.
The reduced need for secondary machining operations after flow forming not only speeds up production but also lowers manufacturing costs and decreases lead times. This efficiency is particularly beneficial for just-in-time production models that many automotive manufacturers rely on to reduce inventory and improve responsiveness to market changes.
Moreover, the integration of flow forming with other manufacturing techniques such as hydroforming, forging, and additive manufacturing expands design possibilities and allows for hybrid components that leverage the strengths of multiple processes. For instance, a flow-formed tube might be combined with a forged flange to create a part that maximizes strength where needed while maintaining lightweight characteristics elsewhere.
As automotive technologies evolve, including the development of autonomous vehicles and advanced driver-assistance systems (ADAS), flow forming will continue to support the production of components that meet the high precision and reliability standards these systems require. Sensor housings, camera mounts, and radar enclosures benefit from the dimensional accuracy and strength that flow forming provides, ensuring consistent performance under various environmental conditions.
Sustainability goals within the automotive industry also align well with the benefits of flow forming. By minimizing waste and energy use, flow forming helps reduce the environmental footprint of vehicle production. Additionally, the longer lifespan and enhanced durability of flow-formed parts contribute to vehicle longevity, reducing the frequency of repairs or replacements and thereby supporting circular economy initiatives.
In conclusion, flow forming’s combination of strength, precision, material efficiency, and design flexibility makes it a cornerstone technology in automotive manufacturing. It enables the production of components that not only meet demanding performance and safety requirements but also support industry-wide goals for sustainability, cost-effectiveness, and innovation. As vehicles become increasingly complex and specialized, flow forming will remain a key enabler in delivering the advanced parts needed for the next generation of automotive technologies.
Flow forming is also playing an increasingly important role in aftermarket automotive parts and motorsports applications, where the demand for high-performance, custom components is especially strong. In these sectors, flow forming allows for the rapid production of lightweight, high-strength parts that can be tailored to specific performance goals or vehicle models. Custom wheels, high-performance drive shafts, and exhaust components manufactured through flow forming offer enhanced durability and weight savings that directly translate into improved acceleration, handling, and fuel efficiency—critical factors for competitive racing and enthusiast vehicles.
The customization capabilities of flow forming are further supported by CNC control and advanced tooling, which enable precise adjustments to wall thickness, diameter, and surface finish. This flexibility makes it possible to produce limited runs or one-off parts economically, helping aftermarket suppliers and race teams respond quickly to evolving performance requirements or track conditions.
In addition to mechanical performance, flow-formed components often exhibit superior fatigue resistance and impact toughness compared to parts made by casting or conventional machining. This resilience is essential in motorsports, where parts must endure extreme stresses and repetitive loading cycles without failure. The process’s ability to refine the metal’s microstructure through controlled plastic deformation enhances these properties, extending component life and reducing the risk of catastrophic failures during high-speed events.
Flow forming also supports lightweighting initiatives that are crucial in competitive automotive environments. By reducing component weight without sacrificing strength or safety, flow forming helps vehicles achieve better power-to-weight ratios, improved braking performance, and more responsive handling. These advantages contribute directly to lap time improvements and overall race performance.
Beyond performance and aftermarket applications, flow forming is increasingly utilized in producing electric vehicle (EV) components such as motor housings, battery enclosures, and structural elements designed to manage thermal loads and electromagnetic interference. The process’s precision and material versatility allow manufacturers to optimize these parts for weight, strength, and heat dissipation, which are key to extending EV range and reliability.
Furthermore, flow forming aligns well with the automotive industry’s growing focus on smart manufacturing and Industry 4.0 integration. Automated monitoring and adaptive process controls improve product consistency, reduce scrap rates, and allow for real-time adjustments to accommodate material variations or changing production demands. This digital integration enhances quality assurance and supports traceability throughout the supply chain, which is increasingly important for meeting regulatory standards and customer expectations.
In summary, flow forming offers automotive manufacturers and aftermarket suppliers a powerful combination of precision, strength, efficiency, and customization. Its ability to produce high-performance, lightweight components tailored to specific applications ensures its continued relevance as vehicles evolve toward greater complexity, electrification, and performance. The process’s synergy with advanced materials and digital manufacturing further positions flow forming as a key enabler of innovation and competitiveness in the automotive sector.
Flow Forming for Aerospace Components
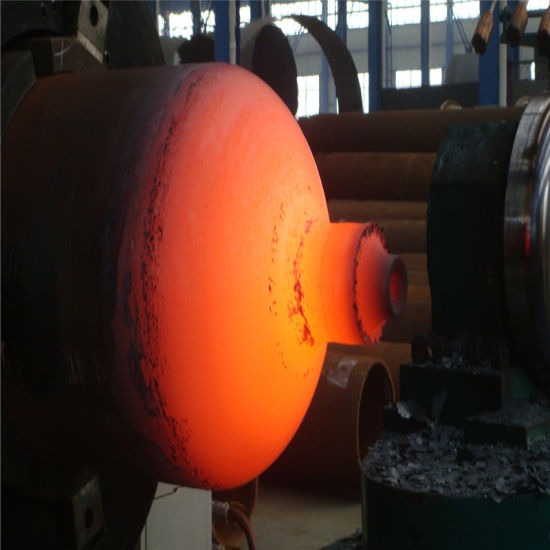
Flow forming is a critical manufacturing process in the aerospace industry, valued for its ability to produce lightweight, high-strength, and precision components essential for aircraft and spacecraft performance. Aerospace applications demand parts that can withstand extreme stresses, temperature fluctuations, and stringent safety standards while minimizing weight to improve fuel efficiency and payload capacity. Flow forming meets these requirements by enabling the creation of seamless, thin-walled cylindrical and conical components with superior mechanical properties.
Key aerospace components produced through flow forming include engine casings, compressor and turbine rings, landing gear parts, structural tubes, and fuel tanks. The process refines the metal’s grain structure via plastic deformation, resulting in enhanced strength, fatigue resistance, and toughness—properties crucial for the reliability and longevity of aerospace parts exposed to cyclic loads and harsh operating environments.
Flow forming’s capacity to maintain tight dimensional tolerances and produce complex geometries reduces the need for extensive machining or welding, which can introduce weaknesses or inconsistencies. This precision supports the fabrication of critical parts with minimal weight and maximum structural integrity, aligning with the aerospace industry’s emphasis on safety and performance.
Materials commonly used in aerospace flow forming include high-strength aluminum alloys, titanium, nickel-based superalloys, and stainless steels. These materials are chosen for their excellent strength-to-weight ratios, corrosion resistance, and thermal stability. Flow forming helps retain or enhance these material properties by minimizing heat input during forming, preserving microstructure and mechanical characteristics that might otherwise degrade through welding or casting.
The process is also instrumental in the production of large, complex components such as fuselage frames, rocket motor casings, and pressurized vessel shells. Its ability to form near-net-shape parts with uniform wall thickness enables substantial weight savings, which translates directly to improved aircraft range and payload capabilities.
Additionally, flow forming supports rapid prototyping and production scalability, allowing aerospace manufacturers to efficiently transition from design iterations to full-scale manufacturing. CNC-controlled flow forming machines facilitate the production of both high-volume standardized parts and specialized, custom components tailored to specific aerospace programs.
Integration with advanced inspection and quality assurance technologies ensures that flow-formed aerospace components meet rigorous industry standards. Non-destructive testing, inline dimensional monitoring, and process traceability contribute to certifying part integrity and compliance with strict aerospace regulations.
In summary, flow forming is an indispensable manufacturing method for aerospace components, offering unparalleled strength, precision, and weight reduction. Its compatibility with advanced materials and complex designs helps aerospace manufacturers meet demanding performance, safety, and efficiency goals essential for modern aircraft and space systems.
Flow forming’s role in aerospace extends beyond structural components to critical engine parts where performance and reliability are paramount. Components such as turbine rings, compressor discs, and exhaust liners benefit from flow forming’s ability to produce seamless parts with fine surface finishes and excellent mechanical properties. The process enhances fatigue resistance and reduces stress concentrations, which is essential in rotating parts subjected to high centrifugal forces and extreme temperatures. This contributes to longer service intervals, improved fuel efficiency, and overall engine performance.
The ability to work with difficult-to-machine materials like titanium and nickel-based superalloys makes flow forming especially valuable in aerospace applications. These materials offer superior strength and corrosion resistance at elevated temperatures but are challenging to form using traditional methods without compromising their properties. Flow forming achieves the desired shapes with controlled deformation, preserving the microstructure and mechanical integrity of these advanced alloys.
Weight reduction achieved through flow forming directly impacts aircraft efficiency and emissions. By producing thin-walled, seamless components that maintain structural integrity, manufacturers can reduce overall aircraft weight, leading to lower fuel consumption and extended range. This aligns with the aerospace industry’s increasing focus on sustainability and environmental responsibility.
Flow forming also facilitates the production of fuel system components and pressure vessels that must withstand high internal pressures and cyclic loading. Seamless construction minimizes leak risks and enhances safety, which is critical for systems handling volatile fuels or pressurized gases.
The aerospace industry’s demand for rapid innovation and customization is well supported by flow forming technology. CNC-controlled machines allow for quick adjustments in design and production parameters, enabling the manufacture of tailored parts for specific aircraft models or mission profiles. This flexibility is especially important in the space sector, where bespoke components often require precise specifications and small batch sizes.
Integration with digital manufacturing tools and real-time process monitoring enhances quality control, ensuring that every flow-formed part meets stringent aerospace standards. Data collected during forming can be used for predictive maintenance and lifecycle management, helping to optimize part performance and reduce downtime.
As aerospace technologies evolve to include more electric aircraft, unmanned aerial systems, and hypersonic vehicles, the demand for components that combine lightweight design with exceptional strength and thermal stability will continue to grow. Flow forming is well positioned to meet these needs through ongoing advancements in tooling, process automation, and materials science.
In conclusion, flow forming is a foundational technology in aerospace manufacturing, delivering components that meet the industry’s rigorous demands for strength, precision, and weight efficiency. Its adaptability, material compatibility, and integration with modern production techniques ensure it will remain essential in advancing aerospace innovation and performance well into the future.
Flow forming also contributes significantly to the aerospace supply chain by enabling cost-effective production without compromising quality. Compared to traditional forging or casting methods, flow forming reduces material waste and lowers energy consumption, helping manufacturers manage production costs while meeting stringent aerospace specifications. This efficiency is particularly valuable in the manufacture of large components or those made from expensive materials like titanium and superalloys.
The seamless nature of flow-formed parts eliminates welds and joints that can act as stress risers or potential failure points, thereby enhancing the safety and reliability of aerospace components. This is critical in applications such as rocket motor casings, pressure vessels, and structural frames, where failure is not an option.
Moreover, flow forming supports repair and refurbishment efforts in aerospace maintenance, repair, and overhaul (MRO) operations. Damaged or worn parts can often be reformed or refurbished using flow forming techniques, extending their service life and reducing the need for costly replacements. This capability supports sustainable practices and cost savings in fleet management.
Collaboration between aerospace engineers and flow forming specialists continues to push the boundaries of what can be achieved with this process. Advances in simulation software, real-time monitoring, and adaptive control systems enable tighter tolerances, improved repeatability, and the ability to form increasingly complex geometries. These innovations allow aerospace manufacturers to design lighter, stronger parts with integrated features that reduce assembly time and improve overall system performance.
The process also complements additive manufacturing by providing a means to refine and finish near-net-shape parts created by 3D printing, combining the design freedom of additive methods with the mechanical benefits of flow forming. This hybrid approach is particularly promising for low-volume, high-complexity aerospace components where performance and weight are critical.
Additionally, the integration of flow forming into digital twin frameworks and smart factory environments supports predictive maintenance and continuous quality improvement. By capturing detailed process data and linking it to product performance over time, manufacturers can optimize production parameters and anticipate maintenance needs, enhancing reliability and reducing downtime.
In summary, flow forming is integral not only to producing high-performance aerospace components but also to improving manufacturing efficiency, sustainability, and supply chain resilience. Its combination of precision, material efficiency, and adaptability positions it as a key enabler for the aerospace industry’s future challenges and innovations, from commercial aviation to space exploration.
Flow Forming Machines for Cylindrical Components
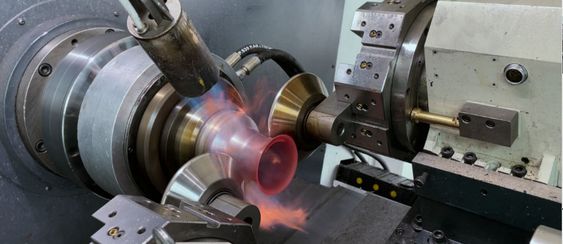
Flow forming machines designed for cylindrical components are specialized pieces of equipment engineered to precisely shape and reduce the thickness of tubular or cylindrical metal blanks into seamless, high-strength parts. These machines play a critical role in industries such as aerospace, automotive, defense, and energy, where cylindrical components with exact dimensions and superior mechanical properties are essential.
At their core, flow forming machines operate by rotating a metal workpiece—usually a thick-walled cylinder or tube—while one or more rollers apply controlled radial pressure. This pressure plastically deforms the metal, stretching and thinning it longitudinally, which results in a longer, thinner-walled, and stronger cylindrical component. The machines are designed to maintain tight tolerances on diameter, wall thickness, and roundness, ensuring consistent part quality.
Modern flow forming machines for cylindrical components typically feature CNC (computer numerical control) systems that allow precise control over parameters such as roller position, feed rate, spindle speed, and pressure. This enables the production of complex geometries, including tapered sections, varying wall thicknesses, and integrated features like flanges or shoulders, all within a single forming operation.
The machines are built to accommodate a wide range of materials, including aluminum, steel, titanium, and high-performance alloys. Their robust construction ensures stability and rigidity, minimizing vibration and deflection during forming, which is vital for achieving high-quality finishes and dimensional accuracy.
Flow forming machines vary in size and capacity depending on the application. Small to medium-sized machines are often used for components like automotive drive shafts, bicycle frames, or aerospace tubing, while large-scale machines handle sizable parts such as missile motor casings, rocket shells, or industrial pressure vessels.
Advanced flow forming machines may incorporate multiple rollers positioned around the workpiece to apply balanced forces, improving control over material flow and surface finish. Some setups include hydraulic or servo-driven actuators that dynamically adjust roller pressure during forming to optimize metal deformation and reduce defects.
In addition to forming, many flow forming machines integrate secondary processes such as in-line measurement systems, surface finishing, or automated loading and unloading to streamline production and improve throughput. These capabilities support both high-volume manufacturing and precision prototype development.
In summary, flow forming machines for cylindrical components are highly specialized tools that combine mechanical precision, advanced control systems, and flexible tooling to produce seamless, high-strength cylindrical parts. Their ability to efficiently form complex geometries from a variety of materials makes them indispensable in industries requiring lightweight, durable, and dimensionally accurate tubular components.
These machines are engineered to handle various sizes and thicknesses of cylindrical blanks, enabling the production of parts with wall thickness reductions sometimes exceeding 50%. This significant thinning is achieved while maintaining or even enhancing the mechanical properties of the material, thanks to the cold working effect imparted by the flow forming process. The refined grain structure resulting from the plastic deformation improves strength, fatigue resistance, and toughness, which are critical attributes for demanding applications.
Flow forming machines for cylindrical components often feature adaptable tooling systems that can be quickly reconfigured or customized to accommodate different part designs and production runs. This flexibility is essential for industries where component specifications frequently change or where multiple variants must be produced on the same equipment. The ability to switch between different mandrels, rollers, and supports without extensive downtime boosts overall manufacturing efficiency.
The precision control provided by CNC systems extends beyond positioning to include monitoring and adjusting parameters in real-time. Sensors and feedback loops detect variations in material behavior or thickness, enabling the machine to compensate dynamically and maintain consistent quality. This level of control reduces scrap rates, enhances repeatability, and ensures that critical tolerances are met with minimal manual intervention.
Because flow forming machines apply force gradually and uniformly, they minimize internal stresses and distortion that can occur with other forming methods. This characteristic is particularly valuable when working with high-strength alloys or materials with tight metallurgical requirements. Additionally, the seamless nature of flow-formed cylindrical components eliminates welds or joints, which are common failure points in pressurized or structural parts.
The integration of advanced diagnostics and automation features allows modern flow forming machines to operate with high degrees of autonomy, reducing operator dependency and enabling continuous production runs. Automated loading, unloading, and part transfer systems can be incorporated to further enhance throughput and reduce labor costs, making flow forming a competitive choice for both small-batch and large-scale manufacturing.
In applications requiring exceptionally smooth surface finishes, such as aerospace or medical tubing, flow forming machines can be equipped with rollers designed for minimal surface disruption. Post-forming processes like polishing or coating are often simplified due to the excellent initial surface quality, contributing to overall cost savings and faster production cycles.
Furthermore, these machines support the manufacture of components with variable wall thickness along their length, achieved by precise manipulation of roller angles and pressures. This capability allows engineers to optimize material distribution according to load requirements, resulting in parts that are both lighter and stronger where needed.
In summary, flow forming machines for cylindrical components combine precision, flexibility, and robust mechanical design to deliver high-quality, seamless tubular parts with excellent mechanical properties and tight tolerances. Their adaptability to different materials and geometries, coupled with advanced control and automation, makes them essential in sectors where performance, reliability, and efficiency are paramount.
Flow forming machines for cylindrical components also offer significant advantages in terms of sustainability and material efficiency. Because the process involves incremental plastic deformation rather than removal of material through machining, it generates minimal scrap, making it highly cost-effective and environmentally friendly. This reduction in waste is especially important when working with expensive or rare alloys like titanium or superalloys, where material savings can have a substantial economic impact.
The reduced need for secondary machining operations not only cuts down production time but also lowers energy consumption and tool wear. Flow-formed parts often require only minimal finishing or trimming, further streamlining the manufacturing workflow. This efficiency supports just-in-time production models and flexible manufacturing systems, enabling companies to respond rapidly to changes in demand or design specifications.
Maintenance and longevity of flow forming machines are enhanced by their robust construction and the use of high-quality components designed to withstand continuous operation under heavy loads. Predictive maintenance systems can be integrated to monitor machine health, reducing unexpected downtime and optimizing service schedules. This reliability is critical for industries such as aerospace and defense, where consistent part quality and supply chain dependability are vital.
In addition to cylindrical tubes and shells, flow forming machines are capable of producing components with complex features such as flanges, shoulders, and variable diameters without requiring additional welding or assembly. This capability enables designers to consolidate multiple parts into a single seamless component, improving structural integrity and reducing assembly time and cost.
Emerging trends in flow forming machine technology include the integration of artificial intelligence and machine learning algorithms to optimize process parameters automatically. These advancements enable the machines to adapt in real-time to material variations or environmental factors, enhancing part quality and reducing operator intervention. Combined with digital twin technology, manufacturers can simulate and predict forming outcomes before actual production, minimizing trial-and-error and accelerating development cycles.
Moreover, the ability to flow form advanced materials, including composites and metal-matrix hybrids, is being explored to meet the growing demand for lightweight, high-performance components in sectors like aerospace and electric vehicles. Flow forming machines equipped with specialized tooling and control systems can handle these novel materials, expanding the process’s applicability and future-proofing manufacturing capabilities.
Overall, flow forming machines for cylindrical components represent a sophisticated fusion of mechanical engineering, materials science, and digital technology. Their ongoing evolution ensures that they will continue to meet the increasingly complex demands of modern manufacturing, delivering parts that combine strength, precision, and efficiency across a wide range of industries.
Automated Flow Forming Systems
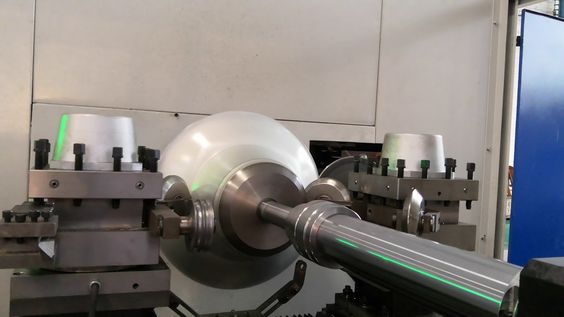
Automated flow forming systems represent the next evolution in flow forming technology, combining advanced mechanical design with cutting-edge automation and control to deliver higher precision, improved efficiency, and greater consistency in manufacturing seamless cylindrical and conical components. These systems integrate robotics, CNC controls, sensors, and data analytics to streamline the entire flow forming process—from material loading and forming to unloading and quality inspection—minimizing human intervention and maximizing throughput.
At the heart of automated flow forming systems is a CNC-controlled machine tool equipped with servo-driven rollers and mandrels that precisely control material deformation. Automation extends beyond just forming: robotic arms or automated conveyors handle raw material feeding and finished part extraction, enabling continuous, unattended operation. This seamless integration reduces cycle times, lowers labor costs, and minimizes the risk of operator error, which is especially valuable in high-volume or high-precision production environments.
Sensors and real-time monitoring technologies play a crucial role by continuously measuring parameters such as wall thickness, diameter, surface finish, roller force, and temperature. These data streams feed into advanced process control algorithms that adjust forming parameters on the fly, ensuring consistent product quality despite variations in material properties or environmental conditions. Automated feedback loops enable predictive maintenance by identifying potential equipment wear or faults before they cause downtime.
Automated systems also often include in-line non-destructive testing (NDT) capabilities such as ultrasonic thickness gauging, laser scanning, or eddy current inspection. This enables immediate detection of defects like cracks, voids, or dimensional deviations, ensuring only parts that meet stringent specifications proceed through the production line. The resulting traceability and quality assurance are critical for industries like aerospace, automotive, and defense, where certification standards are rigorous.
These systems are designed for flexibility, accommodating a wide range of materials—including aluminum, steel, titanium, and superalloys—and varying part geometries. Quick-change tooling and modular machine configurations allow manufacturers to switch between different production runs or adapt to evolving design requirements with minimal downtime. This adaptability supports both mass production and small-batch, high-mix manufacturing models.
Automation also facilitates integration with digital manufacturing platforms and Industry 4.0 frameworks. Data generated during flow forming can be connected to enterprise resource planning (ERP) systems, manufacturing execution systems (MES), and digital twins, enabling comprehensive process optimization, supply chain coordination, and lifecycle management. This connectivity improves responsiveness, reduces waste, and enhances overall operational efficiency.
Moreover, automated flow forming systems contribute to workplace safety by limiting operator exposure to moving machinery, heavy components, and repetitive tasks. This improves ergonomics and reduces the likelihood of accidents or injuries, supporting compliance with occupational health and safety regulations.
In summary, automated flow forming systems elevate the traditional flow forming process by combining precision engineering with sophisticated automation, real-time monitoring, and data integration. These advancements enable manufacturers to produce high-quality, seamless components more efficiently, reliably, and flexibly—meeting the demands of modern industries that require both scalability and precision.
Automated flow forming systems also enhance scalability, allowing manufacturers to ramp up production quickly to meet increasing demand without sacrificing quality or consistency. The integration of robotics and automated material handling means that these systems can operate continuously, 24/7, with minimal human oversight. This continuous operation not only boosts output but also optimizes energy use and reduces downtime associated with shift changes or manual interventions.
The precision and repeatability offered by automation reduce the variability inherent in manual operations, leading to tighter tolerances and higher yields. This is particularly important for critical applications such as aerospace engine components, medical device housings, and defense parts, where stringent quality standards must be consistently met. Automated data logging and traceability ensure that every component’s production history is recorded, supporting certification processes and enabling root cause analysis if defects arise.
Advanced automation also allows for more complex part geometries and customized production runs. Adaptive control systems can modify roller paths, pressures, and spindle speeds on the fly, accommodating subtle variations in design or material batches without requiring a full machine reprogramming. This flexibility reduces changeover times and facilitates just-in-time manufacturing strategies, which are increasingly important in competitive, fast-moving industries.
Incorporating machine learning algorithms into automated flow forming systems is an emerging trend that promises to further optimize the process. By analyzing historical and real-time production data, these algorithms can predict the best forming parameters, detect anomalies early, and even suggest maintenance actions before failures occur. This predictive capability not only improves part quality but also extends machine life and lowers operational costs.
Furthermore, automated flow forming systems can be integrated with other manufacturing processes, such as machining, heat treatment, or surface finishing, to create streamlined production cells. This integration shortens lead times and reduces handling risks, as parts move seamlessly between operations with minimal manual contact.
Environmental benefits also arise from automation. More precise control over material deformation and process parameters reduces energy consumption and material waste. Automated systems optimize resource use, contributing to greener manufacturing practices that are increasingly demanded by regulatory bodies and consumers alike.
Finally, the user interfaces of automated flow forming systems are designed for ease of use, featuring intuitive touchscreen controls, real-time process visualization, and remote monitoring capabilities. This user-centric design reduces training requirements and enables operators to quickly respond to alerts or adjust production settings, further enhancing operational efficiency.
Overall, automated flow forming systems represent a significant advancement in manufacturing technology, combining high precision, operational efficiency, and flexibility with improved safety and sustainability. Their adoption is transforming how industries produce critical cylindrical components, meeting the evolving challenges of modern production with innovation and reliability.
Compact Flow Forming Machines
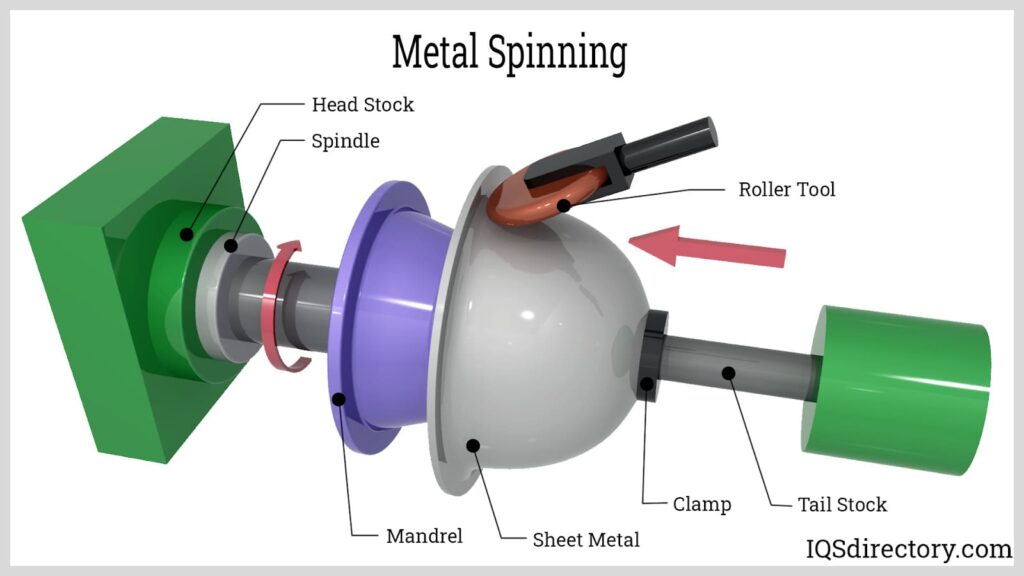
Compact flow forming machines are designed to deliver the benefits of traditional flow forming technology in a smaller, more space-efficient footprint. These machines are ideal for manufacturers who require precision metal forming capabilities but have limited floor space or need portable, flexible equipment for prototyping, small-batch production, or research and development applications.
Despite their reduced size, compact flow forming machines maintain high levels of accuracy and repeatability. They use the same fundamental principles as larger machines—rotating the workpiece on a mandrel while applying controlled pressure through rollers to plastically deform and thin the material—but are engineered with space-saving components and streamlined designs. This makes them well-suited for workshops, small manufacturing facilities, and laboratories.
Compact machines often incorporate CNC control systems to provide precise regulation of roller position, spindle speed, and feed rate, ensuring consistent quality in parts such as small-diameter tubes, sleeves, or cylindrical housings. Their versatility allows them to work with a variety of metals, including aluminum, steel, and some high-strength alloys, though capacity is naturally limited by their size.
These machines frequently feature modular tooling setups, enabling quick changeovers and adaptation to different part geometries or sizes without requiring significant reconfiguration. This flexibility supports low-volume, customized manufacturing and rapid iteration during product development phases.
Because of their compact design, these machines are also easier to install and integrate into existing production lines or research facilities. They can be moved or reconfigured with relative ease compared to large industrial flow forming systems, allowing manufacturers to optimize their workspace as production needs evolve.
Compact flow forming machines provide an efficient and cost-effective solution for producing precision seamless cylindrical components where space and budget constraints make larger equipment impractical. Their combination of accuracy, flexibility, and smaller footprint enables broader access to flow forming technology across industries ranging from aerospace and automotive to medical device manufacturing and specialty metal fabrication.
Compact flow forming machines also often come equipped with user-friendly interfaces and automated controls that simplify operation, reducing the need for highly specialized operators. This accessibility makes them suitable not only for specialized manufacturing plants but also for educational institutions and smaller workshops where flow forming techniques are being introduced or refined.
The smaller scale does not necessarily mean a compromise on material capabilities; many compact machines are engineered to handle a surprising range of alloys, including aluminum, stainless steel, and even certain titanium grades, allowing users to experiment with diverse materials and applications. While they may not match the throughput of large industrial machines, their precision and control allow for high-quality parts suitable for prototyping, small series production, or specialty components that require tight tolerances and excellent surface finishes.
Maintenance and setup for compact flow forming machines tend to be more straightforward, with accessible components and fewer subsystems compared to their larger counterparts. This ease of maintenance reduces downtime and operational costs, making them attractive for facilities with limited technical staff or where minimizing production interruptions is critical.
Additionally, many compact flow forming machines integrate with computer-aided design (CAD) and computer-aided manufacturing (CAM) software, enabling seamless transition from design to production. This integration supports rapid iteration and customization, allowing engineers to test and produce optimized cylindrical components with minimal lead time.
In research and development contexts, compact flow forming machines are valuable for exploring new materials, testing forming parameters, and developing novel component designs before scaling up to full production. Their flexibility and responsiveness enable quick adjustments to forming conditions, facilitating innovation and experimentation without the resource commitments required for larger equipment.
Overall, compact flow forming machines extend the reach of flow forming technology by providing a practical, versatile, and precise solution for manufacturers and researchers with limited space or lower volume requirements. They bridge the gap between large-scale industrial production and experimental or specialized manufacturing, making seamless cylindrical metal forming more accessible and adaptable across a wide range of applications.
Compact flow forming machines also support enhanced sustainability efforts by reducing energy consumption relative to larger, more power-intensive equipment. Their smaller motors and streamlined mechanics require less electricity, contributing to lower operational costs and a smaller environmental footprint. This aspect is increasingly important as manufacturers strive to align production practices with green manufacturing principles and regulatory demands.
Because of their size and agility, compact flow forming machines can be incorporated into flexible manufacturing cells, where they work alongside other automated or semi-automated equipment to create integrated, efficient production lines. This modularity enables manufacturers to scale operations up or down quickly, responding to market fluctuations or custom order requirements without extensive retooling or capital investment.
In terms of precision, compact machines often utilize high-quality spindle bearings, rigid frame designs, and advanced roller technology to maintain tight tolerances and smooth surface finishes, despite their smaller stature. This ensures that parts produced meet the stringent dimensional and quality requirements common in industries such as aerospace, medical devices, and electronics, where reliability and performance are critical.
The portability and ease of setup offered by many compact flow forming machines also make them suitable for on-site or field operations. For example, they can be deployed in remote manufacturing facilities, repair workshops, or research stations, providing critical metal forming capabilities without the need for large-scale infrastructure.
Advances in compact flow forming technology continue to push the boundaries of what smaller machines can achieve, including improved force application, faster cycle times, and the ability to handle more complex geometries. Integration with digital controls, sensors, and even cloud-based monitoring allows operators to maintain high standards of process control and traceability, even in decentralized or smaller-scale production environments.
Ultimately, compact flow forming machines provide an accessible, efficient, and adaptable solution for producing seamless cylindrical parts with precision and repeatability. Their design addresses the growing need for flexible manufacturing solutions that combine quality, speed, and sustainability, making flow forming technology more attainable for a wider range of applications and industries.
Horizontal Flow Forming Machine
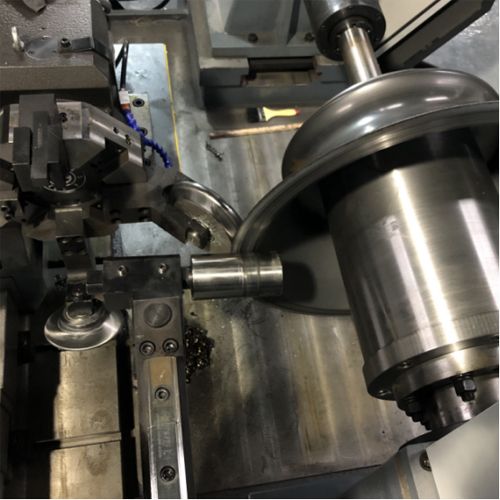
A horizontal flow forming machine is a type of metal forming equipment designed specifically to shape cylindrical or tubular components by rotating the workpiece about a horizontal axis. In this configuration, the workpiece—usually a thick-walled metal cylinder or tube—is mounted horizontally on a mandrel and spun at high speed while one or more rollers apply controlled pressure to plastically deform and reduce its wall thickness. This process elongates the part while maintaining or improving its mechanical properties, resulting in seamless, high-strength components.
The horizontal orientation offers several advantages. It facilitates easier loading and unloading of parts, especially longer or heavier components, by allowing gravity to assist in handling. It also provides better support for long workpieces, reducing deflection or bending during forming and ensuring dimensional accuracy. This setup is commonly used for producing items like aerospace engine casings, automotive drive shafts, pressure vessels, and large-diameter pipes.
Horizontal flow forming machines generally consist of a robust frame supporting a horizontally mounted spindle or mandrel, multiple rollers arranged along the length of the workpiece, and a CNC control system that manages spindle speed, roller pressure, and feed rates. The rollers can be positioned and adjusted to control material flow precisely, enabling the production of parts with variable wall thicknesses, tapered sections, or integrated features such as flanges and shoulders.
CNC automation plays a critical role in modern horizontal flow forming machines by enabling fine-tuned control over the forming parameters and allowing complex part geometries to be produced with high repeatability. Sensors and feedback systems monitor process variables such as force, displacement, and temperature to optimize forming conditions and prevent defects.
Horizontal flow forming machines are engineered to handle a wide range of materials, including aluminum, steel, titanium, and various high-performance alloys. Their sturdy construction minimizes vibration and deformation during forming, which is crucial for maintaining tight tolerances and excellent surface finishes.
These machines vary widely in size and capacity—from smaller units suitable for prototype development and small batch production to large industrial machines capable of forming heavy-duty components for oil and gas, aerospace, and defense sectors. Advanced models often incorporate multiple rollers that can operate independently or in coordination, providing balanced deformation forces and enhanced surface quality.
In summary, horizontal flow forming machines combine the efficiency and precision of flow forming with the practical benefits of horizontal workpiece orientation. This makes them well-suited for manufacturing long, seamless cylindrical parts with complex geometries and demanding quality requirements across various high-tech industries.
Horizontal flow forming machines also excel in their ability to produce components with varying wall thicknesses along the length of the part, a feature critical for optimizing material usage and enhancing performance. By precisely controlling the position, pressure, and feed rate of the rollers, manufacturers can create thicker sections where strength or stiffness is needed and thinner areas where weight reduction is desirable. This variable wall thickness capability is highly valuable in aerospace, automotive, and energy sectors, where weight-to-strength ratio directly impacts efficiency and cost.
The horizontal design simplifies integration with automated material handling systems, such as robotic loaders and unloaders, conveyor belts, or transfer stations, enabling continuous or near-continuous production cycles. This automation reduces manual labor, improves safety by minimizing operator interaction with moving parts, and increases overall throughput. Combined with CNC controls and real-time process monitoring, horizontal flow forming machines can achieve very tight dimensional tolerances and repeatable results even in demanding production environments.
Another advantage of the horizontal orientation is the ease of accommodating longer or more complex parts. Unlike vertical machines, where gravity can affect the uniformity of material flow or cause deformation, horizontal machines support the workpiece evenly along its length. This support reduces the risk of bending or sagging, ensuring more consistent thickness and surface finish. Additionally, horizontal setups allow for straightforward addition of auxiliary tooling or secondary operations, such as in-line machining, heat treatment, or non-destructive testing, further streamlining the manufacturing workflow.
The rugged construction of horizontal flow forming machines enables them to handle high forming forces necessary for working with tough materials like titanium alloys and superalloys. Their stability and rigidity minimize vibration and deflection, which not only protect the machine’s components from premature wear but also enhance the quality of the formed parts. As a result, these machines are preferred for critical applications where structural integrity and precision are paramount.
Furthermore, modern horizontal flow forming machines often feature modular tooling systems, allowing quick changeover between different part designs and sizes. This modularity supports both large-scale production runs and smaller, customized batches without significant downtime, offering manufacturers flexibility in responding to changing market demands or design iterations.
Integration with Industry 4.0 technologies is increasingly common, with horizontal flow forming machines equipped with sensors that collect data on process parameters, machine condition, and part quality. This data can be analyzed to optimize forming cycles, predict maintenance needs, and ensure traceability—key factors for quality assurance and regulatory compliance in sectors such as aerospace and medical device manufacturing.
Overall, horizontal flow forming machines provide a versatile and reliable platform for producing seamless cylindrical components that require precise dimensional control, high structural performance, and excellent surface quality. Their combination of mechanical robustness, automation readiness, and advanced process control makes them indispensable tools for modern metal forming industries aiming for efficiency and high-quality output.
Horizontal flow forming machines also enable manufacturers to reduce lead times significantly by combining multiple forming steps into a single, continuous process. Traditional manufacturing of cylindrical components might require separate operations like forging, machining, and welding, each adding time and potential sources of defects. In contrast, flow forming on a horizontal machine can achieve near-net-shape parts with improved metallurgical properties, often eliminating or minimizing downstream machining and assembly processes.
The process inherently improves material properties through work hardening and grain refinement, resulting in parts with enhanced strength, fatigue resistance, and dimensional stability. This metallurgical advantage is particularly important in high-stress applications such as aerospace engine casings, automotive drive shafts, or pressure vessels, where component failure is not an option.
Horizontal flow forming machines are also adaptable to a wide variety of part sizes and shapes. With adjustable mandrels and interchangeable tooling, they can accommodate components ranging from small tubes and sleeves to large-diameter, thick-walled cylinders. This adaptability makes them a versatile choice for manufacturers dealing with diverse product lines or those aiming to consolidate multiple production processes into a single machine setup.
Safety features are integral to modern horizontal flow forming machines, including enclosed work zones, emergency stop systems, and safety interlocks that protect operators during high-speed rotation and heavy force application. Combined with reduced manual handling due to automation, these safety measures contribute to a safer working environment and compliance with stringent occupational health regulations.
Moreover, horizontal flow forming machines can be customized with features such as temperature-controlled mandrels to handle warm or hot flow forming processes, expanding their capability to work with materials that require elevated temperatures for optimal deformation. This versatility allows manufacturers to tailor the forming process to the specific properties of each material, balancing formability and mechanical performance.
From a maintenance perspective, these machines are designed for durability and ease of service. Key components like bearings, spindles, and hydraulic systems are built to withstand high loads and frequent use, and modular design principles simplify replacement or upgrade of parts. Predictive maintenance technologies integrated into the machine’s control system can alert operators to potential issues before they lead to downtime, enhancing overall equipment effectiveness.
In summary, horizontal flow forming machines offer a comprehensive solution for producing high-quality, seamless cylindrical components with excellent mechanical properties and dimensional accuracy. Their flexibility, robustness, and integration with modern automation and monitoring technologies make them essential in industries where performance, efficiency, and reliability are critical.
Spare Parts for Flow Forming Machines
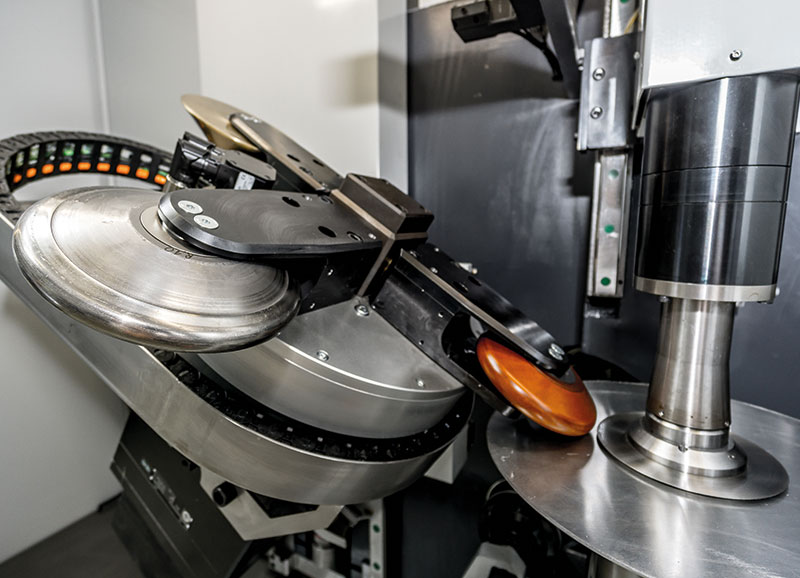
Spare parts for flow forming machines are critical components that ensure the continuous, reliable operation and longevity of these specialized metal forming systems. Given the demanding nature of flow forming—which involves high forces, precise movements, and constant contact between rollers and workpieces—maintaining high-quality spare parts inventory is essential to minimize downtime and preserve production efficiency.
Key spare parts typically include rollers, mandrels, bearings, seals, hydraulic components, spindle assemblies, drive motors, sensors, and control system elements. Rollers are among the most frequently replaced parts, as they endure significant wear and stress during the plastic deformation of metal. They must be made from high-strength, wear-resistant materials—often tool steels or carbide-coated alloys—to withstand abrasive contact and maintain dimensional accuracy over time.
Mandrels, which provide the internal support and shape during forming, also require careful maintenance and occasional replacement. They must retain tight tolerances to ensure consistent wall thickness and part geometry. Spare mandrels are often customized to specific part designs, making early procurement and proper storage important to avoid production delays.
Bearings and seals are vital for the smooth rotation and positioning of both the mandrel and rollers. High-quality bearings reduce friction and prevent overheating, while robust seals protect internal components from contamination by dust, metal particles, or lubricants. Hydraulic components such as pumps, valves, and cylinders control the pressure and positioning of forming rollers and must be regularly inspected and replaced as needed to maintain precise control of forming forces.
Spindle assemblies, including shafts and couplings, transfer rotational motion and torque from the drive motor to the workpiece and must be durable enough to handle high loads without deformation or vibration. Motors, gearboxes, and drive belts or chains also form part of the spare parts ecosystem, ensuring consistent machine power and speed control.
Sensors and control system components—including encoders, load cells, pressure sensors, and PLC modules—are essential for automation and real-time process monitoring. Their proper functioning guarantees that forming parameters stay within specified ranges, safeguarding part quality and preventing equipment damage.
Maintaining an organized inventory of genuine or OEM spare parts, preferably sourced from the machine manufacturer or trusted suppliers, is critical. Using authentic components ensures compatibility, maintains machine warranties, and upholds safety standards. Many manufacturers offer service agreements or spare parts kits tailored to specific machine models to streamline maintenance and repairs.
Quick availability of spare parts minimizes machine downtime, which can be costly in high-volume or just-in-time production environments. Proper documentation, including part numbers, specifications, and replacement schedules, aids maintenance teams in planning and executing repairs efficiently.
In addition, some spare parts can be refurbished or reconditioned—such as rollers that are ground or coated anew—to extend their service life, reducing costs and environmental impact.
In summary, spare parts for flow forming machines are a vital aspect of machine maintenance and operational reliability. A well-managed spare parts program, combined with proactive monitoring and timely replacement, ensures that flow forming equipment continues to produce high-quality seamless components with minimal interruption.
Spare parts management for flow forming machines also involves forecasting wear patterns and lifecycle expectations based on operational data and material characteristics. By analyzing factors such as production volume, forming pressures, and the types of materials processed, maintenance teams can predict when key components are likely to require replacement. This predictive approach helps avoid unexpected breakdowns and aligns spare parts procurement with actual machine needs, optimizing inventory costs and reducing waste.
Customization plays a significant role in spare parts for flow forming machines, as many components are tailored to specific machine models or part designs. Rollers, mandrels, and tooling often have unique dimensions and surface finishes suited to particular applications. Therefore, having access to manufacturer expertise and original design specifications is essential to ensure that replacements maintain the precision and performance required by the flow forming process.
The supply chain for these spare parts can sometimes be complex, especially for highly specialized or custom components. Manufacturers may keep critical spares in stock or offer expedited fabrication services, but lead times can vary depending on part complexity and material availability. Developing strong relationships with trusted suppliers and establishing clear communication channels with the machine manufacturer helps mitigate risks associated with supply delays.
Technological advancements have also influenced spare parts quality and availability. Improved materials, such as advanced coatings for rollers or enhanced alloys for mandrels, extend part lifespan and reduce the frequency of replacements. Additionally, additive manufacturing techniques are emerging as a way to produce complex spare parts more quickly and cost-effectively, especially for rare or obsolete components.
Proper storage and handling of spare parts are crucial to preserving their condition until use. Sensitive components like bearings and seals require clean, controlled environments to prevent contamination or damage. Inventory management systems with barcoding or RFID tracking improve traceability and ensure that parts are rotated and used before expiry or degradation occurs.
Training for maintenance personnel on correct installation and handling of spare parts is another key factor. Even the highest quality component can fail prematurely if not installed properly or if the machine is not operated within its design parameters. Comprehensive manuals, support from manufacturers, and ongoing technical training contribute to effective spare parts usage and overall machine reliability.
Environmental considerations also come into play, as efficient spare parts management reduces waste and supports sustainability goals. Refurbishing and reconditioning parts when feasible, recycling worn components, and selecting materials with lower environmental impact align with modern manufacturing’s increasing focus on green practices.
Ultimately, the effectiveness of a flow forming machine’s spare parts program directly influences production continuity, product quality, and total cost of ownership. By combining strategic planning, supplier collaboration, technological innovation, and skilled maintenance, manufacturers can maximize machine uptime and maintain the high standards demanded by precision flow forming operations.
In addition to the technical and logistical aspects, effective spare parts management for flow forming machines also plays a crucial role in minimizing operational risks. Unexpected machine downtime due to unavailable or incorrect parts can lead to missed delivery deadlines, increased labor costs, and potential damage to customer relationships. Having a well-structured spare parts inventory ensures that critical components are on hand when needed, enabling rapid repairs and reducing the impact of unplanned stoppages.
Manufacturers often implement tiered spare parts strategies, categorizing components based on their criticality and replacement frequency. High-wear items like rollers and seals might be stocked in larger quantities and replaced routinely, while less common or longer-lasting parts are kept in smaller reserves or ordered as needed. This approach balances inventory costs with readiness, ensuring essential parts are always available without excessive capital tied up in stock.
Furthermore, documentation and traceability of spare parts usage are integral to quality control and regulatory compliance. Recording part numbers, installation dates, and maintenance histories helps track machine performance over time and supports audits in industries with strict standards, such as aerospace or medical device manufacturing. This data also facilitates root cause analysis if failures occur, enabling continuous improvement in maintenance practices and part selection.
Collaboration with original equipment manufacturers (OEMs) often includes access to technical support, training, and software updates that can optimize spare parts utilization. OEMs may provide predictive maintenance tools, remote diagnostics, or on-site assistance to help identify when parts need replacement before failure occurs. Leveraging these resources enhances the machine’s operational reliability and extends its service life.
In addition, some manufacturers offer aftermarket spare parts options that provide cost-effective alternatives to OEM components without compromising quality. These parts can be particularly valuable for older machines where OEM parts are scarce or discontinued. However, careful evaluation is necessary to ensure compatibility and maintain warranty coverage.
Incorporating digital inventory management systems with real-time monitoring and automated reorder triggers streamlines spare parts procurement. These systems reduce human error, prevent stockouts, and improve response times. Integration with the machine’s control software can also enable condition-based maintenance, where parts are replaced based on actual wear and usage data rather than fixed schedules.
Ultimately, a proactive, well-organized spare parts management approach is essential for maximizing the productivity and longevity of flow forming machines. It supports seamless manufacturing workflows, maintains product quality, and safeguards the significant investment represented by these precision metal forming systems. By combining technical expertise, strategic planning, and modern technology, manufacturers can ensure that their flow forming operations remain efficient, reliable, and competitive.
Flow Forming Machine Maintenance Plans
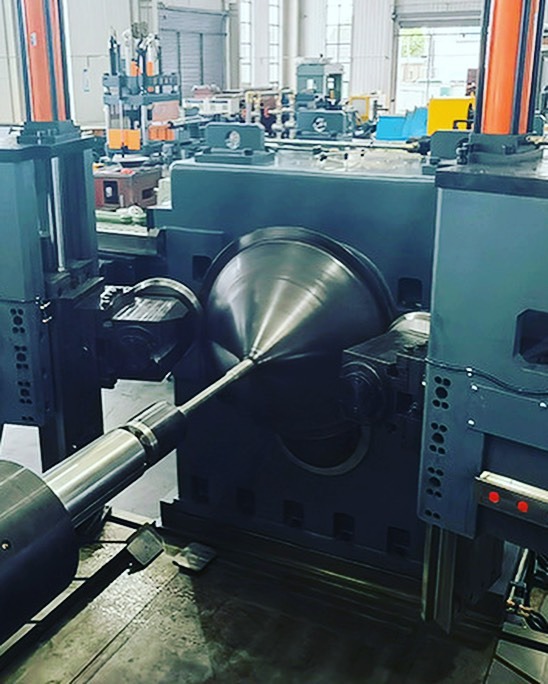
Flow forming machine maintenance plans are structured programs designed to ensure the optimal performance, reliability, and longevity of flow forming equipment through systematic inspection, servicing, and repair activities. Given the precision and mechanical intensity of flow forming processes, well-developed maintenance plans are critical for minimizing downtime, preventing unexpected failures, and maintaining consistent product quality.
A comprehensive maintenance plan typically combines preventive, predictive, and corrective maintenance strategies. Preventive maintenance involves scheduled inspections and routine servicing based on time intervals or machine usage metrics. This includes tasks such as lubrication of bearings and moving parts, checking and tightening bolts and fittings, inspecting rollers and mandrels for wear or damage, verifying hydraulic system pressures and fluid levels, and cleaning critical components to prevent contamination.
Predictive maintenance leverages real-time monitoring and data analysis to assess machine condition and predict when maintenance should be performed. Sensors integrated into the machine may track vibration, temperature, pressure, and load to identify early signs of component degradation. This approach allows maintenance teams to intervene just before a failure occurs, optimizing part replacement schedules and reducing unnecessary downtime.
Corrective maintenance addresses issues identified during inspections or after a breakdown. It includes repair or replacement of worn or damaged parts, realignment of mechanical assemblies, recalibration of control systems, and troubleshooting of electrical or hydraulic faults. Prompt corrective action minimizes the impact on production and prevents secondary damage to the machine.
Effective maintenance plans also incorporate detailed documentation, including maintenance schedules, checklists, part replacement records, and service logs. These records support traceability, quality assurance, and compliance with industry standards or regulations. They also facilitate communication between operators, maintenance personnel, and management.
Training and clear assignment of responsibilities are vital components of successful maintenance programs. Operators must be trained to recognize early signs of problems and perform basic daily checks, while specialized technicians handle more complex servicing and repairs. Regular training updates ensure personnel stay current with machine technology and maintenance best practices.
Advanced flow forming machines often include built-in diagnostic tools and software that assist in maintenance planning by alerting operators to abnormal conditions and suggesting corrective actions. Integration with centralized maintenance management systems can further streamline scheduling, inventory control for spare parts, and reporting.
Environmental factors, such as operating temperature, humidity, and cleanliness of the workspace, should be considered in maintenance planning, as they can significantly affect machine wear and performance. Implementing protective measures like dust covers, controlled environments, or filtration systems helps extend machine life.
Overall, a well-designed flow forming machine maintenance plan maximizes equipment availability, maintains production quality, and protects the substantial capital investment in these complex systems. It combines routine care with data-driven insights and responsive repairs, aligning maintenance activities with operational goals and industry requirements.
Flow forming machine maintenance plans also emphasize the importance of regular calibration and alignment checks to maintain dimensional accuracy and part quality. Over time, mechanical components such as rollers, mandrels, and spindles can shift or wear unevenly, leading to deviations in forming geometry. Scheduled calibration ensures that all machine axes, tooling, and control systems remain within specified tolerances, preventing defects and reducing scrap rates.
Lubrication management is another critical aspect, as proper lubrication reduces friction, prevents overheating, and limits wear on moving parts. Maintenance plans typically include detailed lubrication schedules specifying types of lubricants, application points, and intervals. Automated lubrication systems can be integrated into the machine to maintain consistent lubrication, reducing human error and improving machine uptime.
Hydraulic and pneumatic systems, which control roller pressure and positioning in many flow forming machines, require careful monitoring. Maintenance routines involve checking for leaks, testing pressure levels, replacing worn seals and hoses, and ensuring fluid cleanliness. Contaminated or degraded hydraulic fluids can impair system responsiveness and cause component damage, making fluid analysis and timely replacement essential.
Electrical and control systems also form a vital part of maintenance. Inspecting wiring, connectors, sensors, and PLCs helps prevent failures caused by loose connections, corrosion, or electrical surges. Software updates and backup procedures should be incorporated to maintain optimal machine control and protect against data loss.
Safety systems, including emergency stops, guards, and interlocks, must be regularly tested and maintained to protect operators and comply with regulations. Maintenance plans often specify inspection protocols and testing frequencies to ensure these features function reliably.
Incorporating operator involvement in daily or weekly maintenance checks helps catch minor issues before they escalate. Simple tasks like cleaning debris, checking for unusual noises or vibrations, and monitoring process parameters empower operators to contribute actively to machine health.
Finally, continuous improvement of maintenance plans is essential. Feedback from maintenance activities, failure analysis, and machine performance data should be reviewed periodically to update procedures, adjust schedules, and adopt new technologies or best practices. This dynamic approach ensures maintenance remains aligned with evolving operational demands and technological advances, ultimately sustaining the efficiency and precision of flow forming machines over their service life.
Additionally, maintenance plans for flow forming machines often include periodic overhaul and refurbishment schedules. Overhauls involve comprehensive disassembly, inspection, and replacement or repair of major components such as bearings, spindles, rollers, and hydraulic systems. This deep maintenance helps restore machine accuracy and performance after extended use or heavy production cycles, preventing catastrophic failures and extending the overall service life.
Refurbishment programs may also address surface treatments and coatings on rollers and mandrels, which are subject to wear and corrosion. Restoring these surfaces ensures continued precision in metal forming and protects against material defects in the finished parts. Such refurbishments are typically planned based on wear measurements or part quality feedback.
Another important element is the documentation and analysis of failure modes and maintenance costs. Keeping detailed records allows manufacturers to identify recurring issues or weak points in the machine design or operating practices. This data supports decision-making regarding upgrades, part redesigns, or process adjustments aimed at reducing maintenance frequency and costs.
Integrating predictive analytics and condition monitoring technologies can further enhance maintenance plans. Tools such as vibration analysis, thermal imaging, and oil analysis detect early warning signs of component degradation, enabling maintenance teams to schedule interventions at optimal times. This proactive maintenance reduces unplanned downtime and helps prioritize resource allocation.
Coordination between maintenance and production scheduling is also crucial. Maintenance activities should be planned to minimize impact on manufacturing timelines, ideally aligning with planned production breaks or lower-demand periods. Effective communication between departments ensures that maintenance does not inadvertently disrupt workflows or delay deliveries.
Sustainability considerations are increasingly influencing maintenance practices. Selecting environmentally friendly lubricants, recycling worn parts, and optimizing energy use during maintenance contribute to greener manufacturing operations. These efforts align with corporate social responsibility goals and may also reduce operating costs.
Finally, collaborating closely with machine manufacturers or authorized service providers offers access to specialized expertise, original spare parts, and technical support. This partnership helps maintain warranty compliance and ensures that maintenance procedures conform to OEM recommendations, preserving machine performance and safety standards.
In summary, flow forming machine maintenance plans encompass a holistic approach—combining routine care, advanced diagnostics, strategic overhauls, and continuous improvement—to keep these precision machines operating efficiently, producing high-quality parts while maximizing uptime and minimizing total ownership costs.
EMS Metalworking Machines
We design, manufacture and assembly metalworking machinery such as:
- Hydraulic transfer press
- Glass mosaic press
- Hydraulic deep drawing press
- Casting press
- Hydraulic cold forming press
- Hydroforming press
- Composite press
- Silicone rubber moulding press
- Brake pad press
- Melamine press
- SMC & BMC Press
- Labrotaroy press
- Edge cutting trimming machine
- Edge curling machine
- Trimming beading machine
- Trimming joggling machine
- Cookware production line
- Pipe bending machine
- Profile bending machine
- Bandsaw for metal
- Cylindrical welding machine
- Horizontal pres and cookware
- Kitchenware, hotelware
- Bakeware and cuttlery production machinery
as a complete line as well as an individual machine such as:
- Edge cutting trimming beading machines
- Polishing and grinding machines for pot and pans
- Hydraulic drawing presses
- Circle blanking machines
- Riveting machine
- Hole punching machines
- Press feeding machine
You can check our machinery at work at: EMS Metalworking Machinery – YouTube
Applications:
Flange-punching
Beading and ribbing
Flanging
Trimming
Curling
Lock-seaming
Ribbing