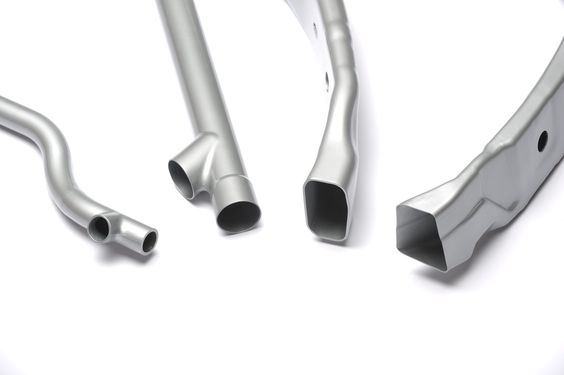
Hydroforming Tools Manufacturers: Sheet hydroforming presses are specialized machines used in the metal forming industry to shape sheet metal into complex geometries by applying hydraulic pressure. Unlike traditional stamping or mechanical presses, which rely on matched male and female dies to form parts, sheet hydroforming presses use a single-sided tool (usually a punch) and a flexible diaphragm or bladder that applies uniform hydraulic pressure to mold the metal sheet into the desired shape. This process allows manufacturers to produce parts with greater precision, fewer defects, and enhanced material efficiency.
The technology has found widespread use in industries such as automotive, aerospace, appliance manufacturing, and medical device production. Its ability to form complex, lightweight, and high-precision components with fewer tooling requirements makes it a popular choice for high-performance applications.
This guide will explore the working principles, types, advantages, and applications of sheet hydroforming presses.
Working Principles of Sheet Hydroforming Presses
Sheet hydroforming presses use hydraulic fluid to apply pressure evenly over a metal sheet, pressing it against a die to create the desired shape. The process involves three main components: a rigid die, a hydraulic bladder or diaphragm, and hydraulic fluid. The die defines the final geometry of the part, while the hydraulic bladder (or diaphragm) transmits the fluid pressure to mold the sheet metal.
1.1 Basic Process Steps
The sheet hydroforming process typically involves the following steps:
- Die Placement: A rigid die, representing the shape of the final part, is placed in the press. This die is a solid, fixed structure.
- Sheet Metal Placement: A flat metal sheet is positioned over the die. Common materials include aluminum, stainless steel, titanium, and mild steel.
- Hydraulic Bladder Engages: A flexible bladder, filled with hydraulic fluid, is placed over the metal sheet. This bladder replaces the traditional upper die used in conventional stamping.
- Pressurization: The hydraulic system applies controlled fluid pressure through the bladder, forcing the sheet metal to conform to the shape of the die below.
- Part Removal: After the pressure is released, the bladder retracts, and the formed metal part is removed from the die.
The uniform application of pressure over the entire sheet ensures smooth material flow, precise geometry, and reduced stress on the metal, resulting in parts with fewer defects like wrinkling or tearing.
1.2 Types of Sheet Hydroforming Presses
There are two main types of sheet hydroforming presses, based on how the hydraulic pressure is applied:
- Fluid Cell Hydroforming Presses: In this method, a flexible diaphragm filled with hydraulic fluid applies uniform pressure over the sheet metal. This press is well-suited for relatively shallow, simple shapes and works well for smaller production runs or prototypes.
- Deep Draw Sheet Hydroforming Presses: Designed for deep-draw applications, these presses use higher hydraulic pressures to form more complex and deeper parts. They can handle deep, intricate shapes and are commonly used in automotive and aerospace applications.
2. Advantages of Sheet Hydroforming Presses
Sheet hydroforming presses offer several advantages over traditional metal forming processes such as stamping and mechanical pressing. These benefits make sheet hydroforming presses an attractive option for high-performance and precision-driven industries.
2.1 Design Flexibility and Complex Geometries
One of the most significant advantages of sheet hydroforming presses is the ability to form complex geometries in a single operation. The flexible bladder evenly distributes pressure across the sheet, allowing for precise and uniform shaping even with intricate designs.
- Shallow and Deep Draws: Sheet hydroforming presses can be used to create both shallow and deep draws, expanding the range of possible geometries. This flexibility is particularly useful in industries like aerospace and automotive, where complex shapes are common.
- Variable Wall Thickness: The process allows for greater control over material thinning and deformation, making it possible to form parts with variable wall thicknesses. This improves the structural integrity and performance of the part.
2.2 Fewer Tooling Requirements
Sheet hydroforming only requires a single rigid die, eliminating the need for a second matching die as in traditional stamping. This reduces tooling costs, especially for low-volume production runs or parts with complex designs that would require intricate tooling.
- Cost Savings in Tooling: With fewer dies needed and no need for expensive matched sets, manufacturers can significantly reduce the upfront tooling costs. This makes sheet hydroforming an ideal choice for prototyping or custom parts where high-cost tooling isn’t justified.
- Reduced Tool Wear: Because the process applies less localized stress on the material and tooling, the life of the die is extended, and maintenance costs are lower.
2.3 Improved Part Quality and Surface Finish
The uniform application of pressure in hydroforming presses results in smoother surfaces and higher-quality finishes than traditional stamping. Since there is no direct contact between the punch and the sheet metal during most of the forming process, defects such as scratches, tool marks, and irregularities are minimized.
- Minimal Surface Defects: The flexible bladder’s uniform pressure prevents localized material stress, which reduces the likelihood of wrinkling, tearing, or surface imperfections. This is particularly important in industries such as aerospace or medical devices, where surface finish quality is critical.
- Consistent Dimensional Accuracy: Hydroforming allows for tight tolerances and high repeatability, ensuring that every part meets the required dimensional specifications. This precision is difficult to achieve with traditional methods, especially for complex shapes.
2.4 Material Efficiency
Because the material is evenly stretched and formed, material thinning is minimized, leading to better material utilization. This efficiency not only reduces material costs but also ensures that the final part is stronger and more durable.
- Reduced Material Waste: Traditional methods often result in high material waste due to excess trimming and discarded parts. In contrast, hydroforming presses form the part directly from the sheet, reducing the amount of scrap material generated.
3. Applications of Sheet Hydroforming Presses
The versatility and efficiency of sheet hydroforming presses make them well-suited for a variety of industries. Below are some of the most common applications:
3.1 Automotive Industry
In the automotive sector, sheet hydroforming presses are used to create lightweight, high-strength components that contribute to better fuel efficiency and safety. Common parts include:
- Body Panels: Hydroformed sheet metal is used for complex body panels, doors, and hoods. The ability to form complex shapes in a single operation reduces the number of welding seams, improving the structural integrity and appearance of the vehicle.
- Structural Components: Sheet hydroforming is used to produce reinforcements, frame parts, and crash structures that need to withstand impact forces while maintaining a lightweight design.
3.2 Aerospace Industry
Aerospace manufacturers rely on sheet hydroforming to produce high-performance components that require both precision and lightweight properties. The process is particularly valuable for creating complex, thin-walled parts that would be difficult or impossible to achieve using conventional methods.
- Fuselage Components: Sheet hydroforming is used to create lightweight structural components for aircraft fuselages, which are critical for reducing the aircraft’s overall weight and improving fuel efficiency.
- Engine Components: Complex parts such as aircraft engine covers and heat shields are often hydroformed due to the process’s ability to produce seamless, high-strength components that can withstand extreme temperatures and stresses.
3.3 Medical Devices
In the medical field, precision, surface finish, and biocompatibility are essential. Sheet hydroforming presses are used to manufacture surgical instruments, implant components, and diagnostic equipment from materials like titanium and stainless steel.
- Surgical Instruments: The smooth, high-quality finishes produced by hydroforming are ideal for medical instruments, ensuring ease of cleaning and sterilization.
- Implant Components: Hydroforming is also used to create biocompatible implants that must conform to specific anatomical shapes and meet stringent regulatory standards.
3.4 Consumer Goods and Appliances
In consumer goods, sheet hydroforming presses are used to manufacture durable and aesthetically pleasing components for products like kitchen appliances, sporting goods, and furniture. The process allows for sleek, modern designs without compromising material strength.
- Appliance Panels: Sheet hydroforming is used to create appliance housings, control panels, and decorative elements for products like refrigerators, washing machines, and ovens.
4. Challenges and Limitations of Sheet Hydroforming
While sheet hydroforming presses offer many advantages, there are certain challenges and limitations to consider.
- Initial Investment: The hydraulic systems and presses required for sheet hydroforming can be more expensive than traditional stamping presses, especially for high-pressure applications.
- Cycle Time: Hydroforming processes, especially deep-draw applications, may have longer cycle times compared to traditional stamping, which could affect overall production speed in high-volume settings.
- Material Limitations: Not all materials are suitable for hydroforming. Low-ductility materials may experience cracking or other defects, limiting their use in this process.
Conclusion
Sheet hydroforming presses represent a powerful tool in modern manufacturing, enabling the production of complex, high-quality components with fewer tooling requirements, improved material efficiency, and greater design flexibility. The technology’s ability to create seamless, precise parts with excellent surface finishes makes it indispensable in industries such as automotive, aerospace, medical devices, and consumer goods.
While there are some limitations, such as higher initial costs and potential cycle time challenges, the benefits of sheet hydroforming make it an increasingly popular choice for manufacturers looking to produce lightweight, high-performance components with precision and cost efficiency.
Working Principles of Sheet Hydroforming Presses
Sheet hydroforming is a metal forming process that uses hydraulic pressure to shape sheet metal into complex geometries. Sheet hydroforming presses are specialized machines designed for this process, offering a flexible and precise method for forming parts with smooth surfaces, complex shapes, and minimal defects. Unlike traditional stamping, where matched male and female dies are used, sheet hydroforming employs a single-sided die and a flexible diaphragm or bladder, which presses the sheet metal into the desired shape under the influence of hydraulic pressure.
This guide will explore the working principles of sheet hydroforming presses, outlining the key steps in the process, the components involved, and the factors that contribute to the accuracy and quality of the final part.
1. Basic Operating Principle
At its core, sheet hydroforming relies on applying uniform hydraulic pressure to force a metal sheet against a die. The pressure is transmitted through a flexible diaphragm or bladder, which ensures that the sheet conforms precisely to the shape of the die. Unlike mechanical presses, which use rigid tooling to deform the metal, sheet hydroforming achieves the desired part geometry through the controlled application of fluid pressure, providing greater precision and fewer defects.
2. Key Components of a Sheet Hydroforming Press
The main components of a sheet hydroforming press include:
- Hydraulic System: The heart of the hydroforming press, responsible for generating the fluid pressure needed to shape the metal. The hydraulic system includes pumps, valves, accumulators, and control systems.
- Rigid Die: A fixed die that defines the shape of the final part. The die can be a solid, one-sided tool that the sheet metal is pressed against during the forming process.
- Flexible Diaphragm/Bladder: A flexible membrane that holds hydraulic fluid and transfers the pressure uniformly to the sheet metal. It replaces the upper die in traditional pressing methods.
- Sheet Metal Blank: A flat piece of metal placed between the die and the diaphragm. Common materials include aluminum, stainless steel, titanium, and other alloys depending on the application.
3. Step-by-Step Working Process
3.1 Die and Sheet Metal Placement
The process begins by placing a rigid die in the lower section of the hydroforming press. This die corresponds to the shape of the final part. A flat sheet of metal, also known as a blank, is positioned on top of the die.
- Material Selection: The metal sheet is typically made from materials with good formability, such as aluminum, steel, stainless steel, or titanium, depending on the application.
The single-sided die means that the sheet metal is pressed into shape by the hydraulic pressure, not by a second die. This is a key difference from traditional stamping methods, where both a male and female die are required.
3.2 Sealing and Clamping
Once the metal sheet is placed on the die, the hydroforming machine’s flexible diaphragm is lowered into position, and the sheet is sealed and clamped between the die and the diaphragm. This clamping ensures that the sheet remains in the correct position throughout the forming process and prevents any movement or misalignment.
- Clamping Force: Sufficient clamping force is applied to prevent the sheet from shifting during the process, which is crucial for achieving high precision and preventing defects.
3.3 Hydraulic Pressure Application
The core of the process involves applying hydraulic pressure through the flexible diaphragm. The hydraulic fluid, which is stored in the system’s accumulator, is pumped into the bladder or diaphragm, and this fluid pressure is transmitted evenly over the surface of the metal sheet.
- Pressure Control: The pressure is gradually increased, and the process is highly controlled to ensure that the metal deforms uniformly without causing defects such as tearing, wrinkling, or thinning.
The pressure forces the sheet metal to conform to the shape of the die. The flexible diaphragm plays a critical role in distributing the pressure evenly over the entire surface of the sheet, allowing for uniform deformation and the formation of complex shapes without localized stress points.
3.4 Metal Forming and Shaping
As the hydraulic pressure builds, the sheet metal flows and stretches to fit the contours of the die. The flexibility of the diaphragm allows the metal to be pressed into deep recesses or drawn into complex shapes that would be difficult to achieve with conventional stamping methods.
- Deep Drawing Capabilities: In deep-draw hydroforming, the press can form deep, complex shapes with minimal thinning or defects, unlike traditional methods that often result in excessive thinning or tearing at sharp corners.
The ability to control material flow during the forming process is one of the key advantages of hydroforming. The press can produce parts with variable wall thickness, enabling thicker sections where strength is required and thinner sections where weight savings are prioritized.
3.5 Pressure Hold and Release
Once the metal has fully conformed to the die and reached the desired shape, the hydraulic pressure is held for a brief period to ensure the part stabilizes and the material fully takes on the form of the die.
- Pressure Holding: This is particularly important for parts with intricate details, where maintaining pressure allows for complete material flow and prevents springback or dimensional inaccuracies.
After the pressure hold phase, the hydraulic fluid is released from the diaphragm, and the press opens, allowing the flexible diaphragm to retract.
3.6 Part Removal
Once the hydraulic pressure is released, the formed part can be removed from the die. The result is a precise, high-quality part with smooth surfaces and minimal defects, ready for post-processing if needed.
- Minimal Finishing: Due to the smooth application of pressure and the absence of direct contact with hard tooling during forming, the final part typically requires minimal finishing. This reduces secondary processing costs and enhances production efficiency.
4. Pressure Control and Forming Accuracy
The success of sheet hydroforming relies heavily on pressure control. Pressure must be carefully managed to ensure that the metal deforms evenly and fills the die without over-stretching or wrinkling. Different parts and materials require varying pressure levels, depending on the complexity of the geometry and the material’s properties.
4.1 Gradual Pressure Increase
The pressure in a sheet hydroforming press is typically increased gradually to avoid sudden deformations that could cause defects. The system monitors and adjusts pressure in real-time, ensuring uniform deformation across the entire surface of the sheet metal.
- Pressure Ramping: Controlled ramp-up of pressure helps prevent localized thinning or tearing, especially when working with high-strength materials like steel or titanium.
4.2 Maximum Pressure
The maximum pressure applied during the process can vary depending on the material being formed and the complexity of the part. In some cases, pressures can exceed 10,000 psi, particularly for high-strength materials or deep-draw components.
5. Advantages of the Hydroforming Press Process
Sheet hydroforming offers several key advantages over traditional stamping or mechanical pressing methods:
- Complex Shapes: Sheet hydroforming presses excel at producing parts with complex geometries, including those with deep draws, sharp corners, and intricate contours.
- Uniform Material Thickness: The gradual application of pressure ensures that material thinning is minimized, resulting in stronger, more reliable parts.
- Fewer Tooling Requirements: Only a single-sided die is needed, reducing tooling costs and setup time compared to traditional stamping, which requires both a male and female die.
- High Surface Quality: The use of a flexible diaphragm means there is no direct contact between the metal and hard tooling, resulting in smooth surfaces with minimal defects, such as scratches or tool marks.
- Material Efficiency: The precise control of material flow leads to reduced waste, as the process uses the exact amount of material needed to form the part without excessive trimming.
Conclusion
Sheet hydroforming presses operate based on the principles of hydraulic pressure and uniform deformation, allowing manufacturers to create complex, high-precision parts with superior surface quality. By using a single-sided die and a flexible diaphragm, sheet hydroforming reduces tooling costs, minimizes material waste, and offers greater design flexibility than traditional metal forming processes. The ability to control pressure precisely ensures that parts are formed with high accuracy, making sheet hydroforming presses an ideal solution for industries that demand lightweight, durable, and intricately shaped components, such as automotive, aerospace, and medical devices.
Basic Process Steps of Sheet Hydroforming
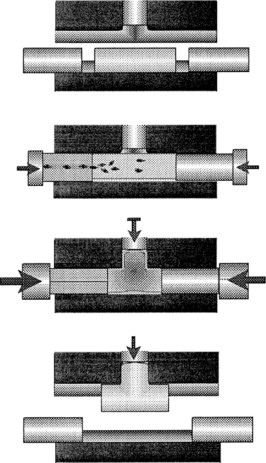
Sheet hydroforming is an advanced metal forming process that utilizes hydraulic pressure to shape sheet metal into complex geometries. This method is widely used in industries such as automotive, aerospace, consumer goods, and medical devices due to its ability to create precise, lightweight, and strong components with minimal defects. The process offers several advantages over traditional stamping, such as reduced tooling requirements, improved material utilization, and better surface quality. However, to fully understand the benefits of sheet hydroforming, it’s important to explore the fundamental steps involved in the process.
This guide will take a comprehensive look at the basic process steps of sheet hydroforming, from material preparation and die setup to hydraulic pressure application and part removal. Each step is critical to ensuring a successful and defect-free final product, with precise control over pressure, material flow, and dimensional accuracy.
1. Material Preparation
The first step in the sheet hydroforming process is the preparation of the metal sheet, also referred to as the blank. The material selection and preparation are critical factors in determining the success of the process and the quality of the final part.
1.1 Material Selection
The choice of material is dictated by the application requirements, including the need for strength, ductility, corrosion resistance, and weight reduction. Common materials used in sheet hydroforming include:
- Aluminum: Lightweight and highly ductile, making it ideal for automotive and aerospace applications where weight savings are crucial.
- Steel: Particularly advanced high-strength steels (AHSS), which offer high strength and durability, often used in safety-critical automotive components.
- Stainless Steel: Known for its corrosion resistance and strength, stainless steel is often used in medical devices and consumer products.
- Titanium: Lightweight, strong, and corrosion-resistant, titanium is frequently used in aerospace and medical industries for high-performance parts.
The material must have sufficient ductility to undergo plastic deformation without cracking or tearing during the hydroforming process. Additionally, the material’s thickness is carefully selected based on the part design and application.
1.2 Sheet Cutting
Once the appropriate material is selected, the metal sheet is cut into the desired dimensions, referred to as the blank. Precision in cutting the blank is essential to ensure that it fits correctly within the die and forms evenly during the process.
- Laser Cutting: This method is often used for precision cutting, especially when dealing with complex geometries or small parts.
- Waterjet Cutting: An alternative for thicker or more heat-sensitive materials that may be affected by the heat generated in laser cutting.
- Shearing or Sawing: For simpler parts or thicker materials, shearing or sawing may be used to cut the sheet into the correct dimensions.
After cutting, the edges of the sheet may need to be deburring to remove any sharp edges or rough areas that could affect the forming process.
1.3 Cleaning and Lubrication
Before the sheet is placed into the hydroforming press, it is essential to clean it thoroughly to remove any contaminants, such as oils, dirt, or oxidation. Contaminants on the surface of the sheet metal can cause defects like scratches or material buildup during forming, compromising the final part’s quality.
- Surface Cleaning: Cleaning may involve washing the sheet in a degreasing solution or using a mechanical cleaning process, depending on the material and contaminants present.
Next, lubrication is applied to the sheet to reduce friction between the metal and the die. Lubrication helps ensure smooth material flow during forming and minimizes the risk of damage to the die or the sheet metal. The type of lubricant used depends on the material and the complexity of the part being formed.
2. Die Setup and Clamping
The second step in the hydroforming process involves preparing the hydroforming press and setting up the die. This step is critical to ensure that the metal sheet is formed correctly and that the final part meets the required specifications.
2.1 Die Placement
In sheet hydroforming, a rigid die is used to define the shape of the final part. Unlike traditional stamping, which requires both a male and female die, sheet hydroforming only requires a single-sided die, simplifying the tooling setup.
- Die Design: The die is typically made of hardened steel or another durable material that can withstand the hydraulic pressure used in the process. It is designed to match the exact geometry of the part being formed, with considerations for material flow and dimensional tolerances.
The die is placed into the lower portion of the hydroforming press. It must be properly aligned and securely mounted to ensure accurate part formation and prevent movement during the application of hydraulic pressure.
2.2 Sheet Placement
Once the die is positioned, the prepared metal sheet (or blank) is placed on top of the die. Careful alignment of the sheet over the die is crucial for achieving uniform material flow and avoiding defects such as wrinkles or uneven thickness.
- Sheet Centering: The blank is centered over the die using positioning guides or markers. This ensures that the metal is evenly distributed across the die, allowing for consistent deformation during the forming process.
2.3 Clamping and Sealing
After the sheet is placed over the die, the flexible diaphragm or bladder of the hydroforming press is lowered over the sheet. The diaphragm is a key component that holds the hydraulic fluid and applies pressure to the sheet during forming.
- Clamping: The sheet is clamped securely between the die and the diaphragm, ensuring that it does not move or shift during the forming process. Proper clamping prevents misalignment and ensures that the sheet conforms precisely to the die’s shape.
- Sealing: A tight seal is created between the sheet and the die, allowing for uniform application of hydraulic pressure. This seal is essential to prevent hydraulic fluid from leaking out during pressurization and to ensure that the metal is evenly deformed.
3. Hydraulic Pressure Application
The core of the sheet hydroforming process involves the application of hydraulic pressure to the metal sheet. This step is where the metal undergoes plastic deformation, taking on the shape of the die.
3.1 Pressurization
With the sheet securely clamped in place, the hydraulic system of the press begins to apply pressure to the sheet through the diaphragm. Hydraulic fluid, typically oil or another high-pressure fluid, is pumped into the diaphragm, which in turn applies even pressure across the surface of the metal sheet.
- Gradual Pressure Increase: The hydraulic pressure is increased gradually to avoid sudden deformation or tearing of the material. The controlled application of pressure ensures that the metal deforms uniformly without introducing defects like wrinkles, thinning, or cracking.
3.2 Metal Forming
As the hydraulic pressure builds, the sheet metal is pressed into the die, forcing it to take on the desired shape. The flexibility of the diaphragm allows the metal to flow into complex geometries, including deep recesses, sharp corners, and intricate contours.
- Material Flow: The pressure forces the material to flow smoothly over the die, conforming to its shape without excessive thinning or buckling. The uniform pressure distribution is key to achieving high-quality parts with minimal surface defects.
- Deep Drawing: In cases where deep draws are required, such as in the automotive or aerospace industry, the press can apply higher pressures to achieve deep forming without tearing or thinning the material excessively. Sheet hydroforming is particularly well-suited for deep-draw applications where traditional stamping might fail due to material limitations.
4. Pressure Holding and Release
Once the metal has been formed into the desired shape, the hydraulic pressure is maintained for a short period to allow the material to stabilize.
4.1 Pressure Holding
The pressure holding phase ensures that the material fully conforms to the die and that any residual stresses are relieved. This step is particularly important for parts with intricate details, where dimensional accuracy and surface quality are critical.
- Stress Relief: Maintaining pressure allows the material to “settle” into the die, reducing the risk of springback or other distortions once the pressure is released.
- Dimensional Accuracy: Holding the pressure helps ensure that the part maintains its exact shape and dimensions, preventing deviations that could compromise the part’s fit or function.
4.2 Pressure Release
Once the holding phase is complete, the hydraulic pressure is gradually released, allowing the diaphragm to retract. This step must be controlled carefully to avoid any springback or deformation of the part as the pressure is removed.
- Gradual Depressurization: The hydraulic fluid is released in a controlled manner, and the diaphragm lifts away from the formed part. Quick or uneven release of pressure can lead to warping or other defects.
5. Part Removal and Finishing
After the pressure has been released, the formed part is ready to be removed from the press.
5.1 Part Removal
The flexible diaphragm is lifted, and the formed part is removed from the die. Care must be taken during part removal to avoid damaging the newly formed part, especially if the geometry includes thin sections or complex contours.
- Ejection Systems: Some hydroforming presses are equipped with automated ejection systems that gently lift the part from the die, reducing the risk of damage.
5.2 Inspection and Finishing
Once the part is removed, it undergoes inspection to ensure that it meets the required specifications in terms of shape, dimensions, and surface quality.
- Visual Inspection: The part is checked for defects such as cracks, wrinkles, or surface irregularities that could affect its performance or appearance.
- Dimensional Inspection: Precision measuring tools or automated scanning systems may be used to verify that the part meets the required tolerances.
In some cases, the part may require post-processing, such as trimming or surface finishing, to meet final product specifications. However, because sheet hydroforming produces parts with smooth surfaces and minimal defects, finishing requirements are typically minimal compared to traditional forming processes.
6. Post-Processing and Quality Control
Once the part has passed inspection, it may undergo post-processing operations depending on the requirements of the application. These operations could include:
- Trimming: Any excess material or “flash” from the forming process is trimmed away to ensure the part meets dimensional tolerances.
- Surface Finishing: In some applications, the surface of the part may be polished, coated, or otherwise treated to improve aesthetics, corrosion resistance, or wear resistance.
- Heat Treatment: In certain cases, parts may undergo heat treatment to further strengthen the material or relieve internal stresses caused by the forming process.
Quality control procedures ensure that every part meets the required specifications before it is shipped to the customer or moved to the next stage of production.
Conclusion
The basic process steps of sheet hydroforming include material preparation, die setup, hydraulic pressure application, pressure holding, and part removal, followed by any necessary post-processing. Each of these steps is critical in ensuring that the final part meets the desired specifications for strength, precision, and surface quality. Sheet hydroforming offers several advantages over traditional forming methods, including greater design flexibility, reduced tooling requirements, and improved material efficiency. By understanding and controlling each step of the process, manufacturers can produce high-quality parts that meet the rigorous demands of industries such as automotive, aerospace, and medical devices.
Components of Sheet Hydroforming Presses
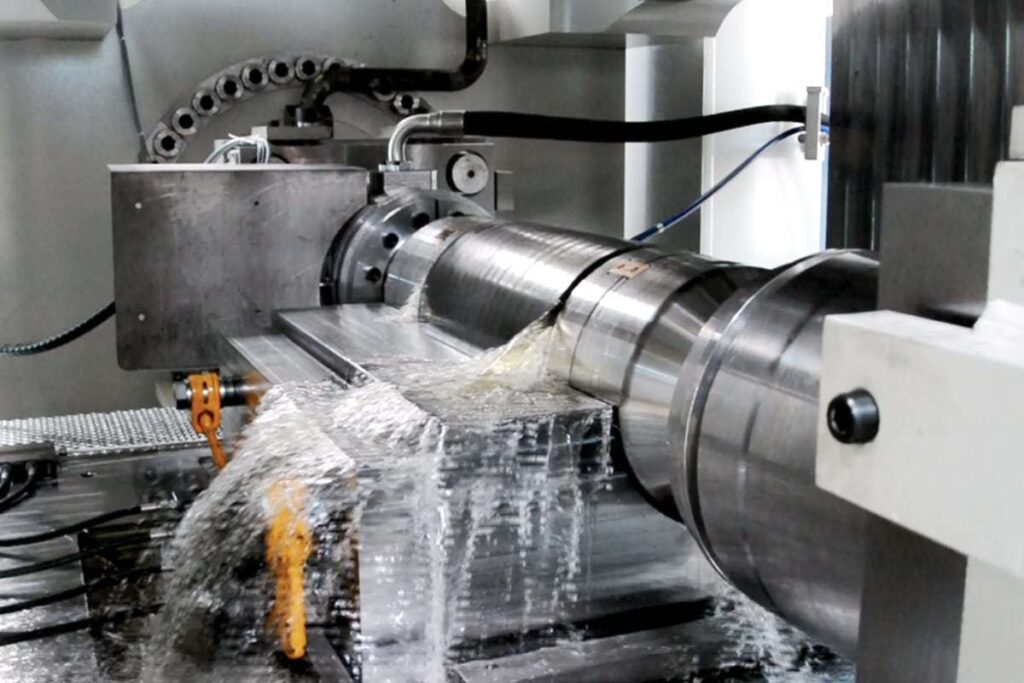
Sheet hydroforming presses are advanced machines designed for the metal forming process known as sheet hydroforming, where hydraulic pressure is used to form sheet metal into complex, high-precision parts. The press uses hydraulic fluid to force a sheet of metal against a rigid die, creating intricate shapes with fewer defects compared to traditional metal forming methods like stamping. The success of sheet hydroforming largely depends on the machine’s design and the individual components that make up the press.
This guide takes a detailed look at the key components of sheet hydroforming presses, explaining the function of each part and how it contributes to the overall performance and precision of the press. From the hydraulic system to the die and control units, each component plays a vital role in ensuring that the process runs smoothly, efficiently, and with the desired level of accuracy.
1. Hydraulic System
At the heart of any sheet hydroforming press is the hydraulic system, which generates the high pressures required to form metal sheets into the desired shapes. The hydraulic system consists of several subcomponents that work together to supply, regulate, and control the pressure applied to the sheet metal.
1.1 Hydraulic Pump
The hydraulic pump is the primary component that generates hydraulic pressure by moving fluid from a reservoir into the system. In sheet hydroforming presses, this pump needs to be powerful enough to generate pressures typically ranging from 10,000 to 20,000 psi (pounds per square inch), depending on the material and complexity of the part being formed.
- Types of Pumps: Hydraulic pumps in sheet hydroforming presses are often piston pumps or axial piston pumps, which are capable of delivering high pressures while maintaining efficiency.
- Flow Rate Control: The pump must be able to adjust its flow rate to match the requirements of the forming process. This ensures that the hydraulic fluid is supplied at the correct pressure and volume throughout the entire cycle.
1.2 Hydraulic Reservoir
The hydraulic reservoir is a large tank that stores the hydraulic fluid used in the system. This fluid is typically a type of oil that can withstand high pressures and temperatures without breaking down. The reservoir ensures that there is a constant supply of fluid available for the pump to draw from.
- Capacity: The reservoir must be sized appropriately to accommodate the fluid volume required for the press. In large presses, this may be several hundred liters or more.
- Cooling System: Since hydraulic systems generate heat during operation, many reservoirs are equipped with cooling systems that help maintain optimal fluid temperatures and prevent overheating.
1.3 Hydraulic Accumulator
The hydraulic accumulator is a critical component that stores pressurized fluid and releases it as needed to maintain consistent pressure during the forming process. Accumulators act as energy storage devices, allowing the system to maintain pressure even when the pump is not actively generating it.
- Pressure Stability: Accumulators smooth out pressure fluctuations, ensuring that the pressure applied to the metal sheet is consistent throughout the forming process. This is crucial for preventing defects such as thinning or wrinkling.
- Types of Accumulators: The most common types used in hydroforming presses are bladder accumulators, which use a bladder to separate the hydraulic fluid from a gas (usually nitrogen) that stores the pressure.
1.4 Hydraulic Valves
Hydraulic valves control the flow of fluid within the system, directing it to various parts of the press and regulating the pressure as needed. Several types of valves are used in hydroforming presses:
- Directional Control Valves: These valves control the direction in which hydraulic fluid flows, determining whether the press is building pressure, holding pressure, or releasing it.
- Pressure Relief Valves: These are safety devices that release excess fluid if the pressure in the system exceeds safe operating limits, preventing damage to the press.
- Flow Control Valves: These regulate the flow rate of hydraulic fluid to ensure that the correct amount of pressure is applied at each stage of the forming process.
1.5 Hydraulic Actuators and Cylinders
Hydraulic actuators convert the pressurized fluid energy into mechanical motion. In sheet hydroforming presses, hydraulic cylinders are the most common type of actuator. They apply force to the flexible diaphragm or bladder, compressing the fluid and pressing the sheet metal against the die.
- Single-Acting vs. Double-Acting Cylinders: Some presses use single-acting cylinders, which apply pressure in one direction, while others use double-acting cylinders, which apply pressure in both directions for greater control and precision.
2. Flexible Diaphragm
One of the most distinctive components of a sheet hydroforming press is the flexible diaphragm or bladder. This component replaces the upper die used in traditional stamping, creating a uniform, even pressure across the entire surface of the sheet metal.
2.1 Material and Construction
The diaphragm or bladder is typically made from rubber or urethane, materials chosen for their flexibility, durability, and ability to withstand high pressures. These materials can deform without tearing, making them ideal for applying pressure evenly across the sheet metal as it conforms to the die.
- Diaphragm Design: The diaphragm must be thick enough to resist bursting under pressure while maintaining enough flexibility to conform to complex geometries in the die.
2.2 Function of the Diaphragm
During the hydroforming process, the diaphragm is filled with hydraulic fluid, which then applies pressure to the sheet metal. The flexible nature of the diaphragm ensures that pressure is distributed uniformly across the metal surface, allowing the sheet to deform without localized stress concentrations.
- Uniform Pressure Application: The diaphragm’s flexibility ensures that pressure is applied evenly, which is critical for forming complex shapes without defects like wrinkling or tearing.
3. Die (Tooling)
The die is the mold that defines the final shape of the part being formed. In sheet hydroforming presses, only a single-sided die is required, unlike in traditional stamping, where both a male and female die are needed. The die is placed in the lower part of the press, and the sheet metal is pressed against it by the diaphragm.
3.1 Die Materials
Dies in sheet hydroforming presses are typically made from hardened steel, aluminum, or cast iron. The material choice depends on factors such as the material being formed, the complexity of the part, and the number of parts being produced.
- Hardened Steel: Often used for high-volume production runs where durability is essential. Steel dies can withstand the repeated high pressures of hydroforming without wearing down.
- Aluminum or Cast Iron: These materials are commonly used for low-volume production runs or for prototypes, where the cost of steel dies may not be justified.
3.2 Die Design
The die is custom-designed to match the geometry of the final part. This involves precise machining to create the necessary contours, bends, and depths that the sheet metal will be pressed into.
- Single-Sided Die: Unlike in traditional stamping, where both male and female dies are used, sheet hydroforming requires only one rigid die. The hydraulic pressure applied by the diaphragm forces the metal into the shape defined by this single die.
3.3 Tooling Costs
One of the major advantages of sheet hydroforming is the reduced tooling costs. Since only one die is required, and no matching upper die is needed, the tooling costs are significantly lower compared to traditional forming methods.
4. Clamping and Sealing Systems
Clamping and sealing systems are essential for ensuring that the sheet metal remains securely positioned over the die during the hydroforming process. Proper clamping prevents the sheet from shifting, while sealing ensures that the hydraulic pressure is applied uniformly across the sheet.
4.1 Clamping Mechanisms
The clamping system holds the metal sheet in place over the die. It ensures that the sheet does not move or deform improperly during the forming process.
- Hydraulic Clamping: Most hydroforming presses use hydraulic clamps that apply uniform force around the edges of the metal sheet to hold it securely in place.
- Mechanical Clamps: In some cases, mechanical clamps are used, particularly for smaller presses or simpler parts. These are generally less flexible than hydraulic clamps but can be sufficient for straightforward applications.
4.2 Sealing Systems
The sealing system ensures that the pressurization process is fully contained, preventing hydraulic fluid from leaking out of the diaphragm and ensuring that the entire surface of the sheet metal is evenly pressurized.
- Rubber Seals: High-pressure rubber seals are commonly used to create a tight seal between the sheet, the die, and the diaphragm, ensuring no loss of pressure during forming.
5. Control Systems
Modern sheet hydroforming presses are equipped with sophisticated control systems that manage the hydraulic pressure, regulate the forming process, and monitor the performance of the press. These control systems are critical for achieving the precision and repeatability required in industries such as aerospace and automotive.
5.1 CNC (Computer Numerical Control) Systems
Many sheet hydroforming presses use CNC control systems, which automate key aspects of the forming process, such as pressure regulation, cycle timing, and die movement. These systems allow for precise control over each stage of the process, ensuring that parts are formed to exact specifications.
- Multi-Axis Control: In presses with multi-axis movement, CNC systems control the movement of the die or diaphragm along multiple axes, enabling the production of more complex geometries.
5.2 Programmable Logic Controllers (PLCs)
PLCs are used to automate the operation of the press, controlling the timing of hydraulic pressure application, clamping, and part removal. PLCs are essential for ensuring that each part is formed consistently and that the press operates efficiently.
- Process Automation: PLCs automate repetitive tasks, such as cycling the press, maintaining hydraulic pressure, and controlling fluid flow. This reduces the need for manual intervention and increases productivity.
5.3 Sensors and Monitoring
Modern sheet hydroforming presses are equipped with various sensors that monitor critical parameters such as pressure, temperature, fluid levels, and die alignment. These sensors feed data back to the control system, allowing real-time adjustments to be made during the forming process.
- Pressure Sensors: These sensors monitor the hydraulic pressure applied to the sheet metal, ensuring that it remains within the desired range for the specific material and part.
- Temperature Sensors: In cases where temperature control is important, sensors monitor the temperature of the hydraulic fluid and the metal sheet to prevent overheating or damage.
6. Ejection Systems
Once the part has been formed and the pressure released, the final component must be safely removed from the die. Ejection systems in sheet hydroforming presses help lift the part out of the die without damaging it.
6.1 Hydraulic Ejectors
Many hydroforming presses use hydraulic ejectors that push the formed part out of the die after the pressure has been released. These ejectors ensure that the part is removed without damage, especially in cases where the geometry of the part makes it difficult to lift manually.
6.2 Robotic Ejection Systems
In automated production lines, robotic ejection systems may be used to remove the part from the press and transport it to the next stage of production. These systems can be programmed to handle parts with precision, reducing the risk of damage and improving overall efficiency.
7. Safety Systems
Given the high pressures involved in sheet hydroforming, safety is a top priority. Modern hydroforming presses are equipped with a variety of safety features to protect operators and prevent damage to the machine.
7.1 Safety Guards and Enclosures
Physical barriers such as safety guards and machine enclosures are used to protect operators from the high-pressure systems and moving parts of the press. These guards are typically equipped with interlocks that prevent the press from operating if they are open.
7.2 Pressure Relief Systems
In the event of a pressure spike or system failure, pressure relief valves automatically release excess fluid to prevent the system from exceeding safe pressure limits. This helps protect both the machine and the operator from potential hazards.
Conclusion
The components of a sheet hydroforming press work together to create a highly efficient and precise metal forming machine capable of producing complex, high-quality parts. From the hydraulic system and flexible diaphragm to the die and control systems, each element plays a critical role in ensuring the success of the hydroforming process. By understanding how these components function and interact, manufacturers can optimize their operations, reduce costs, and improve part quality across a wide range of industries, including automotive, aerospace, and medical devices. As technology continues to advance, sheet hydroforming presses are likely to become even more versatile and capable, enabling the production of increasingly complex and lightweight components.
Applications of Sheet Hydroforming Presses
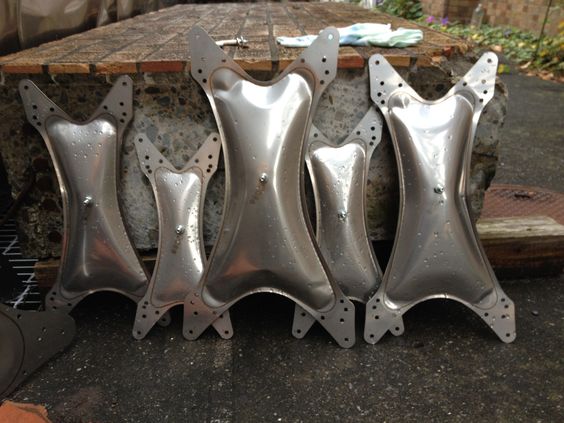
Sheet hydroforming is an advanced metal forming process that leverages hydraulic pressure to shape sheet metal into complex, high-precision components. This method is widely used across a variety of industries, including automotive, aerospace, medical devices, consumer electronics, and more. The main advantage of sheet hydroforming is its ability to create lightweight, durable, and intricately shaped components with minimal tooling and surface defects compared to traditional metal forming processes such as stamping or mechanical pressing.
Sheet hydroforming presses, which are designed specifically for this process, enable the efficient and cost-effective production of parts that require high dimensional accuracy, complex geometries, and excellent surface finishes. This article explores the key applications of sheet hydroforming presses, focusing on how they are used across different industries, the benefits they offer, and specific examples of their use in creating high-performance components.
1. Automotive Industry
The automotive industry is one of the largest adopters of sheet hydroforming technology. As the demand for lightweight, fuel-efficient, and crash-resistant vehicles grows, sheet hydroforming has become a critical process in the production of automotive components. By using hydraulic pressure to shape metal sheets, manufacturers can create parts that are both lightweight and structurally strong, improving vehicle performance and safety.
1.1 Body Panels and Exterior Components
Sheet hydroforming presses are widely used to produce automotive body panels and other exterior components. These parts require high levels of precision and smooth surface finishes, making hydroforming an ideal process for their production. Hydroformed body panels are often used in luxury and high-performance vehicles where weight reduction and aerodynamics are critical.
- Hoods, Doors, and Trunk Lids: Hydroforming is commonly used to manufacture car hoods, doors, and trunk lids. The ability to form complex, curved surfaces with minimal tooling reduces production costs and ensures a high-quality surface finish, reducing the need for additional surface treatments or polishing.
- Fenders and Bumpers: Fenders and bumpers are often hydroformed to create sleek, aerodynamic shapes that improve the overall aesthetics and performance of the vehicle. These components are also designed to absorb impact in the event of a collision, contributing to vehicle safety.
1.2 Chassis and Structural Components
In addition to body panels, sheet hydroforming presses are used to create chassis components and other structural parts that contribute to the overall strength and rigidity of the vehicle. These components must be lightweight yet capable of withstanding the stresses and forces experienced during vehicle operation, particularly in high-performance or off-road applications.
- Subframes and Crossmembers: Hydroforming is used to create subframes and crossmembers, which form the backbone of the vehicle’s structure. These components must be strong enough to support the vehicle’s weight while providing flexibility and impact resistance. Hydroforming allows for the production of lightweight, high-strength parts that meet these requirements.
- Crash Structures and Crumple Zones: Crash structures and crumple zones are critical safety components that absorb energy during a collision, protecting the occupants of the vehicle. Hydroformed parts in these areas are designed to deform in a controlled manner, dissipating energy and reducing the impact force on passengers.
1.3 Lightweighting for Electric Vehicles (EVs)
With the rise of electric vehicles (EVs), manufacturers are increasingly focused on reducing vehicle weight to improve battery efficiency and extend driving range. Sheet hydroforming plays a key role in the lightweighting of EVs, allowing manufacturers to create lightweight structural components that meet performance and safety standards without compromising strength.
- Battery Enclosures: Hydroforming is used to create lightweight, structurally sound battery enclosures that protect the vehicle’s battery pack while minimizing weight. The ability to form intricate shapes with high precision ensures that the enclosure fits tightly around the battery, optimizing space and efficiency.
- Suspension Components: EVs require lightweight suspension components to offset the weight of the battery pack. Hydroformed suspension arms, control arms, and other components are used to reduce weight and improve vehicle handling.
2. Aerospace Industry
The aerospace industry is another major user of sheet hydroforming presses, as the process enables the production of lightweight, high-strength components that are essential for aircraft performance and fuel efficiency. Aircraft must be designed with both strength and lightweight properties to maximize fuel efficiency, range, and payload capacity, making hydroforming an ideal solution for manufacturing structural and non-structural aerospace components.
2.1 Fuselage and Structural Components
One of the most common applications of sheet hydroforming in aerospace is the production of fuselage components and other structural elements that contribute to the aircraft’s overall frame and stability. These parts must withstand extreme loads and stresses during flight, and hydroforming allows manufacturers to create highly complex shapes that are both lightweight and strong.
- Fuselage Frames: Hydroformed fuselage frames provide the structural support for the aircraft’s outer skin. The precision and strength offered by hydroforming make these components crucial for maintaining the integrity of the aircraft during flight.
- Stringers and Longerons: Stringers and longerons, which are used to reinforce the fuselage and wings, are often hydroformed to ensure they meet the aircraft’s weight and strength requirements. These components need to be lightweight while offering the necessary rigidity to maintain the aircraft’s aerodynamic shape and structural integrity.
2.2 Wing Components
Wing components are another area where sheet hydroforming is commonly used. The wing structure must support the weight of the aircraft and handle various aerodynamic forces during flight, making it essential that these components are both lightweight and durable.
- Wing Ribs: Hydroforming is used to create wing ribs, which support the overall structure of the wing and distribute the loads experienced during flight. Hydroforming ensures that these components are lightweight while providing the necessary strength to support the aircraft.
- Spars and Struts: Spars and struts are critical structural elements that reinforce the wings and help distribute the forces experienced during takeoff, landing, and flight. Hydroforming is ideal for producing these parts due to its ability to form complex, strong, and lightweight components.
2.3 Engine Components
In addition to structural components, sheet hydroforming is used in the production of aircraft engine components. These parts must be able to withstand extreme temperatures and pressures, making material strength and precision critical.
- Turbine Housings and Ducts: Hydroformed components are used in turbine housings and air ducts, where precise shapes are required to ensure efficient airflow and optimal engine performance. The ability to create seamless parts with uniform wall thicknesses ensures that these components perform reliably under demanding conditions.
2.4 Fuel and Hydraulic Systems
Fuel lines, hydraulic tubes, and other fluid handling components are often hydroformed to create lightweight, seamless parts that can withstand the high pressures and temperatures found in aerospace applications. Hydroforming allows for the production of complex, thin-walled tubes that offer high precision and leak resistance, ensuring the safe and efficient operation of fuel and hydraulic systems.
3. Medical Devices
In the medical field, precision, surface finish, and material quality are critical factors in the design and manufacture of medical devices. Sheet hydroforming presses are commonly used to create high-precision components for surgical instruments, diagnostic equipment, and implants, ensuring that these devices meet stringent regulatory requirements for performance, safety, and biocompatibility.
3.1 Surgical Instruments
One of the primary applications of sheet hydroforming in the medical field is the production of surgical instruments. These instruments must be lightweight, strong, and easy to clean, making hydroforming an ideal process for their manufacture. Hydroformed instruments often have smooth, seamless surfaces, reducing the risk of contamination and making them easier to sterilize.
- Forceps, Scalpels, and Retractors: Hydroforming is used to create precision surgical tools such as forceps, scalpels, and retractors. The ability to form thin, strong, and lightweight components ensures that these tools meet the necessary performance and hygiene standards required in the operating room.
3.2 Medical Implants
Medical implants such as orthopedic devices, dental implants, and joint replacements require materials that are both biocompatible and strong enough to withstand the forces and stresses they will experience inside the human body. Hydroforming allows for the production of implants with customized shapes and tight tolerances, ensuring that they fit perfectly within the patient’s anatomy.
- Hip and Knee Replacements: Hydroformed components are used in joint replacement devices, where the precision of the forming process ensures a custom fit for each patient, improving the performance and longevity of the implant.
- Spinal Implants: Hydroforming is also used to produce spinal implants, which require highly intricate geometries and exacting tolerances to ensure proper alignment and function.
3.3 Diagnostic Equipment
In addition to surgical instruments and implants, sheet hydroforming is used to produce components for diagnostic equipment such as MRI machines, X-ray equipment, and other medical devices. These devices require parts with precise dimensions and smooth surfaces to ensure accurate readings and long-term durability.
- Casing and Housings: Hydroforming is often used to create the outer casings and housings of diagnostic equipment, where the smooth, seamless surfaces produced by the process help maintain the cleanliness and sterility of the device.
- Electromagnetic Shields: For devices like MRI machines, hydroformed components are used to create electromagnetic shields that protect sensitive electronics from interference, ensuring accurate diagnostic readings.
4. Consumer Electronics and Appliances
Sheet hydroforming presses are also widely used in the production of consumer electronics and appliances, where the ability to create lightweight, aesthetically pleasing, and durable components is essential. The process is especially useful for creating the sleek, modern designs that are popular in today’s consumer products.
4.1 Consumer Electronics
In the world of consumer electronics, sheet hydroforming is used to manufacture the housings, frames, and structural components of devices like smartphones, laptops, and tablets. These products require thin, lightweight parts with precise geometries, making hydroforming an ideal solution.
- Smartphone Housings: Hydroformed aluminum and stainless steel housings are commonly used in high-end smartphones, where a combination of strength, durability, and design aesthetics is required. The smooth surface finish and seamless construction provided by hydroforming ensure that the device has a premium look and feel.
- Laptop Frames: Hydroforming is also used to create the frames and chassis of laptops, providing the necessary structural support while maintaining a slim, lightweight design.
4.2 Home Appliances
The appliance industry benefits from sheet hydroforming presses for the production of kitchen appliances, laundry machines, and other home goods. The ability to form large, complex parts with smooth surfaces and minimal defects makes hydroforming ideal for creating both functional and aesthetically pleasing components.
- Refrigerator Doors and Panels: Hydroforming is used to create the outer panels and doors of refrigerators, where the process ensures a smooth, high-quality surface finish that is resistant to corrosion and easy to clean.
- Oven Liners and Control Panels: In ovens and other kitchen appliances, hydroformed components are used to create control panels and interior liners that can withstand high temperatures while maintaining their shape and finish.
5. Sporting Goods and Bicycles
In the world of sporting goods and bicycles, weight, performance, and durability are critical factors in product design. Sheet hydroforming presses are used to manufacture components that enhance the performance of sports equipment and bicycles by reducing weight without sacrificing strength.
5.1 Bicycle Frames
One of the most common applications of sheet hydroforming in the sporting goods industry is the production of bicycle frames. Hydroforming allows manufacturers to create lightweight, strong frames with complex geometries that improve the aerodynamics and performance of the bike.
- Mountain Bikes and Road Bikes: Hydroformed aluminum and titanium frames are used in both mountain bikes and road bikes, where the ability to create tapered tubes and customized shapes enhances both the strength and comfort of the bike.
- Electric Bicycles (e-Bikes): With the rise of e-bikes, hydroforming has become even more important, allowing manufacturers to integrate battery compartments and electrical components into the frame without adding unnecessary weight.
5.2 Golf Clubs and Other Sporting Goods
In addition to bicycles, hydroforming is used in the production of golf clubs, hockey sticks, tennis rackets, and other sports equipment where lightweight, high-strength materials are essential for performance.
- Golf Club Shafts: Hydroforming allows for the creation of precision-engineered golf club shafts, where the weight distribution and stiffness can be tailored to improve the player’s swing and overall performance.
- Tennis Rackets and Hockey Sticks: The ability to create lightweight, durable frames with minimal material wastage makes hydroforming ideal for producing high-performance tennis rackets and hockey sticks.
6. Energy and Power Generation
The energy and power generation industry also benefits from the use of sheet hydroforming presses, particularly in the production of components for wind turbines, solar energy systems, and oil and gas infrastructure. The process allows for the creation of large, complex parts that can withstand harsh environmental conditions and demanding operational requirements.
6.1 Wind Turbine Components
In wind energy systems, sheet hydroforming is used to produce structural components for wind turbines, including the hub, nacelle, and blades. These components must be both lightweight and durable to maximize energy output and withstand the elements.
- Nacelle Housings: Hydroformed nacelle housings provide the necessary protection for the sensitive electrical components inside the turbine, while maintaining a streamlined design that reduces wind resistance.
- Blade Root Fittings: Hydroforming is used to create the root fittings that connect the wind turbine blades to the hub, ensuring a secure, durable connection that can withstand the forces generated by the spinning blades.
6.2 Solar Energy Components
In solar energy systems, hydroforming is used to create mounting brackets and frames for solar panels, ensuring that they are lightweight, durable, and able to withstand harsh weather conditions.
- Solar Panel Frames: Hydroformed frames provide the necessary support for solar panels, while maintaining a lightweight design that reduces installation costs and improves energy efficiency.
Conclusion
Sheet hydroforming presses are highly versatile machines that are used in a wide range of industries to produce lightweight, high-precision components with complex geometries. From automotive and aerospace applications to medical devices, consumer electronics, and sporting goods, the ability to form intricate shapes with minimal defects and high material efficiency makes sheet hydroforming an invaluable tool for modern manufacturing. As industries continue to demand more efficient, sustainable, and high-performance components, sheet hydroforming will likely play an even greater role in shaping the future of product design and production.
Deep Draw Sheet Hydroforming Press: Overview, Process, and Applications
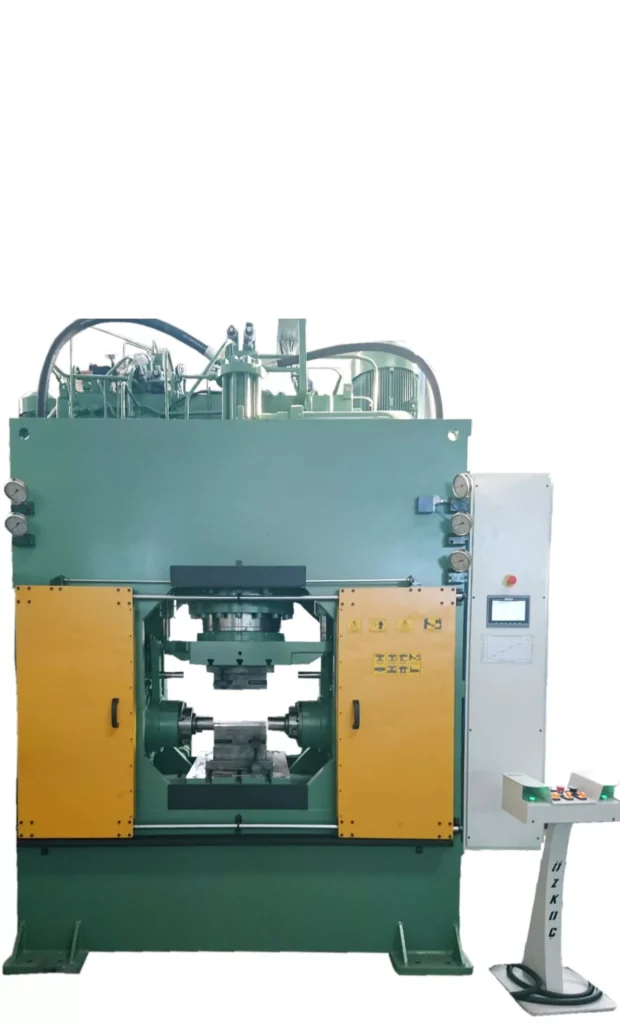
A deep draw sheet hydroforming press is a specialized machine used for forming complex, high-precision parts from metal sheets by applying hydraulic pressure. Unlike conventional stamping or drawing processes that use mechanical forces and matching male-female dies, deep draw hydroforming presses use a single die and hydraulic fluid pressure to shape the material. This advanced process is particularly effective for deep drawing operations, where the sheet metal is stretched into deep and complex shapes, often with minimal thinning or material waste.
Deep draw sheet hydroforming presses offer several advantages, such as reduced tooling costs, improved part quality, and the ability to create complex shapes with fewer defects. These benefits make the technology highly valuable in industries such as automotive, aerospace, medical devices, and consumer electronics.
1. What Is Deep Drawing?
Deep drawing is a manufacturing process in which a sheet metal blank is radially drawn into a forming die by a mechanical punch, typically resulting in a part with a greater depth than diameter. Commonly used to form hollow, cylindrical shapes like cups, cans, and tanks, deep drawing is widely used across various industries to manufacture parts that require significant depth.
In a conventional deep drawing process, the metal is mechanically drawn into the die using a punch, often resulting in defects like thinning, wrinkling, or tearing due to uneven material flow. However, deep draw hydroforming addresses these issues by replacing mechanical force with hydraulic pressure, offering better control over the material during the forming process.
2. Working Principles of Deep Draw Sheet Hydroforming Presses
The core principle of a deep draw sheet hydroforming press lies in the controlled application of hydraulic pressure, which shapes the metal sheet into the desired form by forcing it against a die. The press uses a flexible diaphragm or bladder filled with hydraulic fluid to apply pressure uniformly across the sheet metal.
2.1 Basic Process Steps
- Material Placement: A flat metal sheet (blank) is placed over the die. The material may vary based on the application but commonly includes aluminum, steel, stainless steel, or titanium.
- Clamping and Sealing: The blank is securely clamped to prevent movement during the forming process. The upper section of the press, containing the flexible diaphragm, is then lowered over the metal sheet, sealing the blank in place.
- Hydraulic Pressure Application: Hydraulic fluid is pumped into the diaphragm, which expands and applies uniform pressure across the surface of the metal sheet. This pressure forces the sheet to stretch into the die cavity, forming the desired deep shape.
- Material Flow and Stretching: As pressure builds, the material is drawn into the die, conforming to its shape without the need for a matching upper die. The hydraulic pressure ensures that the material flows smoothly, minimizing defects such as wrinkling or tearing.
- Pressure Holding and Release: Once the part has taken its final shape, the pressure is maintained briefly to allow the material to stabilize. Afterward, the hydraulic pressure is gradually released, and the diaphragm retracts.
- Part Removal: The clamping system is opened, and the deep-drawn part is removed from the die. The part is now ready for post-processing or further inspection.
2.2 Hydraulic Pressure Control
One of the key features of deep draw hydroforming presses is the ability to precisely control the hydraulic pressure applied during forming. The gradual increase in pressure allows the material to stretch uniformly, reducing the likelihood of defects such as material thinning or excessive wrinkling.
- Uniform Pressure Distribution: The hydraulic fluid ensures that pressure is applied evenly across the entire surface of the blank, allowing for uniform material deformation.
- Multi-Stage Pressure Application: In some cases, the pressure may be applied in multiple stages to ensure better control over material flow, especially for complex shapes or parts with variable wall thicknesses.
3. Key Components of Deep Draw Sheet Hydroforming Presses
Deep draw sheet hydroforming presses consist of several key components that work together to ensure the precise and efficient forming of metal parts. Each of these components plays a crucial role in maintaining the press’s performance and accuracy.
3.1 Hydraulic System
The hydraulic system is the core of the deep draw hydroforming press, generating the pressure needed to form the sheet metal into complex shapes. It typically includes the following components:
- Hydraulic Pumps: High-pressure hydraulic pumps provide the force required to move the hydraulic fluid into the diaphragm and exert pressure on the metal sheet.
- Accumulators: These store pressurized fluid and release it as needed, maintaining a consistent pressure throughout the forming process.
- Valves: Hydraulic control valves regulate the flow of fluid, ensuring precise control over the pressure levels at each stage of the process.
3.2 Diaphragm (Flexible Bladder)
The flexible diaphragm or bladder is a critical component in the hydroforming process. Made from durable materials such as urethane or rubber, the diaphragm holds the hydraulic fluid and transmits the pressure uniformly across the sheet metal.
- Durability: The diaphragm must be able to withstand repeated cycles of high pressure without rupturing or losing its flexibility.
- Uniform Pressure Application: Its primary function is to ensure even pressure distribution across the blank, preventing localized stress that could lead to defects.
3.3 Die (Tooling)
The die is the mold that defines the final shape of the deep-drawn part. In deep draw hydroforming, a single-sided die is used to form the part, which reduces tooling costs compared to traditional methods that require both a male and female die.
- Die Material: Dies are typically made from hardened steel, aluminum, or cast iron, depending on the production volume and material being formed.
- Custom Die Design: Each die is custom-designed to match the geometry of the part, ensuring accurate and repeatable results.
3.4 Clamping System
The clamping system holds the sheet metal blank in place over the die during the hydroforming process. Proper clamping ensures that the material does not shift or wrinkle during forming.
- Hydraulic or Mechanical Clamps: Clamping systems may be hydraulic or mechanical, depending on the press design. Hydraulic clamps provide more even pressure distribution and are commonly used in high-precision applications.
3.5 Control System
Modern deep draw hydroforming presses are equipped with computerized control systems that allow operators to manage every aspect of the process, from pressure levels to cycle timing.
- CNC (Computer Numerical Control): Many presses use CNC systems to control the pressure application and movement of the press components, ensuring repeatable and accurate part production.
- Real-Time Monitoring: Sensors monitor critical parameters, such as pressure, temperature, and material deformation, allowing real-time adjustments to prevent defects and ensure quality.
4. Applications of Deep Draw Sheet Hydroforming Presses
Deep draw sheet hydroforming presses are used across various industries, offering unique advantages in producing lightweight, high-strength components with complex geometries. Below are some of the most common applications.
4.1 Automotive Industry
The automotive industry is one of the largest users of deep draw hydroforming technology. As manufacturers strive to reduce vehicle weight while maintaining safety and performance standards, deep draw hydroforming plays a critical role in producing lightweight yet strong components.
- Fuel Tanks and Fluid Reservoirs: Deep-drawn fuel tanks and fluid reservoirs benefit from the process’s ability to create seamless, leak-resistant parts with complex shapes.
- Heat Shields and Engine Covers: Hydroforming allows for the precise shaping of heat shields and engine covers, which require tight tolerances and smooth surfaces to ensure optimal performance.
- Structural Components: Chassis parts, crossmembers, and other structural components are often deep-drawn using hydroforming presses, as the process allows for reduced material thickness while maintaining strength.
4.2 Aerospace Industry
In the aerospace industry, reducing weight is essential for improving fuel efficiency and performance. Deep draw hydroforming is used to create high-precision parts that meet stringent performance and safety standards.
- Aircraft Skin Panels: Hydroforming is used to produce lightweight, seamless skin panels that provide aerodynamic surfaces while reducing material wastage.
- Landing Gear Components: Deep-drawn hydroformed parts are used in landing gear, where they must withstand high stress and repeated impact during landing.
- Engine Components: The process is also used to manufacture parts for aircraft engines, such as turbine housings and exhaust components, where high-strength materials and precise shapes are required.
4.3 Medical Devices
In the medical industry, deep draw hydroforming is used to produce components that require high levels of precision, biocompatibility, and surface quality.
- Surgical Instruments: The process is used to create lightweight, durable surgical tools such as forceps, scalpels, and retractor blades, where precision and smooth finishes are critical.
- Implants: Custom-designed implants, such as joint replacements and spinal devices, benefit from the process’s ability to form intricate, biocompatible shapes with minimal defects.
4.4 Consumer Goods
In the consumer goods sector, deep draw hydroforming is used to create components for household appliances, electronics, and other consumer products.
- Appliance Housings: Seamless, lightweight housings for appliances such as washing machines, dryers, and refrigerators are often hydroformed to provide both structural strength and aesthetic appeal.
- Electronics: In the electronics industry, deep draw hydroforming is used to create lightweight, durable enclosures for devices such as smartphones, laptops, and tablets.
5. Advantages of Deep Draw Sheet Hydroforming Presses
Deep draw hydroforming presses offer numerous advantages over traditional mechanical forming methods. These include:
5.1 Reduced Tooling Costs
Because deep draw hydroforming requires only a single-sided die, tooling costs are significantly lower than in conventional methods that use both a male and female die. This makes hydroforming a cost-effective option, especially for low to medium production runs.
5.2 Improved Part Quality
The uniform pressure distribution in hydroforming results in parts with smoother surfaces and better dimensional accuracy than those produced through mechanical stamping or drawing. The process also minimizes the risk of defects such as wrinkling, tearing, and thinning, ensuring high-quality final products.
5.3 Greater Design Flexibility
Hydroforming offers greater design flexibility, allowing manufacturers to produce parts with complex geometries, deep draws, and intricate contours. This is particularly beneficial in industries like automotive and aerospace, where lightweight, high-performance components are essential.
5.4 Material Efficiency
Hydroforming allows for efficient material usage by minimizing waste and ensuring uniform wall thickness across the entire part. This reduces the overall material cost and improves the structural integrity of the part.
Conclusion
Deep draw sheet hydroforming presses are an essential tool for modern manufacturing, providing a cost-effective, precise, and versatile method for producing complex, high-strength components. Whether used in the automotive, aerospace, medical, or consumer goods industries, deep draw hydroforming enables manufacturers to create lightweight, high-performance parts with minimal defects and reduced material waste. The ability to control hydraulic pressure precisely and form intricate geometries makes deep draw hydroforming a preferred choice for producing parts that require a combination of strength, durability, and precision. As industries continue to demand more efficient and lightweight components, the use of deep draw sheet hydroforming presses will likely continue to grow, driving innovation in both machine design and production capabilities.
What Is Deep Drawing?
Deep drawing is a metal forming process used to manufacture hollow, cylindrical, or complex-shaped parts from flat sheet metal. The process involves radially drawing a sheet metal blank into a forming die using a punch, causing the material to stretch and deform into the shape of the die cavity. Deep drawing is commonly used in various industries to produce parts such as cans, cups, automotive components, appliance parts, and medical devices. The term “deep” refers to the fact that the depth of the part produced is greater than its diameter.
The deep drawing process is distinguished by its ability to create seamless, high-precision, and lightweight parts in a single operation or through multiple drawing stages. It is favored over other metal forming techniques when manufacturers need parts that require significant depth, smooth surfaces, and high strength-to-weight ratios.
1. The Deep Drawing Process: Step-by-Step
The deep drawing process transforms flat sheet metal into a hollow, three-dimensional shape by stretching the material. Below are the basic steps involved in the deep drawing process:
1.1 Blank Preparation
- Sheet Metal Selection: The process begins with a flat sheet of metal, commonly called a blank. The blank is typically cut into a circular shape and must be of a material with good formability, such as aluminum, steel, stainless steel, or copper.
- Blank Size: The size of the blank depends on the dimensions of the desired part. The blank needs to be large enough to stretch and fill the die cavity during the deep drawing process.
1.2 Die and Punch Setup
- Punch and Die: The primary tools in the deep drawing process are the punch and the die. The die defines the outer shape of the part, while the punch pushes the metal blank into the die cavity, forming the internal shape of the part.
- Blank Holder (or Binder): A blank holder is used to clamp the blank in place over the die. The blank holder prevents the sheet metal from wrinkling as it is drawn into the die cavity. The force applied by the blank holder is critical to achieving a smooth part without defects.
1.3 Drawing Stage
- Punch Movement: During the drawing stage, the punch moves downward, applying force to the center of the blank. As the punch pushes the blank into the die, the material is forced to stretch and flow around the punch, taking the shape of the die cavity.
- Metal Flow: The metal sheet experiences a combination of tension and compression as it is drawn. The material flows from the edges toward the center, and any excess material is absorbed by the blank holder to prevent wrinkling.
- Single or Multiple Draws: For shallow parts, a single drawing operation is often sufficient. However, for deeper parts, multiple stages (also known as redrawing) may be required to gradually shape the material without causing excessive thinning, tearing, or failure.
1.4 Ejection and Part Removal
- Ejecting the Part: Once the punch reaches the bottom of the stroke, the metal has been fully formed into the shape of the die cavity. The punch retracts, and the newly formed part is removed from the die.
- Finishing: After the part is ejected, it may require finishing operations such as trimming to remove excess material, deburring to smooth edges, or polishing for surface finishing.
2. Materials Used in Deep Drawing
The deep drawing process can be used with a wide range of metals, but the material must possess certain properties, such as ductility and formability, to prevent failure during the drawing process. The most commonly used materials include:
- Aluminum: Lightweight and corrosion-resistant, aluminum is frequently used for deep drawing applications in automotive, aerospace, and packaging industries.
- Low-Carbon Steel: This material has good formability and strength, making it ideal for producing deep-drawn parts like automotive body panels, engine components, and cans.
- Stainless Steel: Known for its corrosion resistance and strength, stainless steel is used in the food, medical, and chemical industries for components like kitchenware, surgical instruments, and chemical containers.
- Copper and Brass: Both materials are highly ductile and often used in electrical, plumbing, and decorative applications.
The thickness of the material also plays a critical role in the deep drawing process. Thinner sheets are easier to draw but may require multiple stages to avoid excessive thinning, while thicker sheets are more resistant to tearing but require more force to shape.
3. Deep Drawing Challenges and Defects
While deep drawing is an efficient process for producing high-precision parts, it presents several challenges. Manufacturers must carefully control the process to avoid common defects, such as:
3.1 Wrinkling
- Cause: Wrinkling occurs when excess material is not properly controlled by the blank holder, causing folds or ridges to form on the walls of the part.
- Prevention: Proper control of the blank holder force is essential to prevent wrinkling. Too much or too little pressure can lead to material instability during the drawing process.
3.2 Tearing and Fracturing
- Cause: If the material is overstretched during the drawing process, it may tear or fracture. This often happens when the blank is too thick or when insufficient lubrication is used, causing excessive friction between the punch and the blank.
- Prevention: To avoid tearing, manufacturers may use multiple drawing stages, where the material is gradually stretched, or they may select a material with better ductility.
3.3 Thinning
- Cause: Thinning occurs when the material becomes too stretched in certain areas, particularly along the sidewalls of the part. This can weaken the part and affect its structural integrity.
- Prevention: Thinning can be minimized by adjusting the draw ratio (the relationship between the blank diameter and the punch diameter) and ensuring uniform material flow.
3.4 Springback
- Cause: After forming, some materials may return to their original shape due to residual stresses, leading to dimensional inaccuracies.
- Prevention: Springback can be minimized by carefully selecting the material and optimizing the process parameters, such as punch speed and holding pressure.
4. Applications of Deep Drawing
Deep drawing is used in a wide range of industries to produce parts that require complex shapes, seamless construction, and precise dimensional tolerances. Some of the most common applications include:
4.1 Automotive Industry
Deep drawing is extensively used in the automotive industry to manufacture body panels, fuel tanks, exhaust components, and engine housings. Automotive parts require high strength and precision, and deep drawing ensures the production of lightweight, durable components with excellent surface finishes.
4.2 Aerospace Industry
In the aerospace industry, deep drawing is used to produce lightweight structural components, aircraft skin panels, and engine housings. The ability to create complex shapes with minimal material waste makes deep drawing ideal for the production of aerospace parts where weight reduction is critical.
4.3 Packaging Industry
One of the most common uses of deep drawing is in the packaging industry, particularly for manufacturing metal cans, containers, and caps for food, beverages, and cosmetics. The deep drawing process allows for the creation of seamless containers that are lightweight, airtight, and resistant to leaks.
4.4 Medical Devices
In the medical industry, deep drawing is used to produce surgical instruments, implants, and medical device housings. These parts require high precision, biocompatibility, and seamless construction, which makes deep drawing an ideal process for their production.
4.5 Consumer Goods
Deep drawing is also used in the production of various consumer goods such as kitchenware, appliances, and electronics. Items like stainless steel pots, pans, and electronic enclosures are often deep-drawn to provide strength, durability, and smooth surfaces.
5. Advantages of Deep Drawing
The deep drawing process offers several advantages that make it a preferred metal forming technique for many industries:
- High Production Efficiency: Deep drawing can produce large volumes of parts quickly, making it ideal for mass production.
- Cost-Effective for Complex Shapes: Once the tooling is set up, deep drawing is an economical process for producing complex shapes with minimal material waste.
- Seamless Parts: Deep drawing creates parts with seamless construction, eliminating the need for welding or joining, which improves the strength and appearance of the final product.
- Precision and Dimensional Accuracy: The process allows for the creation of parts with tight tolerances and excellent surface finishes, reducing the need for post-processing or finishing steps.
- Versatile Material Options: Deep drawing is compatible with a wide range of metals, offering flexibility in material selection based on the desired properties of the part.
Conclusion
Deep drawing is a versatile and efficient metal forming process used to create high-precision, seamless parts from flat metal sheets. By carefully controlling material flow, pressure, and tool design, manufacturers can produce complex, deep shapes with minimal defects and high material efficiency. Industries such as automotive, aerospace, medical, and consumer goods rely on deep drawing for the production of components that require strength, precision, and durability. The ability to produce lightweight, seamless parts with excellent dimensional accuracy makes deep drawing a valuable tool for modern manufacturing.
Working Principles of Deep Draw Sheet Hydroforming Presses
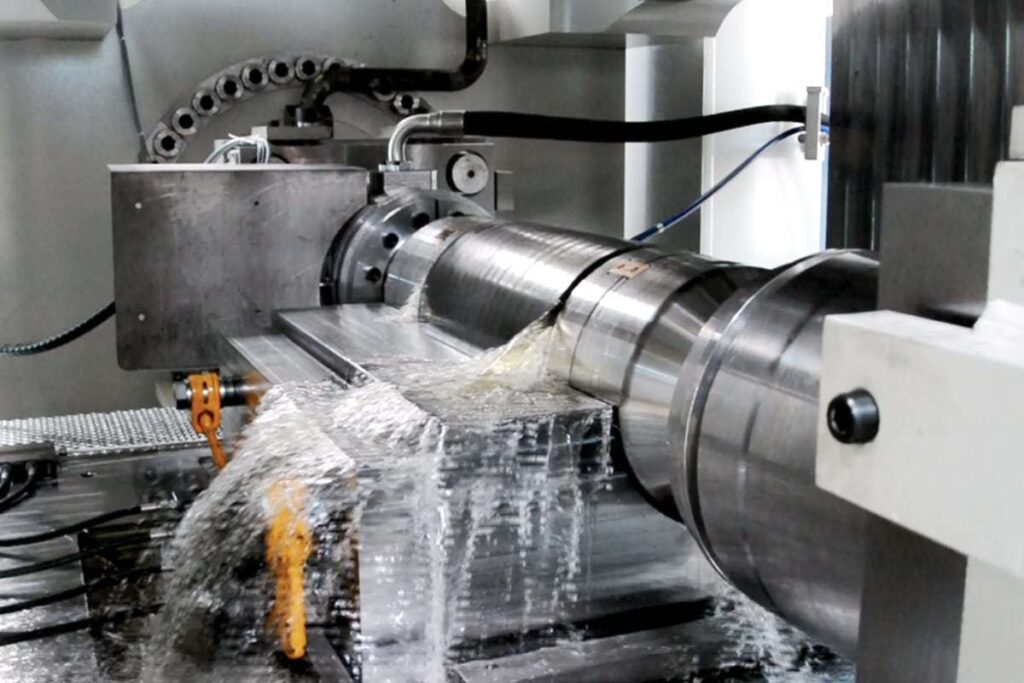
Deep draw sheet hydroforming presses are advanced machines used in metal forming processes to create complex, high-precision parts from flat sheet metal. The process combines deep drawing—where metal is drawn into a die to create parts with significant depth—with hydroforming, which uses hydraulic pressure to shape the material. Unlike traditional deep drawing, which relies on a punch and die, deep draw hydroforming uses hydraulic fluid and a flexible diaphragm to apply uniform pressure across the entire surface of the metal sheet, allowing for the creation of intricate geometries with fewer defects.
This article outlines the working principles of deep draw sheet hydroforming presses, explaining each step of the process, the role of hydraulic pressure, and how the machinery works to form deep-drawn parts.
1. Overview of Deep Draw Hydroforming
The deep draw sheet hydroforming process involves using a single-sided die (unlike traditional deep drawing, which requires both a male and female die) and hydraulic pressure to form a flat sheet of metal into a three-dimensional shape. The process allows for the creation of parts with greater depth than their diameter and is used in industries that require high-precision, lightweight, and seamless components, such as automotive, aerospace, and medical device manufacturing.
Key features of deep draw hydroforming include:
- Hydraulic Pressure: Applied uniformly across the metal surface, reducing defects such as thinning, tearing, and wrinkling.
- Flexible Diaphragm: A bladder or diaphragm filled with hydraulic fluid replaces the upper die, pressing the metal into the die cavity.
- Reduced Tooling: Since only a single-sided die is required, tooling costs and setup times are reduced compared to traditional stamping or deep drawing processes.
2. Key Components of Deep Draw Sheet Hydroforming Presses
Before exploring the step-by-step process, it is important to understand the main components of a deep draw sheet hydroforming press, which play a critical role in shaping the metal.
2.1 Hydraulic System
The hydraulic system is the core of the press, generating the necessary force to shape the sheet metal. The system consists of:
- Hydraulic Pump: Generates high-pressure fluid to apply force to the diaphragm and the sheet metal.
- Hydraulic Accumulator: Stores pressurized fluid and helps maintain consistent pressure throughout the forming process.
- Valves: Regulate the flow of hydraulic fluid, controlling pressure during different stages of the process.
2.2 Diaphragm (Flexible Bladder)
The diaphragm or bladder is a flexible membrane that holds hydraulic fluid. When pressure is applied, the diaphragm presses the metal sheet into the die. The flexibility of the diaphragm allows it to distribute pressure evenly across the metal, minimizing defects such as wrinkles or tears.
2.3 Die (Tooling)
The die is the mold into which the metal is formed. Unlike traditional deep drawing, which uses both a male and female die, deep draw hydroforming only requires a single-sided die, which reduces tooling complexity and cost. The die determines the final shape and geometry of the part.
2.4 Clamping and Sealing System
The clamping system holds the metal sheet in place and prevents it from shifting during the forming process. The clamping and sealing system ensures that the hydraulic pressure is applied uniformly and that the sheet remains securely positioned over the die.
2.5 Control System
Modern presses are equipped with computerized control systems such as CNC (Computer Numerical Control) and PLC (Programmable Logic Controller) systems, allowing for precise control of the forming process. These systems manage variables such as pressure levels, cycle times, and material deformation in real-time to ensure high-quality, repeatable results.
3. Step-by-Step Working Process of Deep Draw Sheet Hydroforming Presses
The deep draw hydroforming process follows a series of carefully controlled steps that transform a flat metal sheet into a three-dimensional, deep-drawn part. Below is a breakdown of the key stages involved:
3.1 Blank Preparation and Material Selection
The process starts with the preparation of the blank, a flat sheet of metal that will be formed into the desired shape. The material chosen for the blank depends on the application and the properties required for the final part. Common materials include aluminum, steel, stainless steel, titanium, and copper.
- Material Selection: The material must have good ductility and formability to ensure it can be stretched and drawn into the deep shape without tearing or breaking.
- Blank Size: The blank must be cut to the correct size and thickness, ensuring that there is enough material to be drawn into the die without excessive thinning or failure.
3.2 Die and Clamping Setup
Once the blank is ready, it is placed over the die inside the press. The die represents the shape of the final part, and it is positioned in the lower half of the press. The blank holder or clamping system is then engaged to hold the sheet in place.
- Die Positioning: The die must be precisely positioned in the press to ensure that the metal sheet is drawn evenly into the die cavity.
- Clamping: Proper clamping is essential to prevent the sheet from shifting during the forming process, which could lead to wrinkling, tearing, or uneven material flow.
3.3 Application of Hydraulic Pressure
The most critical stage of deep draw hydroforming is the application of hydraulic pressure. The hydraulic system pumps fluid into the flexible diaphragm, which presses against the metal sheet, forcing it into the die cavity.
- Gradual Pressure Increase: The hydraulic pressure is gradually increased to prevent sudden deformation, which could cause defects such as tearing or excessive thinning.
- Material Flow: As pressure builds, the sheet metal is drawn smoothly into the die, forming the desired deep shape. The flexibility of the diaphragm ensures that pressure is applied uniformly, allowing the metal to stretch evenly without localized stress points.
For complex or deep parts, the pressure may be applied in multiple stages, allowing the material to be progressively shaped without risking material failure.
3.4 Deep Drawing and Shaping
As the pressure continues to build, the deep drawing process takes place. The blank is pulled into the die cavity, forming a deep part with significant depth. The material undergoes plastic deformation, which means that it permanently takes on the shape of the die once formed.
- Deep Forming: The process allows for the creation of deep-drawn parts with high aspect ratios, meaning that the depth of the part can be much greater than its diameter.
- Material Thinning: While some thinning of the material occurs during deep drawing, the uniform application of pressure minimizes excessive thinning, ensuring that the part maintains structural integrity.
3.5 Pressure Holding and Stabilization
Once the part has taken its final shape, the hydraulic pressure is maintained for a short period to allow the material to stabilize. This is important for relieving any internal stresses in the material and ensuring that the part holds its final dimensions without springback.
- Pressure Holding: The system holds the pressure for a predetermined time to ensure that the material fully conforms to the die shape and achieves the required dimensional accuracy.
3.6 Pressure Release and Part Removal
After the holding phase, the hydraulic pressure is gradually released, and the diaphragm retracts, allowing the clamping system to disengage. The formed part is then removed from the die.
- Controlled Pressure Release: The gradual release of pressure ensures that the part does not spring back or deform after forming, maintaining its precise shape.
- Part Removal: Once the pressure is fully released, the part is gently removed from the die, and it may undergo further inspection or finishing operations such as trimming or polishing.
4. Advantages of Deep Draw Hydroforming
Deep draw hydroforming offers numerous advantages over traditional deep drawing methods. These advantages make it the preferred metal forming process for industries that require high-precision, lightweight, and complex parts.
4.1 Uniform Pressure Application
The use of hydraulic pressure ensures that the metal is formed with even force distribution, minimizing defects such as wrinkling, tearing, or excessive thinning. This results in parts with consistent wall thicknesses and smooth surface finishes.
- Reduced Defects: Wrinkling, buckling, and thinning are reduced due to the uniform pressure applied across the entire surface of the blank.
4.2 Ability to Form Complex Shapes
Deep draw hydroforming presses are ideal for creating complex, intricate shapes with deep draws and tight tolerances. The flexibility of the diaphragm allows for the formation of shapes that would be difficult or impossible to achieve with traditional deep drawing methods.
- Greater Design Freedom: Manufacturers have more flexibility in designing parts with sharp angles, deep recesses, and variable wall thicknesses.
4.3 Reduced Tooling Costs
Since deep draw hydroforming requires only a single-sided die, tooling costs are significantly lower compared to traditional deep drawing, which requires both male and female dies. This also reduces the time required for tool setup and die changes, increasing production efficiency.
- Lower Tooling Investment: The need for only one die reduces both initial tooling costs and ongoing maintenance costs.
4.4 Improved Surface Quality
The uniform pressure and absence of direct contact between the blank and a hard upper die result in parts with smooth surfaces and minimal imperfections. This reduces the need for secondary finishing operations like sanding or polishing.
- High-Quality Finish: Parts produced through hydroforming often require little to no additional finishing, which improves overall production efficiency.
4.5 Material Efficiency
Hydroforming minimizes material wastage by ensuring that the metal is formed with minimal thinning and no need for excess trimming or cutting. The process makes efficient use of the blank, reducing scrap rates and material costs.
5. Applications of Deep Draw Hydroforming
Deep draw sheet hydroforming presses are used in a wide range of industries that require high-performance parts with complex geometries. Some key applications include:
- Automotive Industry: For producing fuel tanks, engine covers, and chassis components that are lightweight yet strong.
- Aerospace Industry: For manufacturing aircraft panels, engine components, and landing gear parts that require precise dimensional accuracy and strength.
- Medical Devices: For creating surgical instruments, implants, and medical equipment housings with smooth surfaces and tight tolerances.
- Consumer Goods: For producing appliance housings, electronics enclosures, and kitchenware that require seamless construction and durability.
Conclusion
The working principles of deep draw sheet hydroforming presses involve using controlled hydraulic pressure to shape metal into complex, deep-drawn parts with high precision and minimal defects. By replacing traditional mechanical force with uniform hydraulic pressure, deep draw hydroforming offers several advantages, including reduced tooling costs, improved part quality, and greater design flexibility. This process is widely used in industries that require lightweight, durable, and complex components, making it an essential technology for modern manufacturing.
Key Components of Deep Draw Sheet Hydroforming Presses
A deep draw sheet hydroforming press is a specialized machine that uses hydraulic pressure to form sheet metal into complex, deep-drawn parts. These presses differ from conventional stamping or deep drawing machines by employing hydraulic fluid and a flexible diaphragm to shape the material, resulting in more uniform pressure application and the ability to create intricate shapes with fewer defects. Understanding the key components of a deep draw hydroforming press is essential for optimizing performance and achieving high-quality results.
This guide provides a comprehensive overview of the main components of deep draw hydroforming presses, explaining their functions and how they contribute to the efficiency and precision of the hydroforming process.
1. Hydraulic System
The hydraulic system is the central element of a deep draw hydroforming press, generating the high-pressure fluid required to form the metal sheet into the die. This system includes several critical subcomponents that work together to control the pressure and ensure the smooth operation of the press.
1.1 Hydraulic Pump
The hydraulic pump is responsible for generating the fluid pressure needed to shape the metal. The pump draws hydraulic fluid from a reservoir and pressurizes it before delivering it to the diaphragm or bladder.
- High-Pressure Capabilities: Deep draw hydroforming presses require pumps capable of generating very high pressures, typically ranging from 10,000 to 20,000 psi, depending on the material and the depth of the draw.
- Pump Types: Common pumps used in hydroforming systems include axial piston pumps and variable displacement pumps, which offer efficient, high-pressure operation with precise control over the flow rate.
1.2 Hydraulic Accumulator
The hydraulic accumulator stores pressurized fluid and releases it as needed to maintain consistent pressure during the forming process. It acts as an energy reservoir that ensures a steady supply of hydraulic pressure even when demand fluctuates.
- Pressure Stabilization: The accumulator helps smooth out pressure fluctuations and ensures that the pressure applied to the metal remains consistent throughout the drawing cycle, preventing defects caused by uneven pressure.
1.3 Hydraulic Valves
Hydraulic valves regulate the flow of hydraulic fluid throughout the system, directing it to various parts of the press and controlling the pressure as needed.
- Directional Control Valves: These valves determine the flow path of the hydraulic fluid, controlling whether the system is pressurizing the diaphragm, holding pressure, or releasing pressure after forming.
- Pressure Relief Valves: Safety devices that automatically release excess hydraulic fluid if the system pressure exceeds the safe operating limits, protecting both the press and the operator from potential hazards.
- Flow Control Valves: Used to adjust the rate at which hydraulic fluid is delivered to the diaphragm, allowing for precise control over the speed and pressure during the forming process.
1.4 Hydraulic Cylinders and Actuators
Hydraulic cylinders and actuators convert the pressurized fluid’s energy into mechanical motion. In deep draw hydroforming presses, these components are used to apply force to the diaphragm or blank holder.
- Hydraulic Cylinders: These are typically double-acting cylinders, which can apply pressure in both directions, ensuring precise control during the press’s drawing and retracting phases.
2. Flexible Diaphragm (Bladder)
The flexible diaphragm (also known as a bladder) is a crucial component in deep draw hydroforming presses, replacing the upper die used in traditional stamping. This diaphragm holds hydraulic fluid and transmits the pressure uniformly across the surface of the sheet metal.
2.1 Material and Construction
The diaphragm is typically made from durable, flexible materials such as rubber or urethane, which are capable of withstanding high pressure without tearing or losing flexibility.
- High-Pressure Resistance: The material must be able to withstand the extreme pressures exerted by the hydraulic fluid while maintaining flexibility to conform to the metal as it is drawn into the die.
- Long Durability: Diaphragms are designed for repeated cycles of high pressure without degrading, which is essential for maintaining consistent forming quality over long production runs.
2.2 Uniform Pressure Application
The diaphragm’s primary function is to ensure uniform pressure distribution over the entire surface of the metal sheet. By evenly distributing the hydraulic pressure, the diaphragm minimizes the risk of localized thinning, tearing, or wrinkling, which are common issues in conventional deep drawing processes.
- Smooth Material Flow: The flexible diaphragm ensures that the metal flows smoothly into the die, reducing stress concentrations and allowing for the creation of intricate shapes without defects.
3. Die (Tooling)
The die is the rigid tool used to shape the metal in the hydroforming press. In deep draw hydroforming, a single-sided die is used, unlike traditional deep drawing, which requires both a male and female die. The die defines the final geometry of the part and must be precisely machined to the desired shape.
3.1 Die Material
The die is typically made from hardened steel or aluminum, depending on the part’s complexity, the material being formed, and the production volume.
- Hardened Steel Dies: Used for high-volume production and forming high-strength materials, such as stainless steel or titanium. Steel dies are more durable and can withstand the repeated high pressures used in hydroforming.
- Aluminum Dies: Commonly used for lower production volumes or materials that are easier to form, such as aluminum sheets. Aluminum dies are lighter and less expensive than steel dies.
3.2 Die Design
The die must be custom-designed to match the shape of the final part. It is typically machined to tight tolerances to ensure precision and repeatability in the forming process.
- Complex Geometries: Deep draw hydroforming allows for the creation of parts with deep, intricate shapes that would be difficult or impossible to achieve using conventional forming methods.
- Single-Sided Tooling: Since only one die is required in hydroforming, tooling costs are reduced, and die setup is simplified.
3.3 Die Holder
The die holder is used to secure the die in place within the press, ensuring that it remains stationary and properly aligned during the forming process. Precise die alignment is critical for ensuring accurate part geometry and preventing defects.
4. Blank Holder (Binder) and Clamping System
The blank holder or clamping system secures the sheet metal blank in place over the die during the hydroforming process. This system is essential for ensuring that the blank does not shift or wrinkle as it is drawn into the die.
4.1 Blank Holder Function
The blank holder applies downward force on the outer edges of the blank, preventing excess material from wrinkling or buckling as it is drawn into the die. The force applied by the blank holder must be carefully controlled to ensure smooth material flow without causing tearing or excessive thinning.
- Controlled Pressure Application: The blank holder’s pressure must be sufficient to keep the blank in place, but not so high that it prevents the material from flowing into the die. This balance is crucial for avoiding defects.
4.2 Hydraulic or Mechanical Clamps
The blank holder is typically powered by hydraulic clamps, which offer precise control over the clamping force and allow for quick adjustments during production. In some cases, mechanical clamps may be used for simpler applications or smaller presses.
- Hydraulic Clamps: These provide uniform clamping pressure, reducing the risk of wrinkling or shifting. Hydraulic clamps are often preferred for high-precision applications and larger parts.
5. Control System
Modern deep draw hydroforming presses are equipped with advanced control systems that manage the various stages of the forming process. These control systems allow for precise management of variables such as pressure, cycle time, and material flow, ensuring consistent results and high-quality parts.
5.1 CNC (Computer Numerical Control)
CNC systems are commonly used to automate the press’s operation, controlling everything from pressure application to die movement. CNC systems offer high levels of precision and repeatability, making them ideal for complex, high-precision parts.
- Automated Pressure Control: The CNC system can control the rate of pressure increase, hold times, and pressure release, ensuring that each part is formed under optimal conditions.
- Multi-Axis Control: In some presses, the CNC system can control multiple axes, allowing for more complex part geometries and more efficient material flow.
5.2 PLC (Programmable Logic Controller)
A PLC system is often used to automate the operation of the press and ensure that each stage of the process is executed in the correct sequence. The PLC manages the press cycle, controlling the movement of the blank holder, the application of hydraulic pressure, and the part ejection.
- Process Monitoring: The PLC continuously monitors the press’s performance, adjusting parameters as needed to ensure the quality and consistency of each part.
- Safety Features: PLC systems often include safety interlocks and emergency stop functions to protect operators and equipment.
5.3 Sensors and Monitoring Systems
Modern deep draw hydroforming presses are equipped with a range of sensors that monitor key variables such as pressure, temperature, material flow, and die alignment. These sensors feed real-time data to the control system, allowing for continuous adjustments during the forming process.
- Pressure Sensors: Ensure that the correct hydraulic pressure is applied throughout the forming process.
- Temperature Sensors: In some applications, controlling the temperature of the hydraulic fluid or the material itself can help prevent defects and ensure consistent part quality.
6. Part Ejection System
Once the deep drawing process is complete, the formed part must be removed from the die. The ejection system lifts the part out of the die, preventing damage to the finished part during removal.
6.1 Hydraulic Ejectors
Many deep draw hydroforming presses use hydraulic ejectors to gently lift the part from the die after the pressure is released. The hydraulic system provides precise control, ensuring that the part is removed without damage or deformation.
- Controlled Ejection: The hydraulic ejectors operate in tandem with the press, allowing for smooth, controlled part removal.
6.2 Automated Ejection Systems
In fully automated production lines, robotic arms or automated ejection systems may be used to remove parts from the press and transfer them to the next stage of production, such as trimming or inspection.
7. Safety Systems
Due to the high pressures involved in deep draw hydroforming, safety systems are essential to protect operators and equipment.
7.1 Safety Guards and Enclosures
Most deep draw hydroforming presses are equipped with physical barriers, such as safety guards and enclosures, to prevent operators from coming into contact with the moving parts of the press during operation.
- Interlocks: These guards are often fitted with interlock systems that prevent the press from operating unless the guards are securely in place.
7.2 Emergency Stop Systems
Emergency stop buttons are strategically placed around the press, allowing operators to quickly halt the machine if an issue arises during operation.
7.3 Pressure Relief Systems
Pressure relief valves and other safety mechanisms ensure that the hydraulic system does not exceed safe operating pressures, protecting both the press and the operator from potential hazards.
Conclusion
The key components of deep draw sheet hydroforming presses work together to create a precise, efficient, and safe metal forming process. From the hydraulic system that generates the forming pressure to the flexible diaphragm that ensures uniform pressure distribution, each component plays a critical role in ensuring that complex, high-precision parts can be produced with minimal defects. As industries such as automotive, aerospace, and medical devices demand increasingly complex and lightweight components, deep draw hydroforming presses will continue to be an essential tool for modern manufacturing
Applications of Deep Draw Sheet Hydroforming Presses
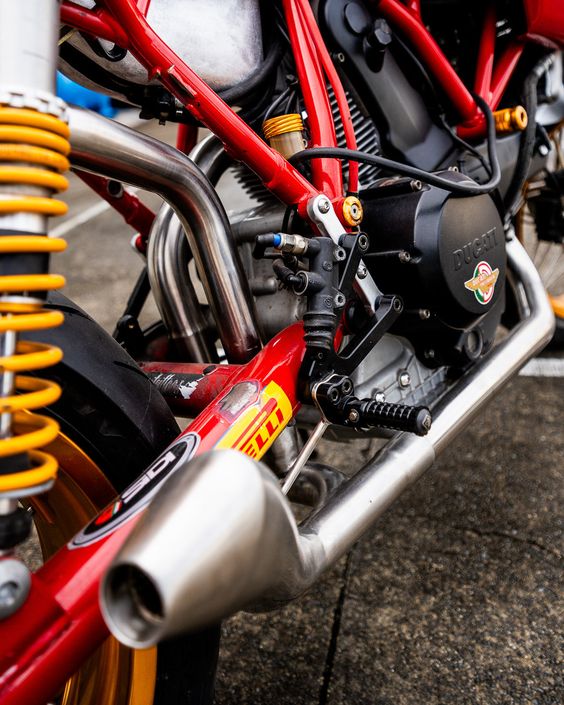
Deep draw sheet hydroforming presses are essential tools in modern manufacturing, offering a highly efficient and versatile process for forming sheet metal into complex, deep-drawn shapes. These presses are widely used across a variety of industries, including automotive, aerospace, medical devices, consumer electronics, and more. Deep draw hydroforming combines the benefits of traditional deep drawing and hydroforming techniques, using hydraulic pressure and a flexible diaphragm to form intricate, seamless parts with minimal defects.
The ability of deep draw hydroforming presses to produce lightweight, durable, and high-precision components with complex geometries makes them indispensable in industries that require tight tolerances, smooth finishes, and strong, lightweight structures.
This article explores the key applications of deep draw sheet hydroforming presses, focusing on how the technology is used across various industries, the specific benefits it offers in each sector, and examples of components commonly produced using this process.
1. Automotive Industry
The automotive industry is one of the largest users of deep draw hydroforming technology. As manufacturers seek to create lighter, stronger, and more efficient vehicles, deep draw hydroforming has become essential for producing components that require complex shapes and high strength-to-weight ratios. The ability to form lightweight structural components, engine parts, and exterior panels makes deep draw hydroforming particularly valuable in this sector.
1.1 Body Panels and Exterior Components
One of the primary applications of deep draw hydroforming in the automotive industry is the production of body panels and other exterior parts. Automotive body panels must be aerodynamic, lightweight, and aesthetically pleasing, with smooth surfaces and complex shapes that are difficult to achieve using traditional stamping methods.
- Car Doors and Hoods: Deep draw hydroforming is used to manufacture car doors, hoods, and trunk lids, which require a combination of strength and lightness. The smooth, seamless finish provided by hydroforming eliminates the need for additional polishing or surface treatments.
- Fenders and Bumpers: Bumpers and fenders are critical for vehicle safety and must be able to withstand impacts. Hydroformed fenders and bumpers can absorb shock and maintain structural integrity while reducing overall vehicle weight.
1.2 Chassis and Structural Components
The chassis is the structural backbone of a vehicle, and its components must provide strength without adding excess weight. Deep draw hydroforming is ideal for manufacturing lightweight structural components that enhance vehicle performance, safety, and fuel efficiency.
- Crossmembers and Subframes: Deep draw hydroforming is used to produce crossmembers, subframes, and other critical components that support the vehicle’s frame. These parts are designed to distribute the vehicle’s load and improve crashworthiness, particularly in the event of an impact.
- Suspension Components: Hydroforming is also used to create suspension components such as control arms, which require complex geometries to improve vehicle handling and ride quality.
1.3 Fuel and Fluid Systems
Deep draw hydroforming is used in the production of fuel tanks, fluid reservoirs, and exhaust systems. These components must be seamless, leak-proof, and capable of withstanding high pressures and temperatures.
- Fuel Tanks: Hydroforming allows for the production of lightweight, high-strength fuel tanks that are seamless, reducing the risk of leaks and improving durability.
- Exhaust Components: Exhaust manifolds and mufflers are hydroformed to create smooth internal surfaces that improve airflow and reduce back pressure, which enhances engine performance and reduces emissions.
1.4 Electric Vehicles (EVs) and Lightweighting
As the automotive industry shifts towards electric vehicles (EVs), the need for lightweight components becomes even more critical. The weight of EVs, largely due to their batteries, affects range and performance. Deep draw hydroforming helps reduce vehicle weight by enabling the production of lightweight structural and battery-related components.
- Battery Enclosures: Hydroforming is used to create lightweight, durable battery enclosures that protect EV batteries from impact while minimizing weight.
- Lightweight Frames and Reinforcements: By using hydroformed parts in the chassis and body, manufacturers can improve the range and efficiency of EVs without sacrificing strength.
2. Aerospace Industry
The aerospace industry requires lightweight, high-strength components that can withstand extreme conditions and provide maximum performance. Deep draw hydroforming presses are widely used to produce aircraft structural components, engine parts, and interior elements that meet stringent aerospace standards.
2.1 Fuselage and Structural Components
Deep draw hydroforming is frequently used to manufacture fuselage components, which form the structural backbone of aircraft. These parts must be lightweight and aerodynamically efficient while maintaining strength and durability.
- Aircraft Skin Panels: Hydroforming is used to create aircraft skin panels with smooth, seamless surfaces. These panels provide structural support and help maintain the aircraft’s aerodynamic shape.
- Bulkheads and Frames: Structural elements such as bulkheads and frames are hydroformed to ensure they are strong enough to withstand the stresses of flight while remaining lightweight.
2.2 Engine Components
Aircraft engines operate under extreme pressures and temperatures, requiring components that are both precise and heat-resistant. Deep draw hydroforming is ideal for producing complex, high-strength engine parts.
- Turbine Housings: Hydroforming is used to create turbine housings, which require high precision and durability to ensure the efficient operation of jet engines.
- Exhaust Components: The process is also used to produce exhaust components that can withstand high temperatures and harsh environments, such as nozzles, ducts, and cowling.
2.3 Lightweighting for Fuel Efficiency
The aerospace industry is always seeking ways to reduce aircraft weight in order to improve fuel efficiency and increase range. Deep draw hydroforming allows manufacturers to produce lightweight components that reduce overall aircraft weight while maintaining structural integrity.
- Wing Ribs and Spars: Hydroformed wing ribs and spars provide the necessary strength and stiffness while minimizing weight, improving the aircraft’s fuel efficiency and performance.
3. Medical Devices
In the medical field, precision, surface quality, and material properties are critical for ensuring patient safety and device reliability. Deep draw hydroforming is widely used to produce high-quality surgical instruments, medical implants, and diagnostic equipment components.
3.1 Surgical Instruments
Deep draw hydroforming is used to create surgical instruments that require smooth surfaces, precise dimensions, and high durability. The seamless construction provided by hydroforming helps ensure that the instruments are easy to clean and sterilize, reducing the risk of contamination.
- Forceps, Scalpels, and Retractors: Hydroforming is used to manufacture forceps, scalpels, and retractors, where smooth, seamless construction is essential for precision and hygiene in surgical procedures.
3.2 Medical Implants
Medical implants such as orthopedic devices, joint replacements, and spinal components must meet stringent biocompatibility and strength requirements. Deep draw hydroforming allows for the creation of customized shapes with high precision and smooth finishes.
- Joint Replacements: Hydroformed parts used in hip and knee replacements ensure a custom fit for each patient, improving the functionality and longevity of the implant.
- Spinal Implants: The process is used to create spinal implants that require intricate shapes and high strength-to-weight ratios to support and stabilize the spine.
3.3 Diagnostic Equipment
In addition to surgical instruments and implants, deep draw hydroforming is used to produce components for diagnostic equipment, such as MRI machines and X-ray devices. These parts require dimensional accuracy and smooth surfaces to ensure optimal performance.
- Casing and Housings: Hydroformed casings and housings for diagnostic equipment are designed to be lightweight, durable, and resistant to damage, ensuring the longevity of the equipment.
4. Consumer Electronics and Appliances
Deep draw hydroforming presses are widely used in the production of consumer electronics and appliances due to their ability to create lightweight, aesthetically pleasing, and durable components. These components often feature intricate designs and smooth finishes, making hydroforming an ideal manufacturing method.
4.1 Consumer Electronics
The electronics industry relies on deep draw hydroforming to produce housings, frames, and structural components for devices like smartphones, tablets, and laptops. These products require lightweight, precision-formed parts with sleek designs that can be mass-produced with minimal defects.
- Smartphone Housings: Hydroformed smartphone casings provide a premium look and feel while ensuring durability and resistance to impact.
- Laptop Chassis: The process is also used to create laptop frames and chassis, where lightweight construction and high strength are essential for mobility and product longevity.
4.2 Appliances
In the appliance industry, deep draw hydroforming is used to produce components for kitchen appliances, laundry machines, and other consumer goods that require both durability and aesthetic appeal.
- Refrigerator Doors and Panels: Hydroformed refrigerator panels and doors offer seamless, corrosion-resistant surfaces that are easy to clean and maintain.
- Oven Liners and Control Panels: Deep draw hydroforming is used to create oven liners and control panels that can withstand high temperatures while maintaining a polished, modern appearance.
5. Energy and Power Generation
Deep draw hydroforming presses play an important role in the energy and power generation sectors, where they are used to produce components for wind turbines, solar panels, and oil and gas equipment. The ability to form lightweight, durable parts with high precision makes hydroforming ideal for the demanding environments of these industries.
5.1 Wind Turbine Components
In the wind energy industry, deep draw hydroforming is used to manufacture structural components for wind turbines, including the hub, nacelle, and blade root fittings. These parts must be able to withstand the high stresses and harsh environmental conditions associated with wind energy production.
- Nacelle Housings: Hydroforming is used to create lightweight nacelle housings that protect the sensitive electrical components of wind turbines from the elements.
5.2 Solar Energy Systems
In solar energy systems, hydroforming is used to create mounting brackets and frames for solar panels. These components must be lightweight, durable, and capable of withstanding harsh weather conditions over extended periods.
- Solar Panel Frames: Hydroformed frames provide the necessary support for solar panels while minimizing weight, reducing installation costs, and improving efficiency.
6. Sporting Goods and Bicycles
In the world of sporting goods and bicycles, weight, performance, and durability are critical factors in product design. Deep draw hydroforming presses are used to manufacture components that improve the aerodynamics, strength, and performance of sports equipment and bicycles.
6.1 Bicycle Frames
Hydroforming is widely used to create bicycle frames, especially for high-performance road bikes, mountain bikes, and electric bicycles (e-bikes). The process allows manufacturers to produce lightweight, strong frames with tapered tubes and complex shapes that enhance the bike’s performance.
- Road Bikes and Mountain Bikes: Hydroformed aluminum and titanium frames are used in high-end bicycles, offering a combination of strength, durability, and reduced weight for improved handling and speed.
- Electric Bicycles: As the demand for electric bicycles (e-bikes) grows, hydroforming is used to create integrated battery housings and lightweight frames that improve battery efficiency and extend the bike’s range.
6.2 Sporting Goods
In addition to bicycles, deep draw hydroforming is used in the production of sporting goods such as golf clubs, hockey sticks, and tennis rackets, where lightweight materials and high strength are essential for improving performance.
- Golf Club Shafts: Hydroformed golf club shafts are engineered to provide optimal weight distribution and stiffness, improving the golfer’s swing and control.
- Tennis Rackets: The process is also used to create tennis racket frames with precise weight and balance, enhancing the player’s performance.
Conclusion
Deep draw sheet hydroforming presses are versatile and powerful tools for manufacturing high-precision, lightweight components across a wide range of industries. From automotive body panels and aerospace engine parts to medical implants and consumer electronics, deep draw hydroforming offers manufacturers the ability to produce complex, durable, and seamless parts with minimal material waste. As industries continue to demand lighter, stronger, and more efficient components, deep draw hydroforming will remain an essential technology for achieving these goals and driving innovation in modern manufacturing.
Deep Draw Sheet Hydroforming Press: Overview, Process, and Applications
A deep draw sheet hydroforming press is a specialized machine used to form complex, high-precision parts from sheet metal through a process that combines deep drawing and hydroforming. Unlike traditional deep drawing, which relies on a punch and die to shape metal, deep draw hydroforming uses hydraulic fluid pressure to force the sheet metal into the shape of the die. This method allows for the creation of intricate geometries, deep-drawn parts, and components with minimal material defects such as wrinkling, tearing, or thinning.
Deep draw hydroforming presses are used extensively in industries that demand lightweight, high-strength components, including automotive, aerospace, medical devices, and consumer goods. The process offers several advantages over traditional methods, including lower tooling costs, improved part quality, and the ability to form complex shapes that are difficult or impossible to achieve with conventional drawing techniques.
1. Overview of Deep Draw Hydroforming
Deep drawing is a metal forming process where a flat sheet of metal (called a blank) is drawn into a die to form a deep, hollow shape. In traditional deep drawing, a mechanical punch pushes the blank into a die, often leading to defects such as tearing, wrinkling, or excessive thinning, particularly with complex shapes or deep draws.
Hydroforming, on the other hand, uses a high-pressure hydraulic fluid to shape the metal. In deep draw hydroforming, this fluid is applied through a flexible diaphragm (bladder), which presses the sheet metal against a die to create the desired shape. This process applies uniform pressure over the entire surface of the metal, resulting in more even material flow and fewer defects.
The combination of deep drawing and hydroforming allows manufacturers to produce deep, intricate parts with precise dimensions, smooth surfaces, and high strength.
2. Key Components of a Deep Draw Sheet Hydroforming Press
To better understand the deep draw hydroforming process, it’s important to explore the components that make up the hydroforming press:
2.1 Hydraulic System
The hydraulic system is the core of the hydroforming press, generating the high-pressure fluid needed to form the metal. It includes:
- Hydraulic Pumps: These generate the high-pressure fluid required for the process, with pressures often exceeding 10,000 psi for complex parts.
- Accumulators: These store pressurized fluid, ensuring consistent pressure throughout the process.
- Valves: These regulate the flow of hydraulic fluid, ensuring controlled pressure at each stage of the process.
2.2 Flexible Diaphragm (Bladder)
The diaphragm or bladder holds the hydraulic fluid and presses it against the metal blank to apply uniform pressure. The diaphragm is typically made from flexible, durable materials such as urethane or rubber, which can withstand the high pressures involved in the process while allowing for even pressure distribution over the metal surface.
2.3 Die (Tooling)
The die defines the shape of the final part and is made from durable materials like hardened steel or aluminum. In deep draw hydroforming, only a single-sided die is needed, reducing tooling costs compared to traditional methods that require both a punch and die.
2.4 Clamping and Sealing System
The clamping system secures the sheet metal blank in place during the forming process, preventing it from shifting or wrinkling. A tight sealing system is also essential to ensure that hydraulic pressure is properly applied without any leaks.
2.5 Control System
Modern presses feature CNC (Computer Numerical Control) or PLC (Programmable Logic Controller) systems that manage the pressure application, cycle times, and other critical parameters. These control systems allow for precise adjustments and real-time monitoring to ensure that parts are formed accurately and consistently.
3. Deep Draw Hydroforming Process
The deep draw hydroforming process involves several steps that transform a flat metal sheet into a deep, intricately shaped part. Here is a step-by-step overview of the process:
3.1 Blank Preparation
The process begins with the preparation of the blank, a flat sheet of metal cut to the appropriate size for the part being formed. The blank material is selected based on the desired characteristics of the final part, with common materials including aluminum, steel, stainless steel, titanium, and copper.
3.2 Die Setup and Clamping
The prepared blank is placed over the die in the hydroforming press. The die defines the final shape of the part, and the blank is clamped securely to prevent movement during forming.
- Clamping: Proper clamping ensures that the blank remains stationary while pressure is applied, preventing issues such as wrinkling or misalignment.
3.3 Application of Hydraulic Pressure
Once the blank is secured, hydraulic fluid is pumped into the diaphragm, which then applies uniform pressure across the entire surface of the blank. This pressure forces the blank to conform to the shape of the die, stretching and forming it into the desired deep shape.
- Gradual Pressure Increase: Hydraulic pressure is applied gradually to avoid sudden deformation, which could cause the metal to tear or thin excessively.
- Even Material Flow: The flexible diaphragm ensures that the pressure is distributed evenly, allowing the material to flow smoothly into the die without localized thinning or buckling.
3.4 Deep Drawing and Shaping
As the pressure builds, the blank is drawn into the die, taking on the final shape. The deep drawing process can produce parts with complex geometries, deep draws, and variable wall thicknesses, depending on the design requirements.
- Forming Complex Parts: Deep draw hydroforming is particularly suited for creating parts with deep recesses, intricate curves, and sharp angles that are difficult to achieve using conventional methods.
3.5 Pressure Holding and Release
Once the part has been fully formed, the hydraulic pressure is maintained for a brief period to allow the material to stabilize and take on its final shape.
- Stabilization: This holding phase helps to relieve internal stresses and ensures dimensional accuracy.
- Controlled Pressure Release: After the pressure is held, it is gradually released to prevent any springback or deformation of the part.
3.6 Part Removal and Finishing
Once the pressure is released, the part is removed from the die. Depending on the application, additional finishing steps such as trimming, deburring, or surface polishing may be required.
4. Applications of Deep Draw Hydroforming Presses
Deep draw hydroforming presses are used in a wide variety of industries due to their ability to produce complex, high-precision components with minimal defects. Here are some of the most common applications:
4.1 Automotive Industry
In the automotive industry, deep draw hydroforming is used to produce lightweight, high-strength components that improve vehicle performance and fuel efficiency.
- Body Panels: Hydroforming is used to create car doors, hoods, trunk lids, and other body panels with smooth surfaces and aerodynamic shapes.
- Chassis Components: Structural parts like crossmembers, subframes, and reinforcements are hydroformed to provide strength while reducing vehicle weight.
- Exhaust Systems and Fuel Tanks: Hydroformed exhaust manifolds, fuel tanks, and mufflers feature seamless, leak-resistant construction that enhances durability and performance.
4.2 Aerospace Industry
The aerospace industry benefits from the ability of deep draw hydroforming to produce lightweight, high-strength components that can withstand the rigors of flight.
- Fuselage Panels: Hydroforming is used to create aircraft skin panels that are lightweight and aerodynamically efficient.
- Engine Components: Complex parts like turbine housings, exhaust ducts, and turbine blades are formed using hydroforming to achieve tight tolerances and high strength.
4.3 Medical Devices
In the medical field, deep draw hydroforming is used to manufacture biocompatible, precision components that are critical for medical devices.
- Surgical Instruments: Instruments such as forceps, scalpels, and retractors are hydroformed to provide seamless, easy-to-sterilize surfaces.
- Implants: Medical implants, such as joint replacements and spinal implants, are hydroformed to meet stringent safety and performance standards.
4.4 Consumer Goods and Appliances
In the consumer goods and appliance industries, deep draw hydroforming is used to create durable, aesthetically pleasing components for a wide range of products.
- Kitchen Appliances: Hydroformed parts such as oven liners, refrigerator doors, and control panels offer durability, smooth finishes, and corrosion resistance.
- Consumer Electronics: Hydroformed smartphone housings, laptop frames, and other electronic enclosures combine lightweight construction with sleek designs.
5. Advantages of Deep Draw Hydroforming
Deep draw hydroforming offers several advantages over traditional deep drawing and other metal forming methods:
5.1 Reduced Tooling Costs
Since deep draw hydroforming uses only a single-sided die, tooling costs are significantly lower compared to traditional methods that require both a punch and die. This also leads to shorter setup times and faster production cycles.
5.2 Improved Part Quality
The uniform pressure applied during hydroforming results in better material flow and fewer defects, such as wrinkling, tearing, or thinning. The process produces parts with smooth surfaces and high dimensional accuracy, reducing the need for post-processing.
5.3 Greater Design Flexibility
Deep draw hydroforming allows for the production of complex geometries that are difficult to achieve with conventional deep drawing. This includes parts with deep draws, sharp angles, and variable wall thicknesses.
5.4 Material Efficiency
The hydroforming process minimizes material wastage, as the blank is formed directly into the desired shape with minimal trimming or cutting. This makes it a more sustainable and cost-effective option for manufacturers.
Conclusion
The deep draw sheet hydroforming press is a versatile and powerful tool that allows manufacturers to produce complex, high-precision parts with minimal defects. By using hydraulic pressure and a flexible diaphragm to shape metal, deep draw hydroforming offers several advantages over traditional methods, including reduced tooling costs, improved part quality, and greater design flexibility. These benefits make it a valuable technology in industries such as automotive, aerospace, medical devices, and consumer electronics, where lightweight, high-performance components are essential.
Şunu dedin:
Overview of Deep Draw Hydroforming (2000 words)
ChatGPT:
Overview of Deep Draw Hydroforming (2000 words)
Deep draw hydroforming is an advanced metal forming process used to create intricate, high-precision parts from sheet metal. By combining traditional deep drawing with the principles of hydroforming, deep draw hydroforming offers a versatile and efficient method for manufacturing parts with complex geometries, deep draws, and seamless construction. It is a widely used technique in industries such as automotive, aerospace, medical devices, consumer goods, and more.
In deep draw hydroforming, hydraulic pressure is used to shape the sheet metal into the desired form by pressing it against a die, offering numerous advantages over traditional deep drawing methods, including improved material flow, reduced defects, lower tooling costs, and the ability to form deep, intricate shapes.
This comprehensive overview of deep draw hydroforming will explore its fundamental principles, the equipment used in the process, the step-by-step process of forming, its applications, and the advantages and limitations associated with this technology.
1. What Is Deep Draw Hydroforming?
Deep draw hydroforming is a sheet metal forming process that involves using hydraulic pressure to shape metal into complex, deep-drawn parts. The process is an enhancement of traditional deep drawing, where a punch is used to mechanically push a sheet metal blank into a die, creating parts such as cups, cans, or tanks. In deep draw hydroforming, a flexible diaphragm (bladder) and hydraulic fluid replace the upper punch, applying uniform pressure over the surface of the blank. This ensures even material flow and reduces the likelihood of defects such as wrinkling, tearing, or thinning.
The term “deep draw” refers to the fact that the depth of the part formed is often greater than its diameter. The combination of hydraulic pressure and deep drawing allows for the creation of deep, hollow parts with complex shapes that would be difficult or impossible to achieve using traditional stamping or mechanical deep drawing methods.
2. The Basic Principles of Deep Draw Hydroforming
2.1 Hydraulic Pressure
The defining feature of deep draw hydroforming is the use of hydraulic pressure to form the metal. Instead of using a mechanical punch to force the metal into a die, as in traditional deep drawing, hydroforming uses a flexible diaphragm filled with hydraulic fluid. The pressurization of this fluid allows the diaphragm to apply even, controlled pressure over the entire surface of the metal sheet. This uniform pressure distribution ensures smoother material flow, minimizes localized stress points, and reduces the likelihood of defects such as thinning or tearing.
2.2 Flexible Diaphragm (Bladder)
A key component of the deep draw hydroforming press is the diaphragm or bladder. This is a flexible membrane made from durable materials such as rubber or urethane, which can withstand high hydraulic pressures. During the forming process, hydraulic fluid is pumped into the bladder, causing it to expand and press against the sheet metal. This forces the metal to conform to the shape of the die beneath it.
The use of a diaphragm allows the metal to be shaped without the need for a matching punch and die set, as required in traditional deep drawing, resulting in reduced tooling costs and greater design flexibility.
2.3 Single-Sided Tooling
One of the primary advantages of deep draw hydroforming is that it requires only single-sided tooling. Unlike conventional deep drawing, which requires both a male and female die to shape the part, deep draw hydroforming uses only a single, rigid die. The diaphragm replaces the upper die by applying hydraulic pressure, which forces the metal blank into the die to create the desired shape. This significantly reduces tooling costs and setup times.
2.4 Material Flow and Formability
The use of hydraulic pressure ensures that the material flows evenly into the die during the forming process. This results in a more controlled and predictable material deformation, allowing for deep draws and the formation of complex geometries. Deep draw hydroforming is particularly well-suited for materials that require precise forming, such as aluminum, steel, stainless steel, titanium, and copper.
3. Deep Draw Hydroforming Equipment and Components
To carry out deep draw hydroforming, specialized equipment is required. The deep draw hydroforming press consists of several key components:
3.1 Hydraulic Press
The hydraulic press is the heart of the hydroforming system. It generates the hydraulic pressure necessary to shape the sheet metal into the die. Presses are typically equipped with hydraulic pumps capable of generating pressures up to 10,000 to 20,000 psi or more, depending on the material and part being formed.
- Hydraulic Pumps: High-pressure pumps provide the necessary fluid power to pressurize the diaphragm and shape the metal.
- Accumulators: Hydraulic accumulators store pressurized fluid and release it when needed to ensure consistent pressure during the forming process.
- Valves: These regulate fluid flow and pressure, ensuring the appropriate amount of force is applied to the blank during forming.
3.2 Flexible Diaphragm
As mentioned, the flexible diaphragm is a crucial component of the hydroforming process. This membrane holds the hydraulic fluid and presses against the metal blank. The diaphragm must be durable enough to withstand high pressures and repeated cycles without failure.
- Material: Diaphragms are typically made from rubber or urethane to ensure flexibility and durability under high-pressure conditions.
- Uniform Pressure Distribution: The diaphragm’s flexibility ensures that pressure is applied evenly across the blank, resulting in consistent material deformation.
3.3 Die (Tooling)
The die used in deep draw hydroforming is a rigid mold that defines the shape of the final part. In hydroforming, only a single-sided die is required, as the diaphragm provides the forming pressure.
- Material: Dies are commonly made from hardened steel or aluminum, depending on the production volume and the material being formed.
- Custom Design: Each die is custom-designed to match the geometry of the desired part, and it must be machined with precision to ensure repeatable results.
3.4 Clamping System
The clamping system holds the sheet metal blank securely in place during the forming process. Proper clamping is essential to prevent the blank from shifting, wrinkling, or misaligning during forming.
- Hydraulic Clamps: In high-precision applications, hydraulic clamps are often used to provide uniform clamping pressure.
- Blank Holders: Blank holders are used to control the flow of the metal as it is drawn into the die, preventing wrinkles or other defects.
3.5 Control System
Modern deep draw hydroforming presses are equipped with CNC (Computer Numerical Control) or PLC (Programmable Logic Controller) systems that control the forming process. These systems allow for precise control over pressure application, cycle time, and material flow, ensuring consistency and repeatability in the parts produced.
4. The Deep Draw Hydroforming Process: Step-by-Step
The deep draw hydroforming process follows a series of carefully controlled steps to ensure accurate, high-quality parts. Here is an overview of the typical steps involved:
4.1 Blank Preparation
The process begins with the preparation of the metal blank, which is typically cut from a sheet of material. The blank is sized to the dimensions of the desired part, ensuring there is enough material to be drawn into the die without excessive thinning or tearing.
4.2 Die Setup and Clamping
The prepared blank is placed over the die, which is positioned in the lower half of the hydroforming press. The blank is then clamped in place using a clamping system or blank holder, ensuring that the sheet remains stationary during forming.
4.3 Application of Hydraulic Pressure
Hydraulic fluid is pumped into the diaphragm, creating high pressure that is applied evenly across the blank. The pressure forces the metal into the die, stretching and deforming it into the desired shape.
- Gradual Pressure Increase: Pressure is increased gradually to prevent sudden deformation, which could lead to defects such as tearing or wrinkling.
- Uniform Pressure Application: The diaphragm ensures that pressure is applied uniformly, allowing the material to flow smoothly into the die and reducing the likelihood of localized thinning or stress points.
4.4 Deep Drawing and Shaping
As the hydraulic pressure continues to build, the blank is drawn deeper into the die, forming the desired part. The flexibility of the diaphragm allows the metal to be shaped into deep, intricate geometries, including sharp angles, deep recesses, and curves.
- Forming Complex Shapes: Deep draw hydroforming is particularly well-suited for creating parts with complex geometries that would be difficult or impossible to achieve using conventional drawing methods.
4.5 Pressure Holding and Release
Once the part has taken on its final shape, the hydraulic pressure is maintained briefly to allow the material to stabilize. After this, the pressure is gradually released, and the diaphragm retracts.
- Stabilization: Holding the pressure allows internal stresses in the material to dissipate, ensuring that the part retains its shape and dimensions.
- Controlled Pressure Release: Gradual release of pressure prevents springback or deformation of the part.
4.6 Part Removal and Finishing
After the pressure has been fully released, the formed part is removed from the die. Depending on the part, additional finishing steps such as trimming, deburring, or polishing may be required.
5. Applications of Deep Draw Hydroforming
Deep draw hydroforming is used across a variety of industries to produce high-precision, lightweight components with complex geometries. Below are some common applications:
5.1 Automotive Industry
In the automotive industry, deep draw hydroforming is used to create body panels, chassis components, fuel tanks, and exhaust systems. These parts require a combination of strength, lightweight construction, and smooth surfaces, making hydroforming an ideal solution.
- Lightweighting for Fuel Efficiency: The ability to produce strong yet lightweight components is critical for improving fuel efficiency in modern vehicles.
- Crash-Resistant Structures: Hydroformed structural components provide improved crash performance, as they can absorb energy more effectively during an impact.
5.2 Aerospace Industry
In the aerospace industry, weight reduction is essential for improving fuel efficiency and increasing payload capacity. Deep draw hydroforming is used to produce aircraft skin panels, engine components, and structural parts that are both lightweight and durable.
- Fuselage and Wing Components: Hydroformed parts such as wing ribs and bulkheads help reduce overall aircraft weight while maintaining structural integrity.
5.3 Medical Devices
In the medical field, deep draw hydroforming is used to manufacture surgical instruments, implants, and medical device housings that require high precision and biocompatibility.
- Seamless, Sterilizable Instruments: Hydroformed instruments feature smooth, seamless surfaces that are easy to sterilize, reducing the risk of contamination during surgery.
5.4 Consumer Goods
In the consumer goods sector, deep draw hydroforming is used to create durable, lightweight, and aesthetically pleasing parts for products such as appliances and electronics.
- Smartphone Housings and Appliance Panels: Hydroformed components provide sleek, durable designs for modern consumer electronics and home appliances.
6. Advantages and Limitations of Deep Draw Hydroforming
6.1 Advantages
- Uniform Pressure Application: The use of hydraulic pressure ensures even material flow and reduces defects such as wrinkling, thinning, and tearing.
- Reduced Tooling Costs: Only a single-sided die is required, reducing tooling costs and setup times.
- Complex Geometries: Deep draw hydroforming allows for the creation of complex, deep-drawn parts with intricate shapes and tight tolerances.
- Material Efficiency: The process minimizes material waste, as the blank is formed directly into the desired shape.
6.2 Limitations
- Cycle Time: Deep draw hydroforming can have longer cycle times compared to traditional stamping or deep drawing, especially for complex parts.
- Initial Investment: The cost of a hydroforming press can be higher than that of traditional presses, though lower tooling costs often offset this in the long term.
- Material Limitations: Not all metals are suitable for deep draw hydroforming, as some materials may not have the required formability for deep drawing.
Conclusion
Deep draw hydroforming is a highly efficient and versatile process that allows manufacturers to create complex, high-precision parts with minimal defects. By using hydraulic pressure to shape sheet metal into intricate geometries, deep draw hydroforming offers significant advantages over traditional deep drawing methods, including lower tooling costs, better material utilization, and the ability to form deep, complex shapes. This makes the process ideal for industries such as automotive, aerospace, medical devices, and consumer goods, where lightweight, high-performance components are essential. As technology continues to advance, deep draw hydroforming is expected to play an increasingly important role in the future of modern manufacturing.
Key Components of a Deep Draw Sheet Hydroforming Press
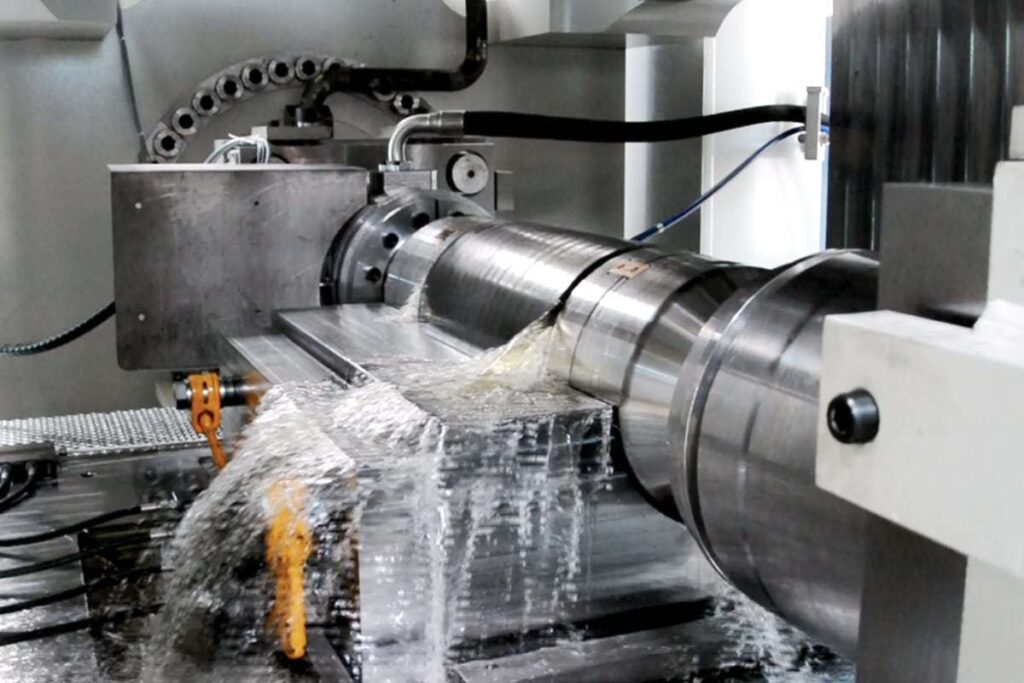
A deep draw sheet hydroforming press is an advanced machine designed to form sheet metal into complex, deep-drawn parts through the use of hydraulic pressure. The process allows manufacturers to create intricate shapes with high precision and minimal defects, making it a valuable tool for industries such as automotive, aerospace, medical devices, and consumer electronics. Understanding the key components of a deep draw hydroforming press is essential for optimizing its performance, ensuring part quality, and maintaining efficient production cycles.
This guide explores the key components of a deep draw sheet hydroforming press, outlining the function of each part and how they contribute to the overall hydroforming process.
1. Hydraulic System
The hydraulic system is the core of any deep draw hydroforming press, generating the high-pressure fluid necessary to form the sheet metal into the desired shape. This system includes multiple subcomponents that work together to control and regulate the flow and pressure of the hydraulic fluid throughout the forming process.
1.1 Hydraulic Pump
The hydraulic pump is responsible for generating the pressurized fluid that powers the diaphragm in the hydroforming press. The pump draws hydraulic fluid from a reservoir, pressurizes it, and delivers it into the diaphragm or bladder to apply force on the sheet metal.
- High-Pressure Output: Deep draw hydroforming presses typically require very high pressures, often exceeding 10,000 psi (pounds per square inch), to deform the metal into the desired shape without causing defects.
- Pump Types: There are several types of hydraulic pumps used in hydroforming systems, such as axial piston pumps and variable displacement pumps. These pumps are designed to provide precise, high-pressure fluid control, which is critical for achieving accurate part formation.
1.2 Hydraulic Accumulator
The hydraulic accumulator stores pressurized fluid and releases it when needed to maintain consistent pressure throughout the forming process. Accumulators act as energy reservoirs that help smooth out pressure fluctuations and ensure that the hydraulic system maintains steady pressure during the entire hydroforming cycle.
- Pressure Stabilization: Accumulators play a key role in stabilizing pressure, ensuring that the sheet metal is formed uniformly, even when the hydraulic demand varies during different stages of the process.
- Types of Accumulators: Common accumulator types used in hydroforming include bladder accumulators, which store pressurized fluid and release it on demand to keep the system running smoothly.
1.3 Hydraulic Valves
Hydraulic valves control the flow of hydraulic fluid within the system, regulating the pressure and directing the fluid to various parts of the press as needed. Several different types of valves are used in a hydroforming press:
- Directional Control Valves: These valves control the flow direction of the hydraulic fluid, determining whether the press is building pressure, holding pressure, or releasing pressure.
- Pressure Relief Valves: These safety valves release excess fluid if the pressure exceeds the system’s safe limits, protecting the press and operator from overpressure conditions.
- Flow Control Valves: These regulate the flow rate of hydraulic fluid into the diaphragm, ensuring that the pressure is applied gradually and evenly during the forming process.
1.4 Hydraulic Actuators and Cylinders
Hydraulic actuators convert the hydraulic fluid’s pressure into mechanical motion. In a deep draw hydroforming press, hydraulic cylinders are used to apply force to the flexible diaphragm or bladder, compressing the fluid and driving the forming process.
- Single-Acting Cylinders: These are designed to apply pressure in one direction, commonly used for applications where precise control of the diaphragm’s movement is essential.
- Double-Acting Cylinders: These can apply pressure in both directions, offering better control over the press’s motion and enabling greater precision in forming deep, intricate shapes.
2. Flexible Diaphragm (Bladder)
The flexible diaphragm, also known as the bladder, is a critical component in a deep draw hydroforming press. It replaces the upper punch typically used in traditional stamping or deep drawing processes. The diaphragm holds hydraulic fluid, and when pressurized, it applies uniform pressure across the entire surface of the metal blank, pressing it into the die to form the desired shape.
2.1 Diaphragm Material and Durability
The diaphragm must be made from a material that is both flexible and durable enough to withstand the repeated high-pressure cycles of the hydroforming process. Common materials include rubber, urethane, and composite materials.
- High-Pressure Resistance: The diaphragm must be capable of withstanding pressures upwards of 10,000 psi without bursting or losing its flexibility. It must also be durable enough to handle multiple forming cycles without wearing out.
- Elasticity and Flexibility: The material used for the diaphragm must maintain its elasticity to ensure that it conforms to the shape of the die and applies even pressure across the metal blank.
2.2 Function of the Diaphragm
The primary function of the diaphragm is to distribute pressure uniformly across the surface of the sheet metal. This ensures that the material flows evenly into the die, minimizing defects such as thinning, wrinkling, or tearing.
- Even Pressure Distribution: The flexibility of the diaphragm allows it to apply pressure uniformly, ensuring that the metal deforms consistently without localized stress points.
- Complex Shapes: The diaphragm’s ability to conform to intricate die shapes allows for the forming of complex, deep-drawn parts that would be difficult to produce using traditional deep drawing methods.
3. Die (Tooling)
The die is the mold used to shape the metal sheet in a deep draw hydroforming press. In traditional deep drawing, both a male and female die are used to shape the part. However, in hydroforming, only a single-sided die is required, as the flexible diaphragm provides the forming force.
3.1 Die Material
Dies for deep draw hydroforming are typically made from materials like hardened steel, cast iron, or aluminum, depending on the application, part complexity, and production volume.
- Hardened Steel Dies: Used for high-volume production and for forming high-strength materials like stainless steel or titanium. Steel dies are durable and capable of withstanding repeated high-pressure cycles without deforming.
- Aluminum Dies: Commonly used for forming softer metals like aluminum or for lower production volumes. Aluminum dies are lighter and easier to machine, though less durable than steel dies.
3.2 Die Design and Complexity
The design of the die is critical to the success of the hydroforming process. Dies are custom-machined to match the geometry of the part being formed, and they must be precisely engineered to ensure dimensional accuracy and repeatability.
- Complex Geometries: Deep draw hydroforming allows for the creation of parts with complex geometries, including sharp angles, deep recesses, and tapered shapes. The die must be carefully designed to ensure smooth material flow into these areas.
- Surface Finish: The die’s surface finish plays an important role in determining the final quality of the part. A smooth die surface ensures that the part has a clean, polished appearance and reduces the need for post-processing.
3.3 Die Holder
The die holder secures the die in place within the hydroforming press. Proper alignment and stability of the die are critical to ensuring that the metal sheet is accurately formed and that the press operates efficiently.
- Alignment Precision: The die holder ensures that the die remains properly aligned with the diaphragm and blank holder, preventing defects caused by misalignment.
4. Clamping and Sealing System
The clamping system holds the metal blank securely in place over the die during the forming process. The clamping system is essential for ensuring that the metal does not shift, wrinkle, or become misaligned while it is being drawn into the die.
4.1 Blank Holder (Binder)
The blank holder or binder is a key part of the clamping system that applies uniform pressure to the edges of the metal blank. It prevents the material from moving during the forming process and controls the flow of the metal as it is drawn into the die.
- Controlling Material Flow: The blank holder ensures that the material flows smoothly into the die, preventing wrinkling or tearing that could occur if the blank moves during the forming process.
- Pressure Application: The pressure applied by the blank holder must be carefully controlled. Too little pressure could result in the blank slipping or wrinkling, while too much pressure could inhibit material flow and lead to tearing.
4.2 Sealing System
The sealing system ensures that the hydraulic fluid is contained within the diaphragm and does not leak during the forming process. A tight seal between the diaphragm, blank holder, and die is essential for maintaining uniform pressure and ensuring that the part is formed correctly.
- Rubber Seals: High-pressure rubber seals are commonly used to create a tight seal between the blank and the die, preventing any loss of hydraulic pressure.
5. Control System
Modern deep draw hydroforming presses are equipped with sophisticated control systems that manage the various stages of the forming process. These systems allow operators to monitor and adjust critical parameters such as pressure, temperature, and cycle time in real-time, ensuring precision and repeatability.
5.1 CNC (Computer Numerical Control)
Many hydroforming presses use CNC control systems to automate the forming process. CNC systems can control the rate at which hydraulic pressure is applied, the movement of the diaphragm, and the cycle timing, ensuring that parts are produced to exact specifications.
- Multi-Axis Control: CNC systems can control the motion of the diaphragm and die along multiple axes, allowing for greater flexibility in forming complex shapes.
- Automated Pressure Control: The CNC system adjusts the hydraulic pressure applied during different stages of the forming process, ensuring that the metal is shaped gradually and accurately.
5.2 PLC (Programmable Logic Controller)
A PLC system is often used to automate and sequence the hydroforming process. The PLC controls the hydraulic pumps, valves, clamping system, and other components to ensure that each stage of the process is executed in the correct order.
- Process Automation: PLC systems automate key functions such as pressure application, blank clamping, and part ejection, reducing the need for manual intervention.
- Process Monitoring: Sensors throughout the press feed data back to the PLC, allowing operators to monitor the system’s performance and make adjustments as needed.
6. Ejection System
Once the part has been formed, it must be safely removed from the die. The ejection system helps lift the formed part out of the die without damaging the newly created component.
6.1 Hydraulic Ejectors
Many hydroforming presses use hydraulic ejectors to gently lift the part out of the die after the forming process is complete. This helps prevent damage to the part, especially when dealing with delicate or complex geometries.
- Controlled Part Removal: The hydraulic ejectors work in tandem with the diaphragm to ensure smooth, controlled part removal without deformation.
6.2 Automated Ejection Systems
In highly automated production lines, robotic arms or automated ejection systems may be used to remove parts from the press and transport them to the next stage of production, such as trimming, inspection, or packaging.
- Robotic Handling: Automated systems can quickly and precisely handle parts, reducing the risk of damage and increasing production efficiency.
7. Safety Systems
Given the high pressures involved in deep draw hydroforming, safety systems are essential to protect both operators and the machine from accidents or malfunctions.
7.1 Safety Guards and Enclosures
Most hydroforming presses are equipped with safety guards or enclosures to protect operators from moving parts and high-pressure systems.
- Interlocks: These guards often include interlock systems that prevent the press from operating unless the safety guards are in place, ensuring a safe working environment.
7.2 Pressure Relief Systems
Pressure relief valves are integrated into the hydraulic system to prevent overpressure conditions that could damage the press or cause injury.
- Automatic Release: In the event of a system malfunction or excessive pressure buildup, the pressure relief valves automatically release fluid to reduce the pressure and prevent damage.
Conclusion
The key components of a deep draw sheet hydroforming press work together to create a highly efficient and precise metal forming machine capable of producing complex, high-quality parts. From the hydraulic system that generates the forming pressure to the diaphragm that ensures even pressure distribution, each element plays a critical role in the success of the hydroforming process. By understanding how these components function, manufacturers can optimize their operations, reduce costs, and improve part quality across a wide range of industries, including automotive, aerospace, and medical devices. As demand for lightweight, high-performance components continues to grow, deep draw hydroforming will remain an essential tool for modern manufacturing.
Deep Draw Hydroforming Process: A Comprehensive Overview (2000 Words)
Deep draw hydroforming is a highly advanced sheet metal forming process that combines elements of both traditional deep drawing and hydroforming. It allows for the creation of complex, high-precision, and deep-drawn parts by utilizing hydraulic pressure to form sheet metal into intricate shapes. This process is widely used in industries such as automotive, aerospace, medical devices, and consumer electronics due to its ability to produce lightweight, seamless, and durable components.
The process of deep draw hydroforming involves the controlled application of hydraulic pressure to deform a flat sheet metal blank into a three-dimensional shape, typically with depths greater than its diameter. By using a flexible diaphragm filled with hydraulic fluid, the metal is pressed against a die, forming the desired geometry with minimal defects such as tearing, thinning, or wrinkling.
In this article, we will explore the step-by-step process of deep draw hydroforming, its key principles, the role of each component, and its benefits and limitations.
1. Overview of the Deep Draw Hydroforming Process
Deep draw hydroforming is a process that can produce parts with deep, complex geometries that would be difficult to achieve with conventional stamping or deep drawing techniques. The term “deep draw” refers to the fact that the depth of the part produced is greater than its diameter.
The process involves using hydraulic fluid pressure to stretch and deform a sheet of metal into a rigid die, producing a part that matches the die’s shape. In contrast to traditional deep drawing, where a punch mechanically forces the metal into the die, deep draw hydroforming uses hydraulic pressure to push a flexible diaphragm against the metal sheet, forcing it into the die.
Key features of the deep draw hydroforming process:
- Uniform pressure application over the entire surface of the blank.
- High formability, allowing for deep, intricate shapes without significant material thinning or tearing.
- Single-sided tooling, reducing the cost and complexity of dies.
2. The Basic Steps of the Deep Draw Hydroforming Process
The deep draw hydroforming process follows a series of carefully controlled steps to transform a flat metal sheet into a deep, intricately shaped part. Each stage plays a critical role in ensuring the final part meets the desired specifications for accuracy, strength, and surface quality.
2.1 Blank Preparation
The process begins with the selection and preparation of the metal blank. The blank is typically a flat sheet of material that has been cut to the appropriate size for the part being formed. The blank’s material must possess high formability and ductility to withstand the stretching and drawing required in deep draw hydroforming.
Common materials used in deep draw hydroforming include:
- Aluminum: Lightweight, corrosion-resistant, and highly formable.
- Steel: Durable and versatile, often used for automotive and industrial applications.
- Stainless steel: Resistant to corrosion and ideal for food, medical, and chemical applications.
- Titanium: Lightweight and strong, often used in aerospace and medical devices.
Blank thickness is also an important factor. Thicker sheets may be more resistant to tearing but require higher forming pressures, while thinner sheets may be more prone to defects like thinning or rupture.
2.2 Die Setup and Clamping
Once the blank is prepared, it is placed over the die in the lower section of the hydroforming press. The die is a solid mold that defines the shape of the final part. The blank is then secured in place using a clamping system or blank holder, which prevents it from shifting or wrinkling during the forming process.
- Die design: The die is custom-machined to match the desired geometry of the part, and it must be precisely aligned with the diaphragm and hydraulic system to ensure accurate part formation.
- Clamping system: Proper clamping is essential for controlling the flow of the metal into the die and avoiding defects such as wrinkling, thinning, or tearing.
2.3 Hydraulic Pressure Application
After the blank is securely clamped over the die, hydraulic fluid is pumped into the diaphragm or bladder located in the upper section of the press. The hydraulic system gradually increases the pressure, causing the diaphragm to expand and apply even force across the entire surface of the metal sheet.
- Hydraulic pressure control: The application of hydraulic pressure is a key factor in determining the success of the forming process. The pressure must be applied gradually to prevent sudden deformation, which could cause tearing or thinning of the material.
- Uniform pressure distribution: The flexibility of the diaphragm ensures that pressure is evenly distributed, allowing the material to flow smoothly into the die without localized thinning or stress points.
At this stage, the hydraulic fluid provides the necessary force to stretch and deform the metal into the shape of the die. Unlike traditional deep drawing, which uses a mechanical punch to shape the metal, deep draw hydroforming applies pressure through the flexible diaphragm, resulting in smoother material flow and reduced risk of defects.
2.4 Deep Drawing and Material Flow
As hydraulic pressure continues to build, the blank is drawn deeper into the die, conforming to its shape. The material undergoes plastic deformation, meaning it permanently takes on the shape of the die once formed. During this stage, the sheet metal is stretched and drawn into the die cavity, forming a deep, hollow shape.
- Material flow: The metal flows from the edges of the blank toward the center, with the flexible diaphragm ensuring uniform distribution of pressure across the entire blank. This prevents wrinkling or thinning that might otherwise occur with traditional deep drawing methods.
- Deep draw capability: Deep draw hydroforming allows for the production of parts with greater depths than conventional methods, making it ideal for applications requiring deep recesses, complex curves, or sharp angles.
This stage of the process is where the benefits of hydroforming over traditional deep drawing become most apparent. The use of hydraulic pressure ensures more controlled material deformation, enabling the creation of complex geometries without the need for multiple tooling stages or secondary forming operations.
2.5 Pressure Holding and Stabilization
Once the part has been fully formed into the die, the hydraulic pressure is maintained for a short period to allow the material to stabilize. This pressure holding phase ensures that the part retains its final shape and dimensional accuracy without springback or deformation.
- Pressure holding: Holding the pressure for a brief time ensures that any internal stresses within the material are relieved, helping the part retain its shape after forming.
- Dimensional accuracy: By maintaining pressure during this stage, the press ensures that the part conforms to the precise shape of the die, achieving tight tolerances and high-quality surface finishes.
2.6 Pressure Release and Part Removal
After the pressure holding phase, the hydraulic pressure is gradually released, allowing the diaphragm to retract. The clamping system is then disengaged, and the finished part is removed from the die.
- Controlled pressure release: A gradual release of pressure helps prevent springback, a phenomenon where the metal tries to return to its original shape due to residual stresses.
- Part removal: Once the pressure has been fully released, the part is gently lifted from the die, either manually or through the use of an ejection system.
At this point, the deep-drawn part is complete. Depending on the application, it may undergo additional post-processing steps, such as trimming, deburring, or surface finishing.
3. Advantages of Deep Draw Hydroforming
Deep draw hydroforming offers several advantages over traditional deep drawing and other metal forming techniques. These advantages make the process particularly attractive for industries that require high-precision, lightweight, and complex components.
3.1 Uniform Pressure Application
One of the most significant advantages of deep draw hydroforming is the uniform application of pressure. The flexible diaphragm ensures that the hydraulic pressure is applied evenly across the entire surface of the metal blank, leading to more consistent material deformation and fewer defects.
- Reduced risk of defects: The uniform pressure helps prevent common deep drawing defects such as wrinkling, tearing, and thinning, which are often caused by uneven material flow in traditional deep drawing.
- Improved surface quality: Parts formed through hydroforming generally have smooth surfaces with minimal imperfections, reducing the need for secondary finishing operations.
3.2 Complex Geometry Capabilities
Deep draw hydroforming is ideal for producing parts with complex geometries that would be difficult or impossible to achieve using conventional deep drawing or stamping methods. The use of hydraulic pressure allows for the creation of parts with deep recesses, sharp angles, compound curves, and variable wall thicknesses.
- Greater design flexibility: Manufacturers can design parts with more complex shapes, enhancing product functionality and performance.
- Seamless construction: The ability to form intricate shapes in a single operation reduces the need for welding or joining, leading to stronger, seamless components.
3.3 Material Efficiency
Hydroforming minimizes material waste by reducing the need for excess trimming or cutting. The blank is directly formed into the desired shape, allowing manufacturers to make more efficient use of raw materials.
- Less material waste: The process generates fewer scraps compared to traditional stamping or deep drawing methods.
- Reduced tooling costs: Since only a single-sided die is required in deep draw hydroforming, tooling costs are significantly lower than those associated with conventional deep drawing, which requires both a male and female die.
3.4 Lower Tooling Costs
Deep draw hydroforming uses a single-sided die, which reduces the cost and complexity of tooling compared to traditional deep drawing methods that require both a punch and die set. This also reduces the time and cost of die setup, making it an economical option for producing both prototypes and production parts.
4. Applications of Deep Draw Hydroforming
Deep draw hydroforming is used in a wide range of industries, including automotive, aerospace, medical devices, consumer electronics, and energy. Its ability to produce lightweight, high-strength components with intricate geometries makes it a versatile and valuable manufacturing process.
4.1 Automotive Industry
In the automotive industry, deep draw hydroforming is used to manufacture body panels, chassis components, and structural parts. These components require high strength-to-weight ratios and precise dimensions to improve vehicle performance and fuel efficiency.
- Body panels: Car doors, hoods, and trunk lids benefit from the smooth surfaces and lightweight construction offered by hydroforming.
- Structural parts: Hydroformed crossmembers, subframes, and crash-resistant components enhance vehicle safety and performance.
4.2 Aerospace Industry
In aerospace, deep draw hydroforming is used to produce lightweight, high-strength components such as fuselage panels, engine housings, and wing ribs. Weight reduction is critical in aerospace applications, where every pound saved translates to greater fuel efficiency and longer flight range.
- Fuselage panels: Hydroforming allows for the creation of seamless, aerodynamically efficient aircraft panels.
- Engine components: Hydroformed turbine housings and ducts can withstand high temperatures and pressures while maintaining precise dimensional accuracy.
4.3 Medical Devices
In the medical industry, deep draw hydroforming is used to produce surgical instruments, implants, and medical device housings. These components require high precision, biocompatibility, and smooth surfaces for hygiene and performance.
- Surgical instruments: Forceps, scalpels, and retractors benefit from the smooth, seamless surfaces created by hydroforming.
- Implants: Custom-shaped implants, such as joint replacements, are formed with precise geometries to fit patient-specific needs.
4.4 Consumer Electronics
Deep draw hydroforming is also used to create lightweight, durable components for consumer electronics and appliances. Components such as smartphone housings, laptop frames, and appliance panels are often hydroformed to provide sleek, modern designs with minimal material usage.
- Smartphone housings: Hydroforming creates smooth, durable, and aesthetically pleasing casings for modern electronic devices.
- Appliance panels: Refrigerator doors, oven liners, and other appliance components benefit from the high-quality finishes and corrosion-resistant properties offered by hydroforming.
5. Limitations of Deep Draw Hydroforming
While deep draw hydroforming offers numerous advantages, it is not without limitations:
5.1 Higher Initial Equipment Cost
Hydroforming presses, particularly those capable of handling high pressures, can be more expensive than traditional deep drawing or stamping equipment. This higher initial investment may not be feasible for small-scale operations or low-volume production runs.
5.2 Longer Cycle Times
Deep draw hydroforming can have longer cycle times compared to traditional deep drawing, especially for complex or deep parts. The process of gradually applying and releasing hydraulic pressure takes more time than mechanical deep drawing methods.
5.3 Material Limitations
Not all materials are suitable for deep draw hydroforming. Certain metals, particularly those with low ductility or poor formability, may not be able to withstand the high pressures involved in the hydroforming process without tearing or cracking.
Conclusion
Deep draw hydroforming is a highly versatile and efficient metal forming process that allows manufacturers to create complex, deep-drawn parts with high precision, minimal defects, and reduced material waste. By using hydraulic pressure to shape metal into intricate geometries, the process offers numerous advantages over traditional deep drawing, including lower tooling costs, uniform pressure application, and the ability to form complex shapes. These benefits make deep draw hydroforming a valuable tool for industries such as automotive, aerospace, medical devices, and consumer goods, where lightweight, high-performance components are essential.
Despite some limitations, such as higher initial equipment costs and longer cycle times, the advantages of deep draw hydroforming make it an increasingly popular choice for modern manufacturing, particularly in industries that demand high-precision, lightweight, and durable parts. As technology continues to advance, deep draw hydroforming is expected to play an even greater role in shaping the future of manufacturing
Applications of Deep Draw Hydroforming
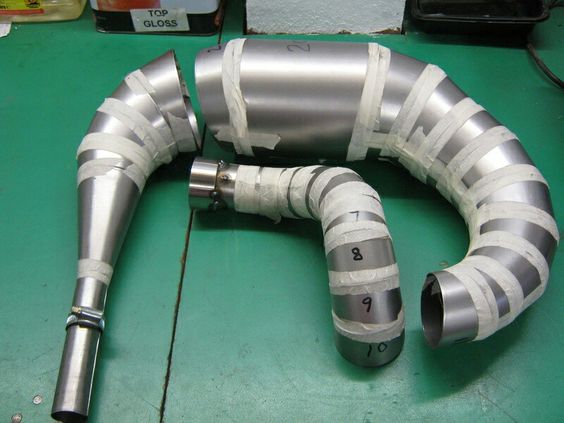
Deep draw hydroforming is a metal forming process that combines elements of deep drawing and hydroforming, using hydraulic pressure to form complex, high-precision parts from sheet metal. This method allows manufacturers to produce deep-drawn, lightweight, and seamless components with intricate geometries, high strength-to-weight ratios, and superior surface finishes. The technology is widely used in a variety of industries, including automotive, aerospace, medical devices, consumer electronics, and energy.
Deep draw hydroforming is particularly valued for its ability to create parts with deep recesses, sharp angles, complex curves, and variable wall thicknesses, often in a single operation. These advantages have led to its adoption in applications where traditional metal forming methods, such as stamping or conventional deep drawing, would be impractical or less efficient.
This comprehensive overview explores the key applications of deep draw hydroforming across several industries, highlighting specific components, the benefits they offer, and how the process enhances performance, efficiency, and quality.
1. Automotive Industry
The automotive industry is one of the largest users of deep draw hydroforming, driven by the need for lightweight, high-strength components that improve vehicle performance, fuel efficiency, and safety. As automakers seek to reduce vehicle weight without sacrificing durability or crashworthiness, deep draw hydroforming has become a critical tool for producing complex, precision-engineered parts.
1.1 Body Panels and Exterior Components
Automotive body panels must be lightweight, strong, and aerodynamic. Deep draw hydroforming allows manufacturers to create smooth, seamless panels with complex curves and sharp contours, ideal for modern vehicle designs. Key components include:
- Car Hoods: Deep draw hydroforming is used to manufacture lightweight yet strong hoods that offer improved fuel efficiency while maintaining structural integrity. The process also ensures that the surface finish is smooth, reducing the need for additional post-processing.
- Doors and Trunk Lids: Doors and trunk lids benefit from the strength and weight savings offered by hydroforming. The ability to create complex shapes in a single operation allows for precise fits and alignment, contributing to vehicle safety and aesthetic appeal.
1.2 Chassis and Structural Components
The chassis is the backbone of any vehicle, and its components must offer high strength and rigidity while minimizing weight. Deep draw hydroforming is ideal for creating lightweight structural components that enhance vehicle performance and safety.
- Crossmembers and Subframes: Hydroforming allows for the creation of crossmembers and subframes that are both lightweight and strong, contributing to better crash resistance and vehicle dynamics. These components are essential for distributing loads and absorbing energy in the event of a collision.
- Suspension Components: Suspension arms and other parts of the vehicle’s suspension system are often hydroformed to improve strength, reduce weight, and enhance handling. The process also ensures that these components have consistent wall thickness, which is critical for performance.
1.3 Fuel and Exhaust Systems
Fuel and exhaust systems must be designed to handle high pressures, temperatures, and corrosive materials. Deep draw hydroforming is used to create seamless, leak-proof components for these systems, improving durability and performance.
- Fuel Tanks: Hydroformed fuel tanks are lighter and more durable than traditional tanks. They offer improved fuel efficiency and reduce the risk of leaks due to their seamless construction.
- Exhaust Manifolds: Hydroformed exhaust manifolds and mufflers have smoother internal surfaces, which enhance airflow, reduce back pressure, and improve engine efficiency.
1.4 Electric Vehicles (EVs) and Lightweighting
As the automotive industry shifts toward electric vehicles (EVs), reducing vehicle weight has become even more critical to extending driving range and improving battery efficiency. Deep draw hydroforming plays a key role in the lightweighting of EV components.
- Battery Enclosures: Hydroforming is used to create lightweight yet strong battery enclosures that protect EV battery packs from impacts and vibrations while optimizing space and weight distribution.
- Lightweight Frames: The ability to form complex, high-strength parts with minimal material waste makes hydroforming ideal for producing lightweight frames and chassis components for EVs.
2. Aerospace Industry
In the aerospace industry, weight reduction and structural integrity are paramount. Aircraft components must be lightweight to improve fuel efficiency and payload capacity, while also being strong enough to withstand extreme pressures, temperatures, and stresses during flight. Deep draw hydroforming is widely used to produce lightweight, high-strength components for both commercial and military aircraft.
2.1 Fuselage and Structural Components
The fuselage is the main body of an aircraft, and its structural components must provide strength and rigidity while minimizing weight. Deep draw hydroforming is used to create fuselage frames, bulkheads, and stringers that offer high strength-to-weight ratios.
- Fuselage Frames: Hydroforming is used to create fuselage frames that support the aircraft’s outer skin. These frames must be lightweight to reduce the overall weight of the aircraft, while still being strong enough to handle the stresses of flight.
- Stringers and Longerons: Hydroformed stringers and longerons provide additional structural support to the fuselage and wings, improving the aircraft’s aerodynamic performance and structural integrity.
2.2 Engine Components
Aircraft engines operate under extreme conditions, with components exposed to high temperatures and pressures. Deep draw hydroforming is used to manufacture engine components that require tight tolerances, high strength, and heat resistance.
- Turbine Housings: Turbine housings formed through hydroforming are lightweight and able to withstand the extreme forces and temperatures generated by jet engines. The process ensures precision and durability, which are critical for engine performance.
- Exhaust Components: Exhaust ducts and manifolds are also hydroformed to create smooth, aerodynamic shapes that improve airflow and reduce turbulence, resulting in more efficient engine operation.
2.3 Lightweighting for Fuel Efficiency
Fuel efficiency is a major concern in the aerospace industry, and reducing aircraft weight is essential to improving it. Hydroforming allows manufacturers to produce lightweight components without sacrificing strength, contributing to more fuel-efficient aircraft.
- Wing Ribs and Spars: Wing ribs and spars are critical for maintaining the structural integrity of the wings while reducing overall weight. Hydroformed parts provide the necessary strength while improving the aircraft’s aerodynamics.
- Landing Gear Components: Lightweight yet strong landing gear components are essential for withstanding the forces of takeoff and landing. Hydroformed parts offer the precision and durability needed for these critical components.
3. Medical Devices
In the medical industry, precision, surface finish, and biocompatibility are critical factors in the design and manufacture of medical devices. Deep draw hydroforming is used to create high-precision components for surgical instruments, implants, and medical equipment that require exacting standards for performance, safety, and hygiene.
3.1 Surgical Instruments
Surgical instruments must be lightweight, durable, and easy to sterilize. Deep draw hydroforming allows manufacturers to produce smooth, seamless instruments with high precision and excellent surface finishes.
- Forceps, Scalpels, and Retractors: Hydroforming is used to create forceps, scalpels, and retractors with smooth, sterile surfaces that reduce the risk of contamination and infection during surgery. The process ensures that these instruments are lightweight, strong, and biocompatible.
- Precision Cutting Tools: Surgical cutting tools benefit from the tight tolerances and sharp edges that can be achieved through hydroforming, enhancing their performance in delicate medical procedures.
3.2 Medical Implants
Medical implants, such as orthopedic devices, dental implants, and joint replacements, require materials that are biocompatible and strong enough to withstand the stresses placed on them by the human body. Deep draw hydroforming allows manufacturers to create customized, patient-specific implants with tight tolerances and smooth finishes.
- Hip and Knee Replacements: Hydroformed hip and knee replacements offer a precise fit, improving the performance and longevity of the implant. The smooth surfaces created by hydroforming reduce wear and friction, improving patient outcomes.
- Spinal Implants: Spinal implants require intricate shapes and exacting tolerances to ensure proper alignment and function. Hydroforming allows for the creation of these complex components with high precision.
3.3 Diagnostic Equipment
In addition to surgical instruments and implants, deep draw hydroforming is used to create components for diagnostic equipment such as MRI machines, X-ray equipment, and other medical devices.
- Casing and Housings: Hydroformed casings and housings for medical devices are lightweight, durable, and easy to clean. The smooth, seamless surfaces provided by hydroforming help maintain the sterility and cleanliness of diagnostic equipment.
- Electromagnetic Shields: In MRI machines and other diagnostic devices, hydroformed electromagnetic shields are used to protect sensitive electronics from interference, ensuring accurate readings and diagnoses.
4. Consumer Electronics and Appliances
Deep draw hydroforming is widely used in the production of consumer electronics and appliances due to its ability to create lightweight, aesthetically pleasing, and durable components. The process is especially useful for creating sleek, modern designs that are in high demand in today’s consumer products.
4.1 Consumer Electronics
In the world of consumer electronics, deep draw hydroforming is used to manufacture housings, frames, and structural components for devices like smartphones, laptops, and tablets. These products require thin, lightweight parts with precise geometries, making hydroforming an ideal solution.
- Smartphone Housings: Hydroformed aluminum and stainless steel housings are commonly used in high-end smartphones, where a combination of strength, durability, and design aesthetics is required. The smooth surface finish and seamless construction provided by hydroforming ensure that the device has a premium look and feel.
- Laptop Frames: Hydroforming is also used to create the frames and chassis of laptops, providing the necessary structural support while maintaining a slim, lightweight design.
4.2 Home Appliances
The appliance industry benefits from deep draw hydroforming for the production of kitchen appliances, laundry machines, and other home goods. The ability to form large, complex parts with smooth surfaces and minimal defects makes hydroforming ideal for creating both functional and aesthetically pleasing components.
- Refrigerator Doors and Panels: Hydroforming is used to create the outer panels and doors of refrigerators, where the process ensures a smooth, high-quality surface finish that is resistant to corrosion and easy to clean.
- Oven Liners and Control Panels: In ovens and other kitchen appliances, hydroformed components are used to create control panels and interior liners that can withstand high temperatures while maintaining their shape and finish.
5. Energy and Power Generation
The energy and power generation industry also benefits from the use of deep draw hydroforming, particularly in the production of components for wind turbines, solar energy systems, and oil and gas infrastructure. The process allows for the creation of large, complex parts that can withstand harsh environmental conditions and demanding operational requirements.
5.1 Wind Turbine Components
In wind energy systems, deep draw hydroforming is used to produce structural components for wind turbines, including the hub, nacelle, and blades. These components must be both lightweight and durable to maximize energy output and withstand the elements.
- Nacelle Housings: Hydroformed nacelle housings provide the necessary protection for the sensitive electrical components inside the turbine, while maintaining a streamlined design that reduces wind resistance.
- Blade Root Fittings: Hydroforming is used to create the root fittings that connect the wind turbine blades to the hub, ensuring a secure, durable connection that can withstand the forces generated by the spinning blades.
5.2 Solar Energy Components
In solar energy systems, hydroforming is used to create mounting brackets and frames for solar panels, ensuring that they are lightweight, durable, and able to withstand harsh weather conditions.
- Solar Panel Frames: Hydroformed frames provide the necessary support for solar panels, while maintaining a lightweight design that reduces installation costs and improves energy efficiency.
5.3 Oil and Gas Components
Deep draw hydroforming is also used in the oil and gas industry to produce pressure vessels, pipes, and fittings that can withstand high pressures and corrosive environments.
- Pressure Vessels: Hydroformed pressure vessels are used to store and transport oil, gas, and other fluids under high pressure, ensuring safety and reliability.
6. Sporting Goods and Bicycles
In the world of sporting goods and bicycles, weight, performance, and durability are critical factors in product design. Deep draw hydroforming is used to manufacture components that enhance the performance of sports equipment and bicycles by reducing weight without sacrificing strength.
6.1 Bicycle Frames
One of the most common applications of deep draw hydroforming in the sporting goods industry is the production of bicycle frames. Hydroforming allows manufacturers to create lightweight, strong frames with complex geometries that improve the aerodynamics and performance of the bike.
- Mountain Bikes and Road Bikes: Hydroformed aluminum and titanium frames are used in both mountain bikes and road bikes, where the ability to create tapered tubes and customized shapes enhances both the strength and comfort of the bike.
- Electric Bicycles (e-Bikes): With the rise of e-bikes, hydroforming has become even more important, allowing manufacturers to integrate battery compartments and electrical components into the frame without adding unnecessary weight.
6.2 Golf Clubs and Other Sporting Goods
In addition to bicycles, hydroforming is used in the production of golf clubs, hockey sticks, tennis rackets, and other sports equipment where lightweight, high-strength materials are essential for performance.
- Golf Club Shafts: Hydroforming allows for the creation of precision-engineered golf club shafts, where the weight distribution and stiffness can be tailored to improve the player’s swing and overall performance.
- Tennis Rackets and Hockey Sticks: The ability to create lightweight, durable frames with minimal material wastage makes hydroforming ideal for producing high-performance tennis rackets and hockey sticks.
Conclusion
Deep draw hydroforming is a versatile and powerful metal forming process that enables manufacturers to produce complex, lightweight, and high-precision parts across a wide range of industries. From automotive body panels and aircraft engine components to medical implants, consumer electronics, and sporting goods, the process offers significant advantages over traditional metal forming techniques, including lower tooling costs, reduced material waste, and the ability to form intricate geometries with seamless construction.
As industries continue to demand more efficient, sustainable, and high-performance components, deep draw hydroforming will play an increasingly important role in modern manufacturing, driving innovation in product design and production efficiency.
Hydroforming Press for Dished Ends: Overview, Process, and Applications
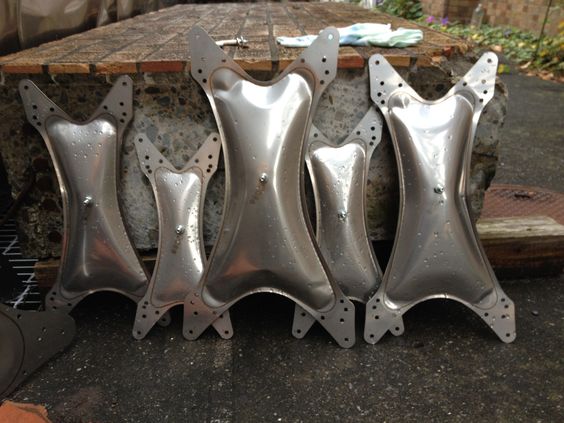
A hydroforming press for dished ends is a specialized machine used to form dished ends—which are concave or convex metal components—by applying hydraulic pressure to a metal blank. Dished ends, also known as tank heads or end caps, are typically used to close the ends of cylindrical vessels such as pressure tanks, boilers, pipelines, and storage tanks. These parts must have high precision, uniform thickness, and sufficient strength to withstand internal or external pressures, making hydroforming an ideal method for their production.
By using hydroforming technology, dished ends can be formed from a flat sheet of metal without the need for welding or joining, resulting in seamless construction, which improves both the mechanical strength and durability of the part. This article provides a comprehensive overview of the hydroforming press used for dished ends, the process involved, key components, and the industries where these parts are essential.
1. Overview of Hydroforming for Dished Ends
Dished ends are critical components in many industries, as they are used to close off the ends of cylindrical pressure vessels and storage containers. They are typically made from metals like steel, stainless steel, aluminum, or titanium, depending on the application and operating conditions. Hydroforming dished ends involves the use of hydraulic pressure to shape a metal blank into the desired concave or convex form, often with minimal thinning or defects.
Traditional methods for forming dished ends, such as spinning, pressing, or welding, may introduce inconsistencies, material wastage, or weaker joints. However, hydroforming provides several advantages:
- Seamless construction eliminates weak points where leaks or failures might occur.
- Precise control over material flow results in uniform thickness and better strength.
- The process can produce complex geometries with tight tolerances in a single forming operation.
Hydroforming presses for dished ends are engineered to handle the large-scale production of tank heads and end caps in industries such as oil and gas, chemical processing, power generation, and food processing.
2. Hydroforming Process for Dished Ends
The hydroforming process for dished ends involves several key steps that ensure the metal blank is shaped accurately and consistently. The following is a step-by-step breakdown of the process:
2.1 Blank Preparation
The process begins with the selection and preparation of the metal blank. The blank is usually a circular sheet of metal cut to the size needed for the dished end. The material is chosen based on the specific application, with common choices including carbon steel, stainless steel, and aluminum alloys.
- Material Considerations: The material must have good formability to withstand the high pressures during the hydroforming process without cracking or excessive thinning.
2.2 Loading and Clamping
Once the blank is prepared, it is positioned over the forming die in the hydroforming press. The blank is securely clamped to ensure that it does not move during the forming process.
- Clamping System: A high-strength clamping system ensures that the blank remains in place and does not wrinkle or misalign while pressure is applied.
2.3 Application of Hydraulic Pressure
Hydraulic fluid is pumped into the diaphragm or bladder located in the upper part of the hydroforming press. As the fluid pressure increases, the flexible diaphragm presses against the blank, forcing it to conform to the shape of the die beneath it.
- Pressure Control: The hydraulic pressure is increased gradually to prevent sudden deformation or tearing of the material. Precise control of the pressure is critical for ensuring uniform wall thickness and material flow.
- Even Pressure Distribution: The flexible diaphragm applies pressure uniformly across the blank, allowing the material to flow smoothly into the die without localized thinning or stress concentrations.
2.4 Forming the Dished End
As pressure continues to build, the blank is shaped into the dished end form. The metal stretches and conforms to the concave or convex die, creating the desired curvature and depth.
- Material Flow: The material flows from the outer edges toward the center of the die, forming the characteristic shape of the dished end.
- Deep Drawing Capability: The hydroforming process allows for the production of dished ends with deep, complex shapes that would be difficult to achieve with traditional methods.
2.5 Pressure Holding and Release
Once the dished end has taken its final shape, the hydraulic pressure is maintained for a short period to allow the material to stabilize. This ensures that the part retains its shape and does not spring back.
- Pressure Holding: Maintaining pressure helps relieve internal stresses in the material, improving the structural integrity of the part.
- Controlled Release: The pressure is gradually released, and the diaphragm retracts, allowing the clamping system to disengage.
2.6 Part Removal and Finishing
The formed dished end is removed from the die and may undergo additional finishing processes such as trimming, deburring, or polishing. In some cases, the part may also be subjected to heat treatment to enhance its mechanical properties.
- Post-Processing: Depending on the application, the dished end may be heat-treated to improve strength and toughness or polished to achieve a smooth surface finish.
3. Components of a Hydroforming Press for Dished Ends
A hydroforming press for dished ends consists of several key components that work together to ensure precision and efficiency in forming large, complex parts. These components include:
3.1 Hydraulic System
The hydraulic system is responsible for generating the high pressure needed to shape the metal blank. It includes pumps, valves, and accumulators that regulate the flow and pressure of hydraulic fluid.
- Hydraulic Pumps: Generate the pressure needed to force the diaphragm against the blank, with pressures often reaching tens of thousands of psi.
- Accumulators: Store pressurized fluid and release it as needed to ensure consistent pressure throughout the forming cycle.
3.2 Diaphragm or Bladder
The diaphragm is a flexible membrane that holds the hydraulic fluid and transmits pressure evenly across the blank. Made from durable materials such as rubber or urethane, the diaphragm must be able to withstand high pressures without rupturing.
- Even Pressure Distribution: The diaphragm ensures that pressure is applied uniformly, minimizing defects such as thinning, tearing, or wrinkling.
3.3 Die (Tooling)
The die defines the shape of the dished end and is typically made from hardened steel or cast iron. The die’s design must precisely match the geometry of the desired part to ensure accurate forming.
- Single-Sided Die: In hydroforming, only a single-sided die is required, as the diaphragm replaces the upper die used in traditional methods.
3.4 Clamping System
The clamping system holds the blank in place during the forming process, ensuring that it does not move or wrinkle.
- High-Strength Clamps: These clamps are designed to apply uniform pressure to the blank, preventing any movement during the application of hydraulic pressure.
3.5 Control System
The control system manages the pressure application, cycle time, and other critical parameters of the hydroforming process. Modern presses often use CNC (Computer Numerical Control) or PLC (Programmable Logic Controller) systems to ensure precise control and repeatability.
4. Applications of Dished Ends
Dished ends are used in a wide range of industries where pressure vessels or storage tanks are required to contain liquids, gases, or other materials under pressure. Below are some of the key applications:
4.1 Pressure Vessels and Boilers
Dished ends are commonly used to close the ends of pressure vessels and boilers in industries such as oil and gas, chemical processing, and power generation. These vessels are designed to hold gases or liquids at high pressures, and the strength and integrity of the dished ends are critical for safety.
- Boiler Ends: Dished ends are used in boilers to contain steam and prevent leaks. The seamless construction provided by hydroforming ensures that the ends can withstand high internal pressures without failure.
4.2 Storage Tanks
Storage tanks used in industries such as water treatment, food processing, and pharmaceuticals often have dished ends to improve the structural integrity of the tank and provide a smoother flow of liquids.
- Chemical Storage Tanks: Dished ends help prevent the buildup of residues and ensure that tanks can be safely pressurized, reducing the risk of leaks or contamination.
4.3 Pipelines and Pipe Fittings
Dished ends are also used in pipelines and pipe fittings to cap off sections of pipes or provide structural reinforcement at the end of a pipe.
- Pipe Caps: Hydroformed dished ends are commonly used as end caps for large-diameter pipelines, providing a leak-proof seal and improving the overall durability of the pipe.
4.4 Heat Exchangers
In heat exchangers, dished ends are used to close off the ends of the vessel and ensure that the internal fluids remain contained while transferring heat.
- End Caps for Heat Exchangers: The precise forming capability of hydroforming ensures that the dished ends are able to withstand the high temperatures and pressures involved in heat exchange applications.
5. Advantages of Hydroforming for Dished Ends
Hydroforming offers several key advantages over traditional forming methods when it comes to producing dished ends:
5.1 Seamless Construction
One of the most significant advantages of hydroforming is the ability to produce seamless dished ends. This eliminates the need for welding or joining, which can introduce weak points or potential leak paths.
- Improved Strength: Seamless construction improves the overall strength and durability of the dished end, making it ideal for high-pressure applications.
- Leak Prevention: Without welded seams, there is less risk of leaks, especially in applications where the dished ends are used in pressure vessels or storage tanks.
5.2 Uniform Wall Thickness
Hydroforming allows for precise control over material flow, resulting in dished ends with uniform wall thickness. This is especially important in pressure vessel applications, where consistent thickness ensures that the part can withstand internal pressures without failing.
- Better Pressure Distribution: Uniform wall thickness helps distribute pressure evenly throughout the dished end, reducing the risk of localized stress points.
5.3 Reduced Material Wastage
The hydroforming process minimizes material wastage by forming the metal blank directly into the desired shape, with minimal trimming or cutting required.
- Cost Savings: Reduced material waste leads to lower production costs, making hydroforming an economical choice for large-scale production of dished ends.
5.4 Complex Shapes
Hydroforming is ideal for creating complex shapes and deep geometries, allowing manufacturers to produce dished ends with customized curvatures and non-standard sizes.
- Design Flexibility: The ability to form intricate shapes gives manufacturers more design flexibility, making it easier to meet specific customer requirements.
Conclusion
The hydroforming press for dished ends is an advanced and highly efficient tool for producing high-quality, seamless components used in pressure vessels, boilers, storage tanks, and more. By applying uniform hydraulic pressure to a metal blank, hydroforming allows for the creation of complex, durable, and precise dished ends with uniform wall thickness, seamless construction, and improved strength. These advantages make hydroforming the preferred choice in industries that require components capable of withstanding high pressures and harsh operating environments.
As industries continue to demand stronger, lighter, and more reliable pressure components, hydroforming technology will remain a critical solution for producing dished ends and other high-performance metal parts.
Overview of Hydroforming for Dished Ends
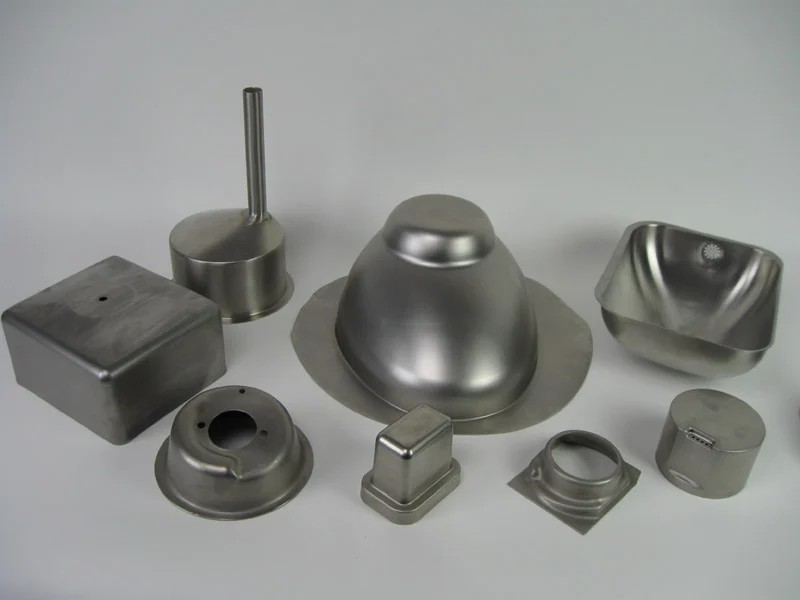
Hydroforming for dished ends is a specialized metal forming process that uses hydraulic pressure to shape metal blanks into dished heads or tank ends. Dished ends, also known as end caps or tank heads, are critical components used in various industries to close the ends of pressure vessels, tanks, boilers, pipelines, and storage containers. These components must be strong, seamless, and durable enough to withstand high pressures, extreme temperatures, and potentially corrosive environments.
The hydroforming process allows manufacturers to create seamless dished ends with complex geometries and uniform thickness, offering significant advantages over traditional forming methods such as stamping, spinning, or welding. In hydroforming, a metal blank is pressed into a concave or convex shape using high-pressure hydraulic fluid applied through a flexible diaphragm. The resulting parts are characterized by their strength, precision, and reliability, making hydroforming an ideal solution for applications that require high-performance metal components.
This article provides a comprehensive overview of hydroforming for dished ends, covering the fundamental principles of the process, the key steps involved, the types of dished ends produced, and the various industries that rely on these components. Additionally, it highlights the benefits and limitations of hydroforming technology, emphasizing its value in modern manufacturing.
1. What Are Dished Ends?
Dished ends are concave or convex metal components used to close the ends of cylindrical vessels, tanks, or pipes. These parts serve an important function in ensuring that the contents of the vessel are contained safely and securely, especially when the vessel is under pressure or exposed to high temperatures. Depending on the design, dished ends can be either shallow or deep, and they are often made from materials such as carbon steel, stainless steel, aluminum, or titanium.
Dished ends come in several common shapes, including:
- Hemispherical: A fully rounded, dome-shaped end that provides excellent strength and pressure resistance.
- Ellipsoidal (2:1): Slightly flattened, with a shape that combines strength with material efficiency.
- Torispherical: A combination of a spherical section and a flat edge, commonly used for medium-pressure applications.
- Flat Ends: Simple, flat closures used in low-pressure or storage applications.
The design and shape of the dished end depend on the specific requirements of the application, including the operating pressure, temperature, and the type of fluid or gas contained within the vessel.
2. Principles of Hydroforming for Dished Ends
Hydroforming is a metal forming process in which hydraulic pressure is used to shape metal into complex geometries. For dished ends, hydroforming offers distinct advantages over traditional forming methods such as stamping, spinning, or pressing. The process uses hydraulic fluid, applied through a flexible diaphragm, to press a metal blank into a forming die, shaping it into a dished end without the need for multiple stages or complex tooling.
2.1 Hydraulic Pressure
At the core of the hydroforming process is the application of hydraulic pressure to a flat metal blank. A flexible diaphragm filled with hydraulic fluid is pressed against the blank, forcing it to conform to the shape of a concave or convex die. The use of hydraulic pressure ensures uniform material deformation, reducing the likelihood of defects such as wrinkling, tearing, or thinning.
- Even Pressure Distribution: Unlike traditional stamping, where force is applied unevenly by a punch, hydroforming uses hydraulic pressure to apply force evenly across the surface of the blank. This leads to better material flow and more consistent part quality.
- High Precision: The process allows for the creation of parts with tight tolerances and complex geometries, making it ideal for producing dished ends that must meet stringent dimensional requirements.
2.2 Material Flow and Formability
During hydroforming, the metal blank undergoes plastic deformation, meaning it is permanently shaped into the form of the die without springback or significant residual stresses. The ability to control material flow during the process ensures that the metal is stretched evenly into the die cavity, minimizing localized thinning or material failure.
- High Formability: Hydroforming is especially effective for materials that are ductile and capable of being stretched without breaking, such as stainless steel, aluminum, and certain high-strength alloys.
- Complex Shapes: The process allows for the formation of deep-drawn shapes, such as dished ends with significant curvature or intricate features, without the need for multiple forming steps.
2.3 Single-Sided Tooling
A key advantage of hydroforming is that it typically requires only a single-sided die. Unlike traditional stamping, which uses both a male and female die to form the part, hydroforming uses a flexible diaphragm to press the blank into a rigid, single-sided die. This reduces tooling complexity and costs, making the process more efficient for large-scale production.
3. Hydroforming Process for Dished Ends: Step-by-Step
The hydroforming process for dished ends follows several distinct stages, each carefully controlled to ensure the accuracy and quality of the final part. Below is an overview of the key steps involved in forming dished ends using a hydroforming press.
3.1 Blank Preparation
The process begins with the selection and preparation of a metal blank. The blank is typically a circular sheet of metal cut to the appropriate size for the dished end being formed. The choice of material depends on the application, with common choices including stainless steel, carbon steel, and aluminum.
- Material Selection: The material must possess the necessary formability and strength to withstand the hydraulic pressures involved in hydroforming without tearing or thinning.
- Blank Size: The diameter and thickness of the blank are determined based on the final size of the dished end and the depth of the draw.
3.2 Loading and Clamping
Once the blank is prepared, it is placed into the hydroforming press over the die. The blank is secured in place using a clamping system to prevent it from shifting or wrinkling during the forming process.
- Clamping System: The clamping system must apply uniform pressure to hold the blank firmly in place, ensuring that it remains aligned with the die throughout the forming cycle.
3.3 Application of Hydraulic Pressure
Hydraulic fluid is pumped into the diaphragm (also known as a bladder), creating the pressure needed to shape the blank into the dished end. As the pressure increases, the diaphragm expands and pushes the blank against the die.
- Gradual Pressure Increase: To avoid sudden deformation or material failure, hydraulic pressure is applied gradually, allowing the metal to stretch and flow into the die cavity.
- Uniform Material Flow: The flexible diaphragm ensures that pressure is applied evenly across the entire surface of the blank, promoting consistent material flow and reducing the risk of defects such as thinning or tearing.
3.4 Shaping the Dished End
As pressure continues to build, the blank is drawn into the die, conforming to the concave or convex shape of the tooling. This stage of the process is critical for ensuring that the part takes on its final shape with uniform wall thickness and smooth curvature.
- Deep Drawing Capability: The hydroforming process allows for the production of deep-drawn shapes, such as hemispherical or ellipsoidal dished ends, in a single operation.
- Complex Geometries: Hydroforming can produce dished ends with complex features, such as non-standard radii or flanged edges, without the need for secondary operations.
3.5 Pressure Holding and Stabilization
Once the dished end has been fully formed, the hydraulic pressure is maintained for a short period to allow the part to stabilize. This helps to relieve any internal stresses that may have built up during the forming process, ensuring that the part retains its shape and dimensional accuracy.
- Pressure Holding: Holding pressure during this stage helps the material fully conform to the die, achieving precise tolerances and surface finishes.
3.6 Pressure Release and Part Removal
After the pressure holding phase, the hydraulic pressure is gradually released, and the diaphragm retracts. The clamping system is disengaged, and the finished dished end is removed from the die.
- Controlled Pressure Release: Gradual release of pressure ensures that the part does not experience springback or deformation after forming.
- Post-Processing: The dished end may undergo additional finishing processes, such as trimming, polishing, or heat treatment, depending on the application.
4. Types of Dished Ends Produced by Hydroforming
The hydroforming process can be used to produce a wide range of dished end types, each suited for different applications and pressure requirements. Below are some of the most common types of dished ends formed using hydroforming technology.
4.1 Hemispherical Dished Ends
Hemispherical dished ends are fully rounded, dome-shaped components that provide excellent strength and pressure resistance. These ends are commonly used in high-pressure applications, such as pressure vessels and boilers.
- Advantages: Hemispherical ends offer the highest strength-to-weight ratio, allowing vessels to withstand extreme pressures with minimal material thickness.
4.2 Ellipsoidal Dished Ends (2:1 Ratio)
Ellipsoidal dished ends, also known as 2:1 ellipsoidal heads, have a slightly flattened shape with a height equal to one-quarter of the vessel’s diameter. These ends are commonly used in pressure vessels where moderate pressure resistance is required.
- Advantages: Ellipsoidal ends provide a balance between material efficiency and strength, making them suitable for a variety of industrial applications.
4.3 Torispherical Dished Ends
Torispherical dished ends feature a rounded crown and a flat edge, creating a dish-like shape that is ideal for medium-pressure applications. These ends are often used in storage tanks and chemical processing equipment.
- Advantages: Torispherical heads are easier to manufacture and are more economical for medium-pressure applications compared to hemispherical ends.
4.4 Flat Dished Ends
Flat dished ends are simple, flat closures used to seal off the ends of storage tanks or low-pressure vessels. These are the least expensive and easiest to manufacture but are not suitable for high-pressure environments.
- Advantages: Flat ends are cost-effective and easy to produce, making them ideal for applications where pressure resistance is not a primary concern.
5. Industries and Applications of Hydroformed Dished Ends
Hydroforming technology for dished ends is widely used across multiple industries where high-performance pressure vessels and storage tanks are required. Below are some of the key industries that rely on hydroformed dished ends.
5.1 Oil and Gas Industry
In the oil and gas industry, dished ends are used in pressure vessels, refinery equipment, and pipelines to handle high-pressure fluids and gases. The seamless construction provided by hydroforming ensures that the vessels can withstand extreme pressures and corrosive environments.
- Pressure Vessels: Hydroformed dished ends are used in pressure vessels for storing and transporting oil, gas, and other fluids under high pressure.
5.2 Chemical Processing
The chemical industry relies on dished ends for reactors, storage tanks, and heat exchangers. These components must be able to withstand harsh chemicals, high temperatures, and pressures, making hydroformed dished ends an ideal choice.
- Chemical Reactors: Hydroformed dished ends are used in chemical reactors to ensure safe containment of reactive chemicals under high pressure and temperature.
5.3 Power Generation
In the power generation industry, dished ends are used in boilers, steam drums, and heat exchangers. These components must maintain their structural integrity under high-pressure steam and extreme heat.
- Boiler Ends: Hydroformed dished ends are used to seal off the ends of boilers, ensuring that they can withstand the high pressures and temperatures involved in steam generation.
5.4 Food and Beverage
In the food and beverage industry, dished ends are used in storage tanks and processing vessels for liquids and gases. The smooth, seamless surfaces provided by hydroforming make it easier to clean and sanitize the equipment, ensuring hygiene and safety.
- Storage Tanks: Hydroformed dished ends are used in storage tanks for beverages, dairy products, and other liquids that require sterile storage conditions.
5.5 Pharmaceuticals
The pharmaceutical industry uses dished ends in pressure vessels and reactors where sterility and contamination prevention are essential. Hydroforming produces seamless parts with smooth surfaces that meet the industry’s strict hygiene standards.
6. Advantages of Hydroforming for Dished Ends
Hydroforming offers numerous advantages over traditional forming methods for producing dished ends:
6.1 Seamless Construction
The seamless construction achieved through hydroforming eliminates the need for welding, reducing the risk of leaks and improving the overall strength of the part.
- Leak Prevention: Without welded seams, there is less risk of leaks in pressure vessels or tanks, making hydroformed dished ends ideal for high-pressure applications.
6.2 Uniform Thickness
Hydroforming ensures uniform wall thickness, which is critical for maintaining the strength and durability of pressure vessels. Even material distribution helps prevent weak spots that could lead to failure under pressure.
- Pressure Resistance: Uniform thickness improves the ability of the dished end to resist internal or external pressures without failure.
6.3 Material Efficiency
The hydroforming process minimizes material waste by forming the metal blank directly into the desired shape. This reduces the need for excessive trimming or cutting, making the process more economical.
- Cost Savings: By reducing material waste and tooling costs, hydroforming offers significant savings in large-scale production.
6.4 Complex Geometries
Hydroforming allows for the creation of complex shapes and deep geometries that are difficult or impossible to achieve using traditional forming methods. This includes deep-drawn shapes, flanged edges, and non-standard radii.
- Design Flexibility: Manufacturers have greater freedom in designing custom dished ends that meet specific application requirements.
7. Limitations of Hydroforming for Dished Ends
While hydroforming offers many advantages, it is not without its limitations:
7.1 Higher Equipment Costs
The initial investment in hydroforming equipment, particularly for large-scale operations, can be higher than traditional metal forming methods such as stamping or spinning.
- Capital Investment: The cost of hydraulic presses and associated tooling can be prohibitive for small-scale operations or low-volume production runs.
7.2 Limited Material Compatibility
Not all materials are suitable for hydroforming. Materials with low ductility or poor formability may crack or fail during the process, limiting the types of metals that can be used.
- Material Constraints: While hydroforming is effective for many metals, it may not be suitable for brittle or low-ductility materials that cannot withstand the stretching forces involved.
Conclusion
Hydroforming for dished ends is a highly efficient and versatile process that enables manufacturers to produce complex, seamless, and high-performance components used in pressure vessels, boilers, storage tanks, and industrial equipment. By utilizing hydraulic pressure to form metal blanks into precise shapes, hydroforming provides several advantages over traditional forming methods, including seamless construction, uniform wall thickness, material efficiency, and the ability to create complex geometries.
Industries such as oil and gas, chemical processing, power generation, and food and beverage rely heavily on hydroformed dished ends for their critical equipment, where strength, reliability, and safety are paramount. Despite its limitations in terms of material compatibility and equipment costs, the benefits of hydroforming for dished ends make it an essential technology in modern manufacturing. As industries continue to demand lighter, stronger, and more durable components, hydroforming will play a key role in shaping the future of high-performance metal parts.
Hydroforming Process for Dished Ends: A Comprehensive Guide
Hydroforming is a highly advanced metal forming process that uses hydraulic pressure to shape metal blanks into complex geometries. When applied to dished ends, which are concave or convex metal components used to close off the ends of cylindrical vessels, tanks, or pipes, hydroforming offers significant advantages over traditional forming methods like stamping, spinning, or pressing. This process is widely used to manufacture pressure vessel heads, boiler ends, storage tank caps, and end closures for pipelines, where strength, precision, and seamless construction are essential.
The hydroforming process for dished ends produces components with uniform wall thickness, seamless construction, and tight tolerances—all key factors for applications involving high pressure, extreme temperatures, or hazardous materials. The ability to form intricate shapes with minimal defects makes hydroforming an ideal solution for manufacturing dished ends used in critical industries such as oil and gas, chemical processing, power generation, and pharmaceuticals.
This article provides a detailed overview of the hydroforming process for dished ends, including each step of the process, the equipment used, the types of dished ends produced, and the advantages and limitations of hydroforming technology.
1. What Are Dished Ends?
Dished ends, also referred to as tank heads, end caps, or pressure vessel heads, are metal closures used to seal the ends of cylindrical containers such as pressure vessels, boilers, tanks, and pipelines. The shape of dished ends can vary, but they are generally either convex (bulging outward) or concave (curved inward), depending on the application.
Dished ends must be strong enough to handle internal pressures and durable enough to maintain structural integrity in extreme environments. The most common materials for dished ends include:
- Carbon steel: High strength and economical, commonly used in pressure vessels.
- Stainless steel: Corrosion-resistant and used in environments requiring high durability.
- Aluminum: Lightweight and resistant to corrosion, used in specific applications where weight is a concern.
The type of dished end used depends on the design requirements of the vessel and the operating conditions, including the pressure, temperature, and the type of fluid or gas contained within the vessel.
2. Hydroforming Principles for Dished Ends
Hydroforming involves using high-pressure hydraulic fluid to deform a metal blank into the desired shape. This is achieved by pressing the blank into a die using a flexible diaphragm filled with hydraulic fluid. The even distribution of pressure ensures smooth material flow and uniform thickness, resulting in a seamless, high-strength part.
The key principles behind the hydroforming process for dished ends include:
2.1 Hydraulic Pressure
The hydroforming process uses hydraulic pressure to form the metal blank into the desired shape. Hydraulic fluid is injected into the flexible diaphragm, which then presses against the blank, forcing it to conform to the shape of the die. The pressure applied during the process can range from 10,000 to 20,000 psi or more, depending on the material and the depth of the draw.
- Gradual Pressure Increase: To prevent defects like tearing or wrinkling, the hydraulic pressure is increased gradually, allowing the material to stretch and flow into the die smoothly.
- Even Pressure Distribution: The use of hydraulic fluid ensures that the pressure is applied evenly across the entire surface of the blank, reducing localized thinning and ensuring consistent wall thickness.
2.2 Material Flow and Stretching
The metal blank undergoes plastic deformation during hydroforming, meaning it is permanently reshaped into the form of the die. The flexibility of the diaphragm allows the material to flow naturally into the die cavity, reducing the risk of defects that are common in traditional forming processes.
- High Formability: Hydroforming is particularly effective for materials with good ductility and formability, such as stainless steel, aluminum, and titanium.
- Uniform Wall Thickness: The even pressure applied during hydroforming ensures that the material is stretched uniformly, resulting in consistent wall thickness across the entire part.
2.3 Single-Sided Tooling
Unlike traditional stamping or deep drawing, which requires both a punch and die to shape the part, hydroforming uses a single-sided die. The diaphragm replaces the punch, pressing the blank into the die with hydraulic pressure. This reduces tooling complexity and cost, making hydroforming more efficient for producing large, deep-drawn parts like dished ends.
3. Step-by-Step Hydroforming Process for Dished Ends
The hydroforming process for dished ends follows a series of carefully controlled steps to ensure that the metal blank is formed accurately and consistently into the desired shape. Below is a step-by-step breakdown of the key stages involved in producing dished ends using a hydroforming press.
3.1 Blank Preparation
The first step in the hydroforming process is the preparation of the metal blank. The blank is typically a circular sheet of metal, cut to the size and thickness required for the final dished end. The material used must be carefully selected based on the operating conditions, such as pressure, temperature, and the type of fluid or gas the vessel will contain.
- Material Selection: Common materials for dished ends include carbon steel, stainless steel, aluminum, and titanium. The material must have good formability and strength to withstand the high pressures involved in hydroforming.
- Blank Thickness: The thickness of the blank is determined by the required strength of the dished end and the depth of the draw. Thicker blanks are used for higher-pressure applications, while thinner blanks are used for less demanding conditions.
3.2 Loading and Clamping
Once the blank is prepared, it is positioned over the forming die in the hydroforming press. The die defines the final shape of the dished end, and the blank is clamped securely in place to prevent it from shifting or wrinkling during the forming process.
- Clamping System: The clamping system holds the blank firmly in place over the die, ensuring that the blank remains stationary as hydraulic pressure is applied.
- Blank Alignment: Proper alignment of the blank is critical to ensure that the part is formed symmetrically and with even material flow.
3.3 Application of Hydraulic Pressure
With the blank clamped securely in place, hydraulic pressure is applied through the flexible diaphragm. Hydraulic fluid is pumped into the diaphragm, gradually increasing the pressure and forcing the blank into the die.
- Gradual Pressure Increase: Hydraulic pressure is applied slowly to allow the metal to stretch and flow into the die without tearing or thinning.
- Even Pressure Distribution: The flexible diaphragm ensures that the hydraulic pressure is distributed evenly across the entire surface of the blank, preventing localized thinning or defects.
3.4 Forming the Dished End
As the hydraulic pressure continues to build, the metal blank is drawn into the die, conforming to its concave or convex shape. The blank undergoes plastic deformation, taking on the final geometry of the dished end.
- Deep Drawing Capability: Hydroforming is ideal for creating deep-drawn parts, such as hemispherical or ellipsoidal dished ends, which require a significant amount of material stretching.
- Complex Geometries: The process can produce dished ends with complex shapes, including flanged edges, non-standard radii, and custom curvatures.
3.5 Pressure Holding and Stabilization
Once the dished end has been fully formed, the hydraulic pressure is maintained for a brief period to allow the material to stabilize. This ensures that the part retains its shape and dimensional accuracy.
- Pressure Holding: Holding the hydraulic pressure for a short time allows the material to fully conform to the die, reducing internal stresses and ensuring precise tolerances.
- Dimensional Stability: By maintaining pressure during this stage, the press ensures that the dished end retains its final dimensions without springback or deformation.
3.6 Pressure Release and Part Removal
After the holding phase, the hydraulic pressure is gradually released, allowing the diaphragm to retract. The clamping system is disengaged, and the finished dished end is removed from the die.
- Controlled Pressure Release: The hydraulic pressure is released slowly to prevent sudden deformation or springback of the part.
- Part Removal: Once the pressure is fully released, the dished end is gently lifted from the die, either manually or using automated systems.
3.7 Post-Processing and Finishing
The dished end may undergo additional post-processing steps, such as trimming, deburring, or polishing, depending on the specific requirements of the application. In some cases, the part may also be subjected to heat treatment to improve its mechanical properties.
- Trimming: Excess material around the edges of the dished end is removed to achieve the desired final shape and dimensions.
- Surface Finishing: The surface of the dished end is polished or treated to improve its appearance, corrosion resistance, or wear properties.
4. Types of Dished Ends Produced by Hydroforming
Hydroforming can be used to produce a variety of dished end shapes, each suited for different applications and pressure requirements. Below are some of the most common types of dished ends that are formed using hydroforming technology.
4.1 Hemispherical Dished Ends
Hemispherical dished ends have a fully rounded, dome-shaped design that offers the highest strength-to-weight ratio. These ends are commonly used in high-pressure applications such as pressure vessels and boilers.
- Advantages: Hemispherical ends provide superior pressure resistance due to their uniform shape and lack of stress concentration points.
- Applications: Used in high-pressure environments, including chemical reactors, storage tanks, and steam drums.
4.2 Ellipsoidal Dished Ends (2:1 Ratio)
Ellipsoidal dished ends, also known as 2:1 ellipsoidal heads, have a slightly flattened shape compared to hemispherical ends. The height of the ellipsoidal head is one-quarter of the vessel’s diameter, making it an efficient design for medium-pressure applications.
- Advantages: These ends offer a balance between material efficiency and strength, making them suitable for a wide range of pressure vessel applications.
- Applications: Commonly used in pressure vessels for chemical processing, food and beverage storage, and oil and gas.
4.3 Torispherical Dished Ends
Torispherical dished ends have a rounded crown with a flat edge, creating a dish-like shape. These ends are typically used in low-to-medium pressure applications where moderate pressure resistance is required.
- Advantages: Torispherical heads are easier to manufacture and are more economical compared to hemispherical or ellipsoidal ends.
- Applications: Often used in storage tanks, heat exchangers, and low-pressure processing equipment.
4.4 Flat Dished Ends
Flat dished ends are simple, flat closures used to cap the ends of storage tanks or low-pressure vessels. These ends are the easiest to manufacture and are used in applications where pressure resistance is not a primary concern.
- Advantages: Flat ends are cost-effective and can be produced quickly, making them ideal for non-pressurized storage tanks.
- Applications: Commonly used in storage tanks for water, chemicals, and non-pressurized liquids.
5. Industries and Applications of Hydroformed Dished Ends
Hydroformed dished ends are essential components in several industries where pressure vessels, storage tanks, and boilers are used. Below are some of the key industries that rely on hydroformed dished ends.
5.1 Oil and Gas Industry
In the oil and gas industry, dished ends are used in pressure vessels, pipelines, and refinery equipment to handle high-pressure fluids and gases. The seamless construction and strength provided by hydroforming ensure that these components can withstand harsh environments and extreme pressures.
- Pressure Vessels: Hydroformed dished ends are used in pressure vessels for storing and transporting oil, gas, and other fluids under high pressure.
- Pipeline End Caps: Dished ends are commonly used as end caps for large pipelines, providing a secure seal and enhancing the structural integrity of the pipeline.
5.2 Chemical Processing
In the chemical industry, dished ends are used in reactors, storage tanks, and pressure vessels that handle hazardous chemicals under high pressure and temperature. The ability to produce seamless, corrosion-resistant parts makes hydroforming ideal for this industry.
- Chemical Reactors: Hydroformed dished ends are used in chemical reactors to contain reactive chemicals safely and maintain pressure integrity.
5.3 Power Generation
The power generation industry uses dished ends in boilers, steam drums, and heat exchangers. These components must be able to withstand high temperatures and pressures, making hydroformed dished ends the preferred choice for power plants.
- Boiler Ends: Hydroformed dished ends are used in high-pressure steam boilers to ensure safe containment of steam and hot water.
5.4 Food and Beverage
In the food and beverage industry, dished ends are used in storage tanks and processing vessels for liquids and gases. The smooth, seamless surfaces provided by hydroforming help maintain hygiene and prevent contamination.
- Storage Tanks: Hydroformed dished ends are used in storage tanks for beverages, dairy products, and other foodstuffs that require sterile storage conditions.
5.5 Pharmaceuticals
In the pharmaceutical industry, dished ends are used in pressure vessels and reactors where sterility and cleanliness are essential. Hydroforming produces smooth, seamless parts that meet the industry’s strict hygiene standards.
6. Advantages of Hydroforming for Dished Ends
Hydroforming offers several advantages over traditional methods of forming dished ends:
6.1 Seamless Construction
One of the most significant advantages of hydroforming is the production of seamless dished ends. Seamless construction eliminates the need for welding, which can introduce weak points or potential leak paths.
- Leak Prevention: Seamless construction reduces the risk of leaks in pressure vessels, pipelines, and storage tanks, making hydroformed dished ends ideal for high-pressure applications.
6.2 Uniform Wall Thickness
Hydroforming ensures uniform wall thickness, which is critical for maintaining the strength and durability of dished ends. This consistency in material thickness helps prevent weak spots that could lead to part failure under pressure.
- Improved Pressure Resistance: Uniform thickness helps distribute pressure evenly across the entire dished end, improving its ability to withstand internal or external pressure.
6.3 Complex Geometries
Hydroforming allows for the creation of complex geometries and deep-drawn shapes that are difficult or impossible to achieve with traditional forming methods. This includes producing dished ends with flanged edges, sharp curvatures, and customized shapes.
- Design Flexibility: Hydroforming gives manufacturers greater freedom in designing parts with intricate shapes and precise tolerances.
6.4 Reduced Material Waste
The hydroforming process minimizes material waste by forming the blank directly into the desired shape with minimal trimming or cutting. This leads to more efficient use of raw materials and lower production costs.
- Cost Efficiency: By reducing material waste and eliminating the need for complex tooling, hydroforming offers significant cost savings for high-volume production.
7. Limitations of Hydroforming for Dished Ends
While hydroforming offers many advantages, it also has some limitations:
7.1 Higher Equipment Costs
Hydroforming equipment, particularly for large-scale operations, can be more expensive than traditional forming methods like stamping or spinning. The high cost of hydraulic presses and tooling may make hydroforming less feasible for small-scale operations or low-volume production.
- Capital Investment: The initial investment required for hydroforming equipment can be a barrier for manufacturers with limited budgets or low production volumes.
7.2 Material Limitations
Not all materials are suitable for hydroforming. Some materials with low ductility or poor formability may crack or fail during the process, limiting the range of metals that can be used.
- Material Constraints: While hydroforming is effective for many metals, brittle materials or those with limited stretchability may not perform well in the process.
Conclusion
The hydroforming process for dished ends is a highly efficient and precise method of producing seamless, high-performance components used in a variety of industries, including oil and gas, chemical processing, power generation, food and beverage, and pharmaceuticals. By using hydraulic pressure to form metal blanks into complex shapes, hydroforming provides several advantages over traditional forming methods, including seamless construction, uniform wall thickness, and the ability to produce complex geometries.
The versatility and reliability of hydroformed dished ends make them ideal for applications where strength, durability, and leak prevention are critical. As industries continue to demand more efficient and cost-effective solutions for manufacturing high-performance components, hydroforming technology will remain a valuable tool for producing dished ends and other critical metal parts.
Components of a Hydroforming Press for Dished Ends
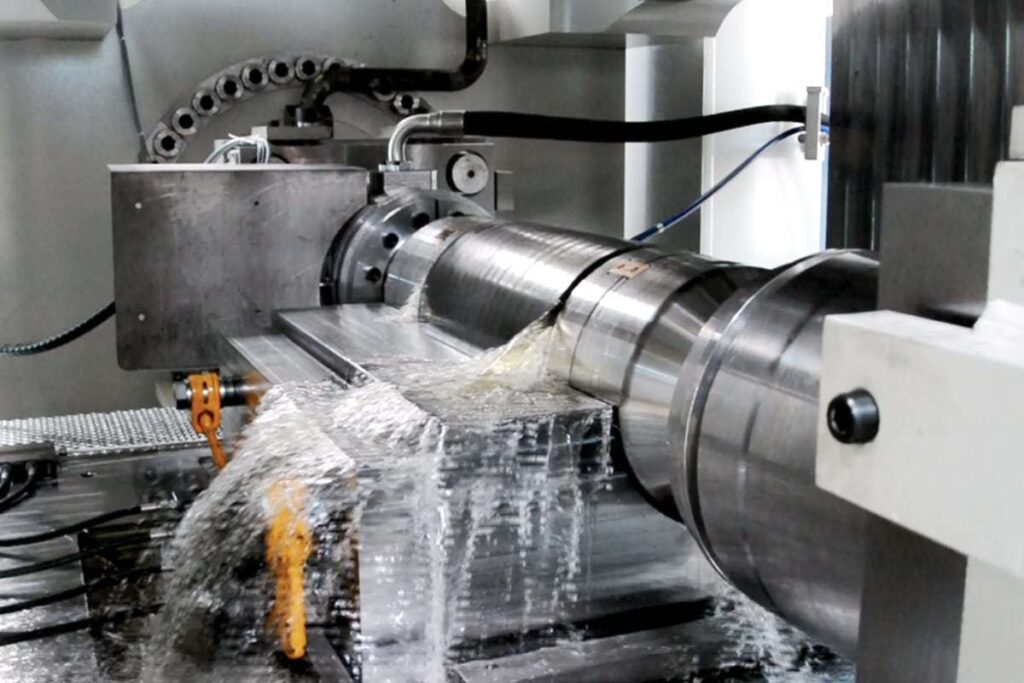
A hydroforming press designed for manufacturing dished ends—used in pressure vessels, tanks, boilers, and pipelines—requires specialized components to ensure that the process produces high-precision, seamless metal parts with consistent quality. These dished ends, also known as tank heads or end caps, must be able to withstand extreme pressures and temperatures, making their precise manufacture essential to their performance.
The hydroforming process uses hydraulic pressure to shape metal blanks into complex, curved geometries, and the hydroforming press is the primary machine responsible for carrying out this task. The press is made up of several critical components, each playing a vital role in the overall functionality of the process. Understanding these components, their functions, and how they interact with each other is key to ensuring successful operation and high-quality output in the production of dished ends.
This article provides a comprehensive overview of the key components of a hydroforming press for dished ends, explaining the purpose of each part, its role in the process, and how it contributes to the efficient production of precision metal parts.
1. Hydraulic System
At the core of any hydroforming press is the hydraulic system, which generates the high pressures necessary to form the metal blank into the desired shape. The hydraulic system includes pumps, valves, and reservoirs, all of which work together to pressurize hydraulic fluid and control its movement through the press.
1.1 Hydraulic Pump
The hydraulic pump is responsible for generating the hydraulic pressure that powers the diaphragm in the hydroforming press. The pump moves hydraulic fluid from the reservoir into the diaphragm, applying pressure to the metal blank. The amount of pressure generated by the pump is critical for forming large, thick dished ends and varies depending on the material being shaped.
- Types of Pumps: Pumps used in hydroforming can be axial piston pumps or variable displacement pumps, which are designed to deliver high-pressure hydraulic fluid. Pressures in hydroforming systems often exceed 10,000 psi or more, depending on the application.
- Precision Control: The hydraulic pump must provide precise control over the pressure applied during the forming process to prevent defects such as thinning, wrinkling, or tearing.
1.2 Hydraulic Valves
Hydraulic valves control the flow of hydraulic fluid within the system, regulating pressure and directing fluid to various parts of the press at specific stages of the process. Several different types of valves are used in a hydroforming press, including:
- Directional Control Valves: These valves determine the path of hydraulic fluid, allowing it to flow into or out of the diaphragm to build or release pressure.
- Pressure Relief Valves: Safety mechanisms that release hydraulic fluid if the pressure exceeds the safe operating limits of the system, preventing overpressure damage to the equipment or part.
- Flow Control Valves: These valves regulate the speed and volume of hydraulic fluid entering the diaphragm, ensuring smooth pressure application throughout the forming process.
1.3 Hydraulic Accumulator
The hydraulic accumulator stores pressurized fluid and releases it as needed to maintain steady pressure during the forming process. It acts as a reservoir that helps smooth out pressure fluctuations, ensuring that the system maintains consistent force on the metal blank.
- Pressure Stabilization: By holding extra hydraulic fluid, the accumulator ensures that pressure is consistently maintained, even as demand fluctuates during the forming process. This leads to more even material flow and improved part quality.
- Energy Storage: Accumulators also help store energy for rapid pressure changes, ensuring that the system responds quickly to changes in forming conditions.
2. Flexible Diaphragm (Bladder)
One of the defining components of a hydroforming press is the flexible diaphragm or bladder. This element is responsible for transmitting the hydraulic pressure generated by the system to the metal blank, shaping it into the form of the die.
2.1 Material of the Diaphragm
The diaphragm is made from flexible materials such as rubber, urethane, or other high-strength elastomers that can withstand repeated high-pressure cycles. The material must be capable of stretching and deforming without breaking, while also being durable enough to handle the friction and wear that occurs during the forming process.
- Durability: The diaphragm must withstand the high pressures involved in forming thick metal blanks (often exceeding 10,000 psi), as well as the repeated cycles of expansion and contraction during production.
- Flexibility: The material used must be highly elastic, ensuring that it can stretch evenly over the blank, apply uniform pressure, and retract without losing its shape.
2.2 Function of the Diaphragm
The diaphragm serves as the intermediary between the hydraulic fluid and the metal blank. When pressurized, it presses against the blank, forcing it into the die and shaping it into the desired form.
- Uniform Pressure Application: The flexibility of the diaphragm allows it to apply pressure evenly across the entire surface of the blank, ensuring consistent material deformation without localized thinning or tearing.
- Complex Shaping Capability: The diaphragm’s ability to conform to intricate die geometries allows for the production of complex dished ends with sharp radii, flanged edges, or deep draws.
3. Die (Tooling)
The die is the mold that defines the final shape of the dished end. In hydroforming, only a single-sided die is needed because the diaphragm replaces the upper punch used in traditional metal forming methods. The die is positioned in the lower part of the hydroforming press and determines the precise geometry of the dished end being formed.
3.1 Material of the Die
The die is typically made from hardened steel, tool steel, or cast iron, materials that are capable of withstanding the repeated pressures exerted by the hydraulic system without deforming or wearing out.
- Steel Dies: Commonly used for high-volume production, steel dies provide durability and the ability to withstand high-pressure cycles during long production runs.
- Aluminum Dies: In some cases, aluminum dies may be used for forming lighter materials or for small-batch production, though they are less durable than steel dies.
3.2 Die Design
The die must be custom-designed to match the specific shape and geometry of the dished end being produced. This includes ensuring that the die can accommodate the desired curvature, depth, and any additional features, such as flanged edges or customized radii.
- Geometrical Accuracy: The die’s design must be precise to ensure that the formed part has the exact dimensions and tolerances required for the application.
- Surface Finish: The surface of the die plays a role in determining the final surface finish of the dished end. A smooth die surface helps ensure a high-quality finish on the formed part, reducing the need for post-processing.
3.3 Die Holder and Support System
The die holder secures the die in place within the hydroforming press, ensuring that it remains properly aligned during the forming process. The support system ensures that the die does not shift under pressure, maintaining dimensional accuracy and preventing damage to the part or the equipment.
- Rigid Support: The die holder must provide strong, stable support for the die, preventing any movement that could lead to misalignment or defects in the formed part.
4. Clamping System
The clamping system is responsible for holding the metal blank securely in place over the die during the hydroforming process. Proper clamping is essential for preventing the blank from shifting, wrinkling, or becoming misaligned as pressure is applied.
4.1 Blank Holder
The blank holder or binder is the component that applies uniform pressure around the edges of the metal blank to keep it stationary during forming. This ensures that the blank does not wrinkle or fold as it is drawn into the die.
- Controlling Material Flow: The blank holder ensures that the metal flows smoothly into the die without excessive thinning or tearing, especially when forming deep, complex shapes.
- Preventing Wrinkling: Proper pressure from the blank holder prevents the edges of the blank from wrinkling, which is a common issue in traditional metal forming processes.
4.2 Sealing System
The sealing system ensures that the hydraulic pressure is contained within the diaphragm and does not leak during the forming process. A tight seal between the blank, the diaphragm, and the die is essential for maintaining pressure and ensuring a successful forming operation.
- Rubber Seals: High-strength rubber seals or other sealing materials are used to create a tight, leak-proof seal between the blank and the die, allowing pressure to build evenly without loss of hydraulic fluid.
5. Control System
Modern hydroforming presses are equipped with sophisticated control systems that allow operators to monitor and adjust various parameters of the forming process in real-time. These systems ensure precise control over pressure application, cycle timing, and other critical factors, resulting in higher-quality parts and increased production efficiency.
5.1 CNC (Computer Numerical Control)
CNC systems are used to automate the hydroforming process and control the movement of the diaphragm, the application of hydraulic pressure, and the timing of each stage of the cycle. CNC systems allow for greater precision and repeatability in part production.
- Multi-Axis Control: CNC systems can control the movement of the diaphragm and die along multiple axes, enabling the production of more complex geometries and tighter tolerances.
- Automated Pressure Control: The CNC system can adjust the rate at which hydraulic pressure is applied, ensuring that the pressure increases gradually and evenly across the blank.
5.2 PLC (Programmable Logic Controller)
A PLC system is another type of automation system used in hydroforming presses. It automates key functions such as pressure application, blank clamping, and part ejection, allowing for more efficient and consistent production cycles.
- Real-Time Monitoring: The PLC system monitors sensors throughout the press, ensuring that the machine operates within safe parameters and that each part is formed accurately.
- Process Sequencing: PLC systems can control the sequence of operations, ensuring that each stage of the hydroforming process occurs in the correct order.
6. Ejection System
Once the dished end has been formed, it must be safely removed from the die. The ejection system helps lift the part from the die without damaging its shape or surface.
6.1 Hydraulic Ejectors
Hydraulic ejectors are used to gently lift the formed part out of the die. These ejectors apply controlled pressure to remove the part smoothly without causing deformation or damage.
- Smooth Removal: Hydraulic ejectors ensure that the part is removed evenly, preventing warping or bending that could occur with manual removal.
6.2 Automated Ejection
In highly automated hydroforming systems, robotic arms or automated ejection systems may be used to remove the formed part and transport it to the next stage of production, such as trimming or finishing.
- Efficient Handling: Automated ejection systems reduce the risk of damage to the part and speed up production by minimizing manual handling.
7. Safety Systems
Given the high pressures involved in hydroforming, safety systems are critical to ensure the safe operation of the press and protect both the operator and the equipment.
7.1 Pressure Relief System
The pressure relief system is designed to release hydraulic pressure if it exceeds the safe operating limits of the system, preventing damage to the press or injury to the operator.
- Automatic Pressure Release: In the event of overpressure, the relief valves automatically release fluid to reduce pressure and prevent accidents.
7.2 Safety Guards and Interlocks
Most hydroforming presses are equipped with safety guards that prevent the operator from coming into contact with moving parts or high-pressure systems. These guards often include interlocks that prevent the press from operating unless the guards are securely in place.
- Operator Protection: Safety guards and interlocks protect the operator from potential hazards, such as moving machinery or sudden pressure releases.
Conclusion
A hydroforming press for dished ends is a highly specialized piece of equipment designed to form complex, high-strength components from metal sheets using hydraulic pressure. Each component of the press—from the hydraulic system and diaphragm to the die and control systems—plays a critical role in ensuring that the dished ends are formed accurately, consistently, and with minimal defects.
The hydraulic system provides the high pressure needed to shape the metal, while the diaphragm ensures that pressure is applied evenly across the blank. The die determines the shape of the part, and the clamping system prevents the blank from shifting during forming. Control systems ensure precision and repeatability, while ejection systems safely remove the part from the die.
As industries continue to demand high-performance, seamless components for pressure vessels, boilers, storage tanks, and pipelines, the hydroforming press will remain a critical tool in modern manufacturing. By combining precision, efficiency, and design flexibility, hydroforming technology enables manufacturers to produce dished ends that meet the most demanding standards for quality and performance.
Applications of Dished Ends
Dished ends, also known as tank heads or end caps, are critical components in the construction of pressure vessels, storage tanks, boilers, and other cylindrical containers used in a wide range of industries. These metal components are designed to cap or close off the ends of cylindrical vessels, providing structural integrity, maintaining pressure, and ensuring the safe containment of gases, liquids, and other materials. Dished ends are widely used in industries such as oil and gas, chemical processing, power generation, food and beverage, and pharmaceuticals.
Dished ends are available in a variety of shapes, including hemispherical, ellipsoidal, torispherical, and flat designs, each with unique advantages suited to specific applications. The choice of dished end design and material depends on factors such as operating pressure, temperature, and the nature of the substances contained within the vessel.
This article provides a comprehensive overview of the applications of dished ends across multiple industries, highlighting how these components contribute to the functionality, safety, and efficiency of pressure vessels and other containment systems.
1. Oil and Gas Industry
The oil and gas industry is one of the largest users of dished ends due to the extensive use of pressure vessels, storage tanks, pipelines, and refinery equipment. Dished ends play a critical role in ensuring the structural integrity of these vessels, which must withstand high pressures, extreme temperatures, and corrosive environments. In many cases, dished ends are used to cap the ends of cylindrical vessels that store or transport hydrocarbons and other hazardous substances.
1.1 Pressure Vessels
Pressure vessels in the oil and gas industry are used to contain gases and liquids under high pressure. These vessels require strong, durable dished ends to ensure the safe containment of their contents. Hemispherical and ellipsoidal dished ends are commonly used in pressure vessels due to their ability to withstand high internal pressures.
- Hemispherical Dished Ends: These provide the highest strength-to-weight ratio and are ideal for high-pressure vessels, such as those used in natural gas processing, oil refineries, and offshore drilling platforms. The fully rounded shape evenly distributes internal pressure, reducing stress points and enhancing safety.
- Ellipsoidal Dished Ends (2:1): These are also widely used in pressure vessels. Their flattened design offers a balance between strength and material efficiency, making them suitable for vessels operating under moderate pressures in oil and gas facilities.
1.2 Refinery Equipment
In oil refineries, dished ends are used in equipment such as reactors, distillation columns, and separators. These vessels must endure high temperatures, pressures, and the presence of corrosive chemicals, which makes the choice of dished ends and materials critical.
- Torispherical Dished Ends: These are commonly used in refinery equipment, where medium pressures are encountered. The combination of a rounded crown and a flat edge provides sufficient strength while being more economical than hemispherical designs.
- Stainless Steel and Alloy Materials: Due to the harsh operating conditions in refineries, dished ends are often made from stainless steel or nickel-based alloys that offer high corrosion resistance and the ability to withstand extreme temperatures.
1.3 Storage Tanks
Storage tanks in the oil and gas industry are used to hold large volumes of oil, gas, and other hydrocarbons. Dished ends provide a reliable way to close off these tanks while maintaining structural integrity, especially for pressurized tanks.
- Flat Dished Ends: These are commonly used for non-pressurized storage tanks that store oil, water, or other liquids. Flat ends are more cost-effective and provide a simple solution for sealing storage tanks in low-pressure applications.
- Ellipsoidal Dished Ends: For pressurized storage tanks, ellipsoidal ends are often chosen to handle the moderate pressures encountered during storage and transport.
1.4 Pipelines and Pipe Fittings
Dished ends are also used in pipelines and pipe fittings in the oil and gas sector, where they serve as end caps or closures that seal off the ends of pipelines, particularly at terminal points or junctions.
- Hemispherical End Caps: These are used at the ends of high-pressure pipelines to ensure that the pipeline remains sealed and can handle the high internal pressures associated with oil and gas transport.
- Corrosion-Resistant Materials: In pipelines that transport corrosive substances, dished ends made from materials like stainless steel or duplex steel are used to prevent material degradation and maintain pipeline integrity.
2. Chemical and Petrochemical Processing
In the chemical and petrochemical industries, dished ends are widely used in reactors, heat exchangers, storage tanks, and distillation columns. These vessels are subjected to high pressures, temperatures, and chemical exposure, making the strength and material selection of dished ends critical for safety and longevity.
2.1 Chemical Reactors
Chemical reactors are used to carry out chemical reactions under controlled conditions of temperature and pressure. Dished ends are used to seal these reactors, ensuring that the internal environment remains stable and that there is no leakage of hazardous chemicals.
- Ellipsoidal Dished Ends: These are commonly used in chemical reactors because they offer a good balance between pressure resistance and material efficiency. The ellipsoidal shape reduces the overall height of the vessel, which can be beneficial in compact reactor designs.
- Corrosion-Resistant Materials: Reactors handling highly reactive or corrosive chemicals often require dished ends made from materials like stainless steel, Hastelloy, or Inconel, which offer high resistance to chemical attack.
2.2 Heat Exchangers
In heat exchangers, dished ends are used to close off the ends of the shell or tubes where fluids are exchanged to transfer heat between two mediums. The dished ends must be capable of withstanding the pressures generated by the flow of fluids through the system, as well as the high temperatures involved in heat transfer processes.
- Torispherical Dished Ends: These are commonly used in heat exchangers due to their cost-effectiveness and ability to handle medium pressures. The combination of a spherical crown and a flat edge allows for efficient heat transfer and structural integrity.
- High-Temperature Materials: In heat exchangers operating at elevated temperatures, dished ends made from heat-resistant materials such as stainless steel or titanium are often used to prevent thermal degradation.
2.3 Distillation Columns
Distillation columns are critical components in chemical and petrochemical processing plants, where they are used to separate different chemical components based on their boiling points. Dished ends are used to seal the top and bottom of these columns, ensuring that the internal pressure and temperature are maintained during operation.
- Hemispherical and Ellipsoidal Dished Ends: These are often used in high-pressure distillation columns, as they can handle the high internal pressures and temperatures generated during the distillation process. The rounded shapes ensure even pressure distribution, reducing the risk of failure.
- Corrosion-Resistant Materials: Dished ends made from alloy steels or stainless steel are commonly used in distillation columns that handle corrosive substances or require long-term resistance to chemical attack.
3. Power Generation and Energy Sector
The power generation industry relies heavily on pressure vessels, boilers, and heat exchangers, all of which utilize dished ends to maintain pressure and structural integrity in high-temperature environments. These vessels play a crucial role in energy production processes, including steam generation, nuclear power, and renewable energy systems.
3.1 Steam Boilers
In steam boilers, dished ends are used to close off the ends of the boiler and maintain the pressure required to generate steam for power generation. These boilers operate at high temperatures and pressures, making it essential to use dished ends that can withstand the harsh conditions.
- Hemispherical Dished Ends: These are commonly used in high-pressure steam boilers because of their superior strength and ability to evenly distribute internal pressure. The hemispherical shape is ideal for preventing stress concentrations and ensuring long-term durability.
- High-Temperature Materials: Dished ends for steam boilers are often made from alloy steels or chromium-molybdenum steel, which can withstand the extreme temperatures and pressures generated during steam production.
3.2 Heat Exchangers in Power Plants
Heat exchangers are used in power plants to transfer heat between fluids, playing a critical role in processes such as steam condensation and waste heat recovery. Dished ends are used to seal the ends of these heat exchangers, ensuring that the fluids are contained and the heat transfer process remains efficient.
- Ellipsoidal and Torispherical Dished Ends: These are commonly used in heat exchangers operating under medium pressures. The dished ends must be capable of withstanding the pressure fluctuations that occur during the heat exchange process.
- Corrosion and Temperature Resistance: In power plants that use high-temperature fluids or corrosive gases, dished ends made from corrosion-resistant alloys are necessary to prevent material degradation and ensure reliable operation.
3.3 Nuclear Power Vessels
In nuclear power plants, dished ends are used in pressure vessels that contain nuclear reactors or other components of the power generation system. These vessels must be designed to withstand extreme pressures and radiation exposure, making the choice of dished ends critical to the safety and efficiency of the plant.
- Hemispherical and Ellipsoidal Dished Ends: Due to their ability to handle high internal pressures, hemispherical and ellipsoidal dished ends are commonly used in nuclear reactor pressure vessels. Their rounded shapes help distribute pressure evenly, reducing the risk of failure under extreme conditions.
- Radiation-Resistant Materials: Dished ends used in nuclear power plants are often made from radiation-resistant materials such as stainless steel or titanium, which offer durability and long-term stability in high-radiation environments.
4. Food and Beverage Industry
In the food and beverage industry, dished ends are widely used in storage tanks, processing vessels, and pressure cookers. These components must meet strict hygiene standards, as they come into direct contact with food and beverages. Dished ends in this industry are typically made from stainless steel or other food-grade materials to prevent contamination and ensure easy cleaning.
4.1 Storage Tanks
Storage tanks for liquids such as milk, beer, and wine often use dished ends to seal the ends of the tank while maintaining the cleanliness and sterility of the stored product. The dished ends must be made from materials that are easy to clean and resistant to corrosion.
- Ellipsoidal Dished Ends: These are commonly used in pressurized storage tanks for beverages, where they help maintain the internal pressure required for carbonation and preservation. The smooth surface finish ensures easy cleaning and sanitation.
- Stainless Steel: Stainless steel is the preferred material for dished ends in the food and beverage industry due to its corrosion resistance and ability to maintain hygiene standards.
4.2 Processing Vessels
In food processing plants, dished ends are used in vessels where food is cooked, mixed, or stored under pressure. These vessels must be able to withstand both high temperatures and pressure while ensuring that the food remains uncontaminated.
- Torispherical Dished Ends: These are often used in processing vessels due to their ability to handle medium pressures and temperatures. The rounded crown and flat edge provide the strength needed for pressurized cooking or mixing processes.
- Corrosion Resistance: Dished ends in food processing vessels are typically made from stainless steel or aluminum, which offer high resistance to corrosion and easy maintenance.
4.3 Pressure Cookers and Autoclaves
In commercial kitchens and industrial food processing facilities, pressure cookers and autoclaves use dished ends to maintain the pressure necessary for fast and efficient cooking. The dished ends help contain steam and heat, ensuring consistent cooking temperatures.
- Hemispherical Dished Ends: These are commonly used in high-pressure cooking vessels due to their strength and ability to maintain internal pressure without failure.
- Stainless Steel Construction: Pressure cookers and autoclaves often feature dished ends made from stainless steel, which offers excellent heat resistance and ease of cleaning.
5. Pharmaceutical and Biotech Industry
In the pharmaceutical and biotech industries, dished ends are used in pressure vessels, reactors, and storage tanks that must meet strict standards for cleanliness, sterility, and contamination prevention. These industries rely on dished ends to maintain the integrity of sensitive processes and ensure the safe storage of pharmaceutical ingredients and products.
5.1 Sterile Storage Tanks
Storage tanks used in the pharmaceutical industry are often used to store liquids, gases, or ingredients that must remain sterile and free from contamination. Dished ends help seal these tanks, ensuring that the contents remain uncontaminated during storage and handling.
- Ellipsoidal Dished Ends: These are commonly used in sterile storage tanks where moderate pressure is required to maintain the sterility of the contents. The smooth, seamless surface of the dished end ensures that there are no crevices where contaminants can accumulate.
- 316L Stainless Steel: Dished ends used in pharmaceutical storage tanks are often made from 316L stainless steel, which is highly resistant to corrosion and easy to clean, making it ideal for sterile environments.
5.2 Bioreactors
Bioreactors are used in the biotech industry to grow microorganisms or cells under controlled conditions. Dished ends are used to close off the ends of these reactors, ensuring that the internal environment remains stable and free from contaminants.
- Hemispherical and Ellipsoidal Dished Ends: These shapes are commonly used in bioreactors, where the smooth, rounded surfaces help prevent contamination and allow for easy cleaning. The dished ends must be capable of withstanding the pressures generated during fermentation or cell growth processes.
- Hygienic Design: Dished ends used in bioreactors are typically made from hygienic stainless steel with polished surfaces to meet strict cleanliness standards.
5.3 Pressure Vessels in Pharmaceutical Manufacturing
In pharmaceutical manufacturing, pressure vessels are used for mixing, sterilizing, and storing sensitive ingredients under controlled pressure. Dished ends are used to maintain the pressure and ensure the safe containment of pharmaceutical products.
- Torispherical and Ellipsoidal Dished Ends: These are commonly used in pharmaceutical pressure vessels due to their ability to handle moderate pressures while providing a smooth, cleanable surface.
- Corrosion-Resistant Materials: Dished ends in pharmaceutical pressure vessels are made from stainless steel or nickel-based alloys to prevent corrosion and ensure the longevity of the vessel.
Conclusion
Dished ends are an essential component in a wide range of industries, playing a critical role in ensuring the safety, functionality, and efficiency of pressure vessels, storage tanks, reactors, and heat exchangers. From high-pressure applications in the oil and gas and power generation industries to sterile environments in pharmaceutical and food processing sectors, dished ends offer the strength, durability, and precision needed to meet the demands of modern industrial processes.
The choice of dished end shape—whether hemispherical, ellipsoidal, torispherical, or flat—is determined by the specific application, with each design offering unique advantages in terms of strength, pressure resistance, and material efficiency. Additionally, the selection of materials, such as stainless steel, carbon steel, or specialized alloys, plays a crucial role in ensuring the long-term performance and reliability of the dished ends in demanding environments.
As industries continue to evolve and demand more efficient and high-performance components, the applications of dished ends will remain integral to the safe and reliable operation of pressure vessels, tanks, and containment systems across a wide range of sectors.
Hydroforming Steel: Process, Benefits, and Applications
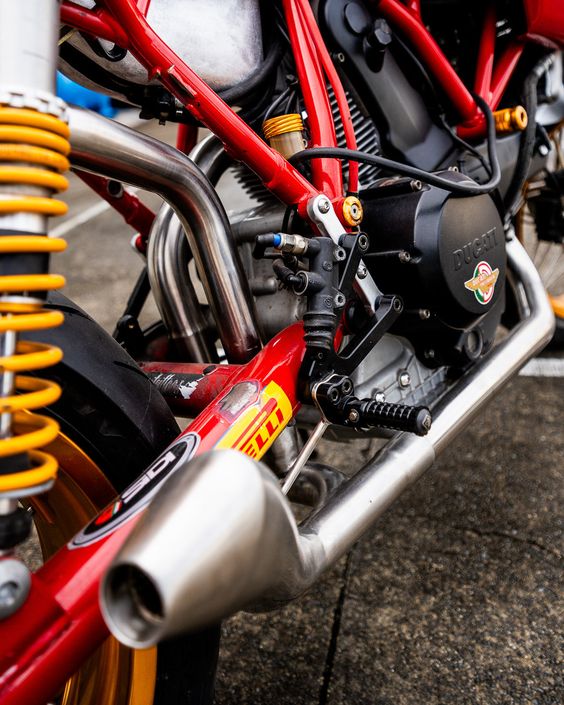
Hydroforming steel is a metal forming process that uses high-pressure hydraulic fluid to shape steel sheets or tubes into complex, high-strength components. This technique allows manufacturers to produce intricate geometries with high precision and minimal defects, making it ideal for applications in industries such as automotive, aerospace, construction, appliances, and energy. Hydroforming steel offers several key advantages over traditional stamping or pressing methods, including uniform material distribution, enhanced structural integrity, reduced material waste, and lower tooling costs.
Steel, being a highly versatile and strong material, is particularly well-suited for hydroforming processes. By using hydraulic pressure to form steel, manufacturers can create components with superior mechanical properties that meet the demands of modern engineering applications, including parts that are lightweight, durable, and capable of withstanding extreme loads or harsh environments.
This article provides an in-depth overview of hydroforming steel, exploring the process, its benefits, the types of steel used, and the applications where hydroformed steel parts are essential.
1. Overview of Steel Hydroforming
Hydroforming is a cold-forming process in which steel is shaped by applying high-pressure hydraulic fluid to either a steel sheet or a steel tube that is placed over a die. The pressure causes the steel to conform to the shape of the die, producing a component with precise dimensions and smooth surfaces. This process can be applied to a wide range of steel grades, depending on the mechanical properties and strength required for the final product.
Types of hydroforming processes used for steel:
- Sheet Hydroforming: A flat steel sheet is pressed into a die by hydraulic pressure from a flexible diaphragm, shaping the sheet into complex geometries with tight tolerances.
- Tube Hydroforming: A steel tube is expanded and pressed into the die using internal hydraulic pressure, forming it into the desired shape. This process is commonly used to create hollow structural components.
Steel grades commonly used in hydroforming include:
- Mild Steel (Low-Carbon Steel): Offers excellent formability and is commonly used for automotive body panels, frames, and appliances.
- High-Strength Low-Alloy (HSLA) Steel: Known for its high strength and good formability, HSLA steel is widely used in automotive and aerospace applications.
- Stainless Steel: Offers high corrosion resistance and is used for components that require both strength and environmental durability, such as exhaust systems, appliances, and structural components.
- Advanced High-Strength Steel (AHSS): Combines high strength with excellent energy absorption capabilities, making it ideal for safety-critical automotive components such as crash structures and reinforcement beams.
2. The Hydroforming Process for Steel
The hydroforming process for steel can be broken down into several key steps, depending on whether the process involves sheet or tube hydroforming. The process ensures uniform deformation and structural integrity in the final component.
2.1 Sheet Steel Hydroforming
In sheet hydroforming, a flat steel sheet is placed over a die in the hydroforming press. Hydraulic fluid is pumped into a flexible diaphragm, which presses the steel sheet against the die, shaping it into the desired form.
Process steps:
- Blank Preparation: A flat sheet of steel is cut to the appropriate size and thickness based on the final part’s dimensions.
- Clamping and Sealing: The steel blank is clamped securely over the die to prevent shifting or wrinkling during the forming process.
- Pressure Application: Hydraulic fluid is pumped into the diaphragm, which presses the steel sheet against the die. The pressure is applied gradually to avoid defects such as tearing or thinning.
- Part Removal: After the steel has conformed to the die, the hydraulic pressure is released, and the formed part is removed from the press.
2.2 Tube Steel Hydroforming
In tube hydroforming, a hollow steel tube is placed inside the die, and hydraulic fluid is pumped into the interior of the tube, causing it to expand and conform to the die’s shape.
Process steps:
- Tube Placement: The steel tube is placed inside the die and clamped securely at both ends.
- Internal Pressurization: Hydraulic fluid is pumped into the tube, causing the tube to expand and press against the die. This expands the tube’s diameter and reshapes it into the desired form.
- Axial Feeding: Additional force may be applied at the ends of the tube to help it stretch and conform to the die more accurately, especially for long or complex shapes.
- Part Removal: Once the desired shape is achieved, the hydraulic pressure is released, and the hydroformed steel tube is removed from the die.
3. Advantages of Hydroforming Steel
Hydroforming steel offers numerous advantages over traditional metal forming methods, particularly when creating components that require complex geometries, high precision, and strength. Below are some of the key benefits of hydroforming steel:
3.1 Enhanced Structural Integrity
The hydroforming process applies uniform hydraulic pressure across the entire steel sheet or tube, allowing the material to stretch and flow more evenly into the die. This uniform deformation results in components with superior structural integrity and consistent wall thickness, which are critical for parts that must withstand high loads, impact forces, or pressures.
- Improved Strength: By avoiding sharp bends and material thinning, hydroformed steel parts are less prone to fatigue and cracking, improving their overall durability and performance.
- Seamless Construction: Hydroforming eliminates the need for multiple parts or welds, creating seamless components that are stronger and less likely to fail under stress.
3.2 Complex Geometries and Design Flexibility
One of the key advantages of hydroforming is its ability to produce complex shapes with tight tolerances. Steel components formed through hydroforming can feature sharp angles, smooth curves, variable wall thicknesses, and deep recesses that would be difficult or impossible to achieve through stamping or traditional pressing methods.
- Integrated Features: Hydroforming allows manufacturers to integrate multiple design features, such as flanges, holes, and ribs, into a single part, reducing the need for secondary operations.
- 3D Geometries: Tube hydroforming, in particular, is ideal for creating hollow components with intricate 3D shapes, such as automotive chassis frames, exhaust components, and structural reinforcements.
3.3 Material Efficiency and Weight Reduction
Hydroforming maximizes material efficiency by reducing the need for excess trimming or cutting. The process directly shapes the steel blank or tube into the final component, minimizing waste and making better use of raw materials.
- Reduced Material Waste: By forming the component in a single step, hydroforming reduces material wastage compared to traditional methods that require multiple forming stages or additional trimming.
- Lightweighting: Hydroforming allows for the production of lightweight steel components with thin walls and complex shapes, making it an ideal solution for industries seeking to reduce the weight of their products without compromising strength or performance, such as the automotive and aerospace industries.
3.4 Lower Tooling Costs
Compared to traditional stamping or deep drawing processes, hydroforming uses single-sided tooling, which reduces the complexity and cost of tooling. Since only one die is required, manufacturers can reduce upfront investment in tooling while still achieving high precision and repeatability.
- Simplified Tooling: Hydroforming eliminates the need for separate upper and lower dies, simplifying the tooling process and reducing the overall cost of the operation.
- Versatility: The same hydroforming press can be used to produce a variety of different components by simply changing the die, offering greater flexibility and cost-effectiveness for manufacturers producing multiple part designs.
3.5 High-Quality Surface Finish
Hydroformed steel parts typically have a smooth, high-quality surface finish that requires minimal post-processing. The even application of hydraulic pressure reduces surface defects such as tool marks, scratches, or dents that are common in traditional metal forming methods.
- Reduced Finishing Requirements: The smooth surface finish produced by hydroforming reduces the need for secondary finishing operations, such as polishing or grinding, further lowering production costs and lead times.
4. Applications of Hydroformed Steel
Hydroforming steel components are used across a wide range of industries, particularly where strength, durability, and weight reduction are critical. Below are some of the key applications of hydroformed steel parts.
4.1 Automotive Industry
The automotive industry is one of the largest users of hydroformed steel components due to the process’s ability to produce lightweight, high-strength parts that improve vehicle performance, fuel efficiency, and safety.
- Chassis Components: Hydroformed steel chassis frames and subframes provide enhanced crash performance by absorbing impact forces more effectively. The seamless construction reduces the number of joints and welds, which are often weak points in traditional stamped or welded assemblies.
- Exhaust Systems: Hydroformed exhaust components such as manifolds and pipes offer smoother internal surfaces, improving airflow and reducing back pressure, which enhances engine performance and efficiency.
- Suspension Parts: Hydroformed steel is used to manufacture control arms, crossmembers, and strut towers, all of which require high strength and precision for optimal vehicle handling and stability.
- Crash Structures: Advanced high-strength steel (AHSS) is commonly hydroformed to create crash structures, such as side-impact beams and bumper reinforcements, that are designed to absorb energy during collisions and improve passenger safety.
4.2 Aerospace Industry
In the aerospace industry, hydroformed steel components are valued for their ability to withstand high loads and harsh environmental conditions while remaining lightweight. These parts are often used in structural components and systems that require both strength and precision.
- Aircraft Fuselage Components: Hydroformed steel parts such as ribs, spars, and bulkheads provide the structural integrity needed to support the aircraft’s frame while minimizing weight.
- Engine Components: Hydroforming is used to produce complex engine parts, such as turbine housings and exhaust ducts, that require high strength and precise geometries to withstand the extreme temperatures and pressures generated by aircraft engines.
- Landing Gear: Steel landing gear components are hydroformed to provide the necessary durability and impact resistance for repeated landings, while reducing the overall weight of the aircraft.
4.3 Construction Industry
In the construction industry, hydroformed steel components are used to create structural supports, beams, and pipes that require high strength and durability. Hydroforming allows construction companies to produce complex steel parts that meet the demands of modern architectural designs.
- Steel Beams and Supports: Hydroformed steel beams and columns provide the necessary strength for building construction while offering design flexibility for complex architectural structures.
- Piping Systems: Hydroformed steel pipes and fittings are used in structural applications, as well as in water and gas pipelines, due to their seamless construction and ability to withstand high internal pressures.
4.4 Appliances and Consumer Goods
Hydroforming steel is also widely used in the manufacture of appliances and consumer goods, where the process allows for the creation of durable, aesthetically pleasing components with smooth surfaces and complex designs.
- Household Appliances: Hydroformed steel components are used in refrigerator liners, oven panels, washing machine drums, and other household appliances that require high durability and corrosion resistance.
- Consumer Electronics: The strength and surface finish achieved through hydroforming make it ideal for producing enclosures, frames, and housings for electronics such as smartphones, laptops, and tablets.
4.5 Energy and Power Generation
In the energy industry, hydroformed steel components are used in pipelines, pressure vessels, turbines, and heat exchangers that operate under high pressures and temperatures.
- Heat Exchangers: Hydroformed steel tubes are commonly used in heat exchangers for power plants, where their seamless construction ensures efficient heat transfer and reduces the risk of leaks.
- Turbine Components: Hydroformed steel parts such as turbine blades and housings are used in gas and steam turbines, where they must withstand high mechanical loads and temperatures during operation.
- Pressure Vessels: Hydroformed steel components are used to create pressure vessels for storing gases and liquids under high pressure, such as in chemical processing plants, oil refineries, and energy facilities.
5. Limitations of Hydroforming Steel
While hydroforming offers many advantages, it is not without its limitations. Below are some challenges associated with hydroforming steel:
5.1 High Initial Investment
The initial cost of hydroforming equipment, particularly the hydraulic presses and specialized dies, can be high. This makes hydroforming more suitable for high-volume production where the investment can be offset by the reduction in material waste and tooling costs.
5.2 Limited Material Compatibility
Not all steel grades are suitable for hydroforming. Materials with low ductility or poor formability, such as certain high-carbon steels, may crack or fail during the process, limiting the range of steels that can be used.
5.3 Longer Cycle Times
Compared to traditional stamping or pressing methods, hydroforming can have longer cycle times, particularly for complex or large components. This may limit its efficiency for applications requiring very high production speeds.
Conclusion
Hydroforming steel is a highly efficient and versatile manufacturing process that allows manufacturers to create complex, high-strength components with superior structural integrity, material efficiency, and surface quality. By applying hydraulic pressure to steel sheets or tubes, hydroforming produces parts with uniform thickness, seamless construction, and intricate geometries, making it ideal for industries such as automotive, aerospace, construction, energy, and consumer goods.
The process’s ability to form lightweight yet durable components contributes to improved product performance, reduced material waste, and lower overall production costs. Despite some limitations, such as high initial investment and longer cycle times, hydroforming remains a critical technology for modern manufacturing, offering design flexibility and performance improvements in a wide range of applications. As technology continues to evolve, hydroforming steel will play an increasingly important role in the future of advanced manufacturing.
Types of Hydroforming Processes Used for Steel
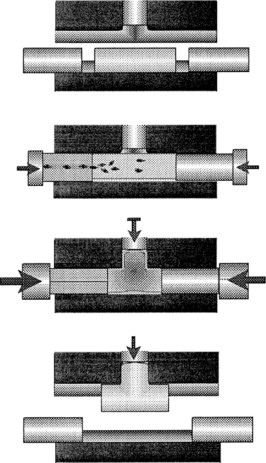
Hydroforming is a versatile and efficient metal forming process that uses high-pressure hydraulic fluid to shape steel and other metals into complex, high-precision components. By applying hydraulic pressure, hydroforming can transform steel sheets or tubes into lightweight, seamless, and structurally robust parts with minimal defects and high accuracy. This capability is particularly valuable in industries such as automotive, aerospace, construction, appliances, and energy, where components need to be durable, lightweight, and complex.
Several hydroforming processes are used to form steel, each tailored to specific types of parts and applications. These processes are generally classified based on whether they form steel sheets or steel tubes, but variations in pressure application, material movement, and forming conditions give rise to distinct techniques. Understanding the different types of hydroforming processes helps manufacturers choose the most suitable method for their applications, ensuring optimal part quality, performance, and cost-efficiency.
This article provides an in-depth overview of the main types of hydroforming processes used for steel, including sheet hydroforming, tube hydroforming, and specialized techniques such as high-pressure hydroforming, low-pressure hydroforming, and explosive hydroforming. It explores how each process works, its applications, and the benefits it offers for steel component manufacturing.
1. Overview of Hydroforming for Steel
Hydroforming is a cold-forming process in which hydraulic pressure is applied to steel sheets or tubes to form them into the desired shape. The hydraulic fluid is typically pressurized inside a flexible diaphragm or directly inside the steel tube, causing the steel to conform to the shape of a die. The process creates parts with high strength, uniform wall thickness, precise geometries, and smooth surfaces.
Types of steel used in hydroforming include:
- Low-carbon steel (mild steel): Known for its excellent formability and wide use in automotive body panels and appliance components.
- High-strength low-alloy (HSLA) steel: Combines strength and ductility, ideal for structural components in the automotive and aerospace industries.
- Stainless steel: Offers corrosion resistance, commonly used in exhaust systems, kitchen appliances, and other applications requiring environmental durability.
- Advanced high-strength steel (AHSS): Provides superior strength-to-weight ratios, often used for safety-critical automotive components and lightweighting efforts.
Each hydroforming process has distinct advantages depending on the type of steel being used and the desired final part characteristics.
2. Sheet Hydroforming
Sheet hydroforming is a metal forming process used to shape flat steel sheets into complex 3D geometries by pressing the sheet into a die using hydraulic pressure. The process is especially well-suited for producing parts with deep draws, complex curves, and uniform thickness, which are difficult to achieve with traditional stamping or pressing methods.
2.1 Types of Sheet Hydroforming Processes
There are two primary types of sheet hydroforming:
- Hydro-mechanical deep drawing: In this process, a punch mechanically presses the steel sheet into the die, while hydraulic fluid applies back pressure through a diaphragm to help control the flow of the material and prevent wrinkling. This method combines the benefits of mechanical deep drawing and hydroforming, making it ideal for producing deep, complex shapes with uniform wall thickness.
- Diaphragm sheet hydroforming: In this technique, the steel sheet is placed over a die, and hydraulic fluid inside a flexible diaphragm presses the sheet against the die. The pressure is applied evenly across the sheet, resulting in precise shapes without the need for a punch.
2.2 Process Steps
The basic steps in sheet hydroforming are as follows:
- Blank preparation: A steel blank (flat sheet) is cut to the required size and placed over the die.
- Clamping: The blank is clamped securely to prevent movement during the forming process.
- Hydraulic pressure application: Hydraulic fluid is pumped into a flexible diaphragm, pressing the sheet into the die. In hydro-mechanical deep drawing, a punch may assist the hydraulic pressure to form deeper or more complex parts.
- Forming: The steel sheet is formed into the die, taking on the final shape.
- Pressure release and part removal: Once the part is formed, the hydraulic pressure is released, and the part is removed from the die.
2.3 Applications of Sheet Hydroforming
Sheet hydroforming is used to produce a wide range of steel components, including:
- Automotive body panels: Lightweight steel parts such as hoods, roofs, doors, and fenders are commonly formed using sheet hydroforming, which allows for smooth surfaces and tight tolerances.
- Aircraft skin panels: In aerospace applications, sheet hydroforming is used to create fuselage panels, wing skins, and other structural parts that require high precision and strength.
- Consumer appliances: Refrigerator panels, oven liners, and washing machine drums are often manufactured using sheet hydroforming, which provides consistent surface finishes and precise dimensions.
2.4 Advantages of Sheet Hydroforming
- Complex geometries: Sheet hydroforming allows for the creation of complex, 3D shapes that would be difficult to achieve with traditional metal forming processes.
- Uniform wall thickness: The process ensures even material distribution, minimizing thinning in critical areas.
- High-quality surface finishes: Hydraulic pressure is applied smoothly, resulting in parts with minimal surface defects and reduced need for secondary finishing.
- Reduced tooling costs: Unlike traditional stamping, which requires matched dies (upper and lower), sheet hydroforming typically uses only a single-sided die, reducing tooling costs.
3. Tube Hydroforming
Tube hydroforming is a process used to shape hollow steel tubes by expanding and pressing them into a die using internal hydraulic pressure. The technique is especially useful for producing lightweight, structurally robust components with complex shapes, variable wall thicknesses, and integrated features.
3.1 High-Pressure Tube Hydroforming
High-pressure tube hydroforming is the most common form of tube hydroforming, in which high internal hydraulic pressure is used to expand a steel tube into the shape of a die. The internal pressure can reach up to 10,000–20,000 psi or more, depending on the steel grade and the complexity of the part being formed.
Process steps:
- Tube placement: A hollow steel tube is placed inside a closed die.
- Clamping and sealing: The tube is clamped securely at both ends to prevent movement or leakage of hydraulic fluid.
- Pressurization: Hydraulic fluid is pumped into the tube, expanding it against the walls of the die. Additional axial force may be applied to the ends of the tube to help it conform to the die shape, especially in cases where long, complex parts are being formed.
- Part removal: After the part has fully conformed to the die, the hydraulic pressure is released, and the hydroformed part is removed from the die.
3.2 Low-Pressure Tube Hydroforming
Low-pressure tube hydroforming uses lower hydraulic pressures to shape steel tubes into the desired form. Instead of expanding the tube to fill the entire die cavity, this process is used to gently shape the tube, often in combination with mechanical forming.
Process characteristics:
- Lower pressures are used to minimize material strain, making it ideal for applications where precision and minimal material thinning are required.
- Axial feed is used more extensively in low-pressure hydroforming, allowing the tube material to flow more easily into the die.
3.3 Applications of Tube Hydroforming
Tube hydroforming is widely used in the automotive, aerospace, and construction industries, as well as for producing sports equipment and appliances.
- Automotive chassis and frames: Tube hydroforming is commonly used to produce automotive chassis components, such as frame rails, engine cradles, and suspension parts. These components are lightweight yet structurally strong, contributing to vehicle safety and fuel efficiency.
- Exhaust systems: Automotive exhaust components, including exhaust manifolds and pipes, are often manufactured using tube hydroforming. The process ensures smooth internal surfaces that improve exhaust flow and reduce emissions.
- Bicycle frames: Lightweight, hydroformed steel tubes are used to create high-performance bicycle frames with enhanced strength and durability, offering cyclists better handling and reduced weight.
3.4 Advantages of Tube Hydroforming
- Complex hollow structures: Tube hydroforming is ideal for creating hollow steel components with intricate shapes and integrated features, such as flanges, ribs, and brackets, in a single operation.
- Weight reduction: By precisely controlling material flow and wall thickness, tube hydroforming produces lightweight steel components without compromising strength.
- Fewer assembly operations: Tube hydroforming reduces the need for welding and joining separate components, improving part strength and reducing production time.
- High strength-to-weight ratio: Tube hydroforming produces parts with superior strength and durability, making them ideal for safety-critical applications in the automotive and aerospace sectors.
4. High-Pressure Hydroforming
High-pressure hydroforming is a technique used to shape steel components by applying extremely high hydraulic pressures, typically exceeding 10,000 psi, to force the material into complex shapes. This process is most commonly used for tube hydroforming, where the internal pressure inside the tube causes the material to expand and conform to the die.
4.1 Process Characteristics
- Extremely high pressures allow for the formation of intricate shapes with sharp angles, tight radii, and variable wall thicknesses.
- Axial feeding: In high-pressure hydroforming, axial force is often applied to the ends of the steel tube to help it flow into the die and maintain uniform wall thickness, especially for long parts.
- Precision control: The application of high hydraulic pressures requires precise control to avoid tearing or thinning of the steel.
4.2 Applications of High-Pressure Hydroforming
High-pressure hydroforming is widely used in industries where lightweight, high-strength steel components are required, such as the automotive and aerospace industries.
- Automotive crash structures: High-pressure hydroforming is used to produce crash-resistant components, such as side-impact beams and bumper reinforcements, which are designed to absorb impact forces during collisions.
- Aircraft structural components: High-pressure hydroformed steel parts, such as fuselage frames, are used in aircraft to reduce weight while maintaining the necessary strength to withstand extreme loads during flight.
5. Low-Pressure Hydroforming
Low-pressure hydroforming is a technique in which lower hydraulic pressures, typically under 5,000 psi, are used to form steel components. This process is often used when shaping large, shallow parts or when the material does not need to expand as dramatically as in high-pressure hydroforming.
5.1 Process Characteristics
- Lower pressures are used to prevent excessive stretching or thinning of the steel, making it ideal for parts that require more precise control of wall thickness.
- Combined with mechanical force: In some cases, low-pressure hydroforming may be combined with mechanical punches or presses to help guide the material into the desired shape.
5.2 Applications of Low-Pressure Hydroforming
Low-pressure hydroforming is commonly used for parts that require minimal material deformation and consistent wall thickness, such as:
- Automotive body panels: Large steel body panels for vehicles, such as hoods and doors, are often hydroformed using lower pressures to ensure smooth surfaces and precise geometries.
- Appliance components: Low-pressure hydroforming is used to produce parts like refrigerator panels, oven doors, and washing machine drums, where the final part must maintain a consistent wall thickness.
6. Explosive Hydroforming
Explosive hydroforming is a specialized technique that uses the energy from a controlled explosion to create extremely high pressures that shape steel components. The shockwave generated by the explosion replaces the hydraulic fluid used in traditional hydroforming processes, applying pressure to the steel to form it into the die.
6.1 Process Characteristics
- Explosive charges are detonated in a water-filled chamber, creating a shockwave that forces the steel blank or tube into the die.
- Extremely high pressures: Explosive hydroforming can generate pressures that far exceed traditional hydraulic systems, allowing for the formation of very large or thick steel components.
- Specialized applications: Due to the complexity and safety requirements of the process, explosive hydroforming is generally reserved for large-scale, high-performance applications where other methods would be impractical.
6.2 Applications of Explosive Hydroforming
Explosive hydroforming is primarily used in industries that require large, thick steel components or parts with extreme strength requirements, such as the aerospace and defense sectors.
- Aircraft structural components: Explosive hydroforming is used to create large, high-strength steel parts for aircraft fuselages and landing gear.
- Defense applications: The process is used to form steel parts for military vehicles, naval ships, and armored components that need to withstand extreme impacts and loads.
Conclusion
Hydroforming processes used for steel, including sheet hydroforming, tube hydroforming, high-pressure hydroforming, low-pressure hydroforming, and explosive hydroforming, offer a wide range of capabilities for producing lightweight, strong, and complex steel components. Each process has its own advantages and is suited for specific applications, from creating automotive body panels and aircraft structural components to manufacturing appliance parts and pressure vessels.
The ability to form steel into intricate shapes with high precision, uniform wall thickness, and seamless construction makes hydroforming a critical technology in modern manufacturing. Whether used to produce hollow structural components, lightweight chassis frames, or deep-drawn panels, hydroforming enables manufacturers to meet the growing demands for performance, efficiency, and sustainability in industries such as automotive, aerospace, construction, energy, and consumer goods. As technology advances, hydroforming processes will continue to play a pivotal role in the development of next-generation steel components.
The Hydroforming Process for Steel
Hydroforming is a highly advanced and efficient method of shaping metal, particularly steel, into complex, high-strength components. The process uses high-pressure hydraulic fluid to shape steel sheets or tubes into desired geometries by pressing them into a die. Compared to traditional metal forming techniques like stamping or pressing, hydroforming offers numerous advantages, including improved structural integrity, weight reduction, cost efficiency, and the ability to form parts with complex geometries and uniform wall thickness. The versatility of the hydroforming process makes it widely applicable across industries such as automotive, aerospace, construction, and appliances.
The hydroforming process for steel can be divided into two major categories: sheet hydroforming and tube hydroforming. Each category utilizes hydraulic pressure in different ways to shape the steel, and both processes offer unique benefits depending on the specific application.
This article provides an in-depth overview of the hydroforming process for steel, discussing the basic principles, the steps involved in the process, the types of hydroforming, and the advantages of this technology. It also covers the types of steel used in hydroforming and the industries that benefit from its application.
1. Overview of the Hydroforming Process
Hydroforming is a cold-forming process that involves shaping steel by applying hydraulic pressure through a flexible diaphragm or directly inside a steel tube. The process can create lightweight, strong, and seamless steel components with minimal defects and high precision. The hydroforming press plays a crucial role in applying the hydraulic pressure needed to shape the steel, while the die determines the final shape of the component.
There are two primary forms of hydroforming for steel:
- Sheet hydroforming: This process shapes flat sheets of steel by pressing them into a die using hydraulic pressure.
- Tube hydroforming: In this process, a hollow steel tube is expanded and shaped by internal hydraulic pressure to form complex, hollow parts.
Hydroforming is especially valuable for creating parts with complex geometries, sharp contours, and variable wall thicknesses, which are difficult to achieve with traditional stamping or pressing methods.
1.1 Key Characteristics of Hydroforming for Steel
- High strength-to-weight ratio: The process produces lightweight steel parts with excellent structural integrity, making it ideal for industries focused on weight reduction, such as automotive and aerospace.
- Uniform material distribution: Hydroforming ensures even distribution of material across the part, minimizing thinning and improving the overall strength and durability of the component.
- Seamless construction: Components produced through hydroforming often have a seamless design, reducing the need for welding or assembly, which enhances part performance and reliability.
2. The Hydroforming Process: Step-by-Step
The specific steps involved in the hydroforming process for steel vary slightly depending on whether the process involves sheets or tubes. Below are the general steps common to both types of hydroforming.
2.1 Blank Preparation
The first step in the hydroforming process is the preparation of the steel blank or steel tube. The blank is typically a flat steel sheet, while the tube is a hollow cylindrical steel piece. The size and thickness of the blank or tube are determined by the desired final part dimensions.
- Material selection: Depending on the application, different types of steel are selected for the hydroforming process, including low-carbon steel, high-strength low-alloy (HSLA) steel, stainless steel, or advanced high-strength steel (AHSS).
- Cutting: The steel blank or tube is cut to the appropriate size and shape to fit the hydroforming press and die.
2.2 Loading and Clamping
Once the steel blank or tube is prepared, it is placed in the hydroforming press and positioned over or inside the die. The blank or tube is then clamped securely to prevent movement during the forming process.
- Clamping system: The clamping system ensures that the blank or tube remains stationary as the hydraulic pressure is applied. This prevents wrinkling, misalignment, or shifting, which could compromise the final part’s quality.
2.3 Application of Hydraulic Pressure
The core of the hydroforming process involves applying hydraulic pressure to the steel blank or tube. This pressure forces the steel to conform to the shape of the die, resulting in the desired final geometry.
- Hydraulic fluid: Hydraulic fluid is pumped into a flexible diaphragm (for sheet hydroforming) or directly into the steel tube (for tube hydroforming) to generate the pressure needed for forming. In sheet hydroforming, the diaphragm presses the blank into the die. In tube hydroforming, the fluid expands the tube against the die walls.
- Gradual pressure increase: The pressure is applied gradually to prevent defects such as thinning, tearing, or wrinkling. This gradual application of pressure allows the steel to stretch and flow evenly into the die cavity.
2.4 Forming the Part
As hydraulic pressure builds, the steel blank or tube undergoes plastic deformation, permanently taking on the shape of the die. This step is critical for creating the desired part geometry, including complex curves, deep draws, and intricate contours.
- Material flow control: The hydroforming process ensures even material flow, minimizing localized thinning or defects that are common in traditional metal forming methods.
- Axial force (tube hydroforming): In tube hydroforming, axial force may be applied at the ends of the tube to help it stretch into the die more easily, especially for longer or more complex parts.
2.5 Pressure Holding and Release
Once the part has been fully formed, the hydraulic pressure is held for a short period to allow the material to stabilize. This ensures that the steel component retains its shape and dimensional accuracy.
- Pressure holding: Maintaining pressure for a brief period helps to relieve any internal stresses in the material and ensures the part fully conforms to the die.
- Controlled pressure release: The hydraulic pressure is gradually released, and the flexible diaphragm (in sheet hydroforming) retracts, or the tube (in tube hydroforming) deflates, allowing the part to be safely removed from the die.
2.6 Part Removal and Finishing
The final step involves removing the formed steel part from the die and inspecting it for any defects. Depending on the application, the part may undergo additional finishing processes such as trimming, deburring, or polishing.
- Trimming and deburring: Excess material may be trimmed away from the edges, and any rough edges or burrs are smoothed to meet precise specifications.
- Surface finishing: If required, the surface of the part can be polished, treated, or coated to improve corrosion resistance or aesthetic appearance.
3. Types of Hydroforming for Steel
There are two primary types of hydroforming used for steel: sheet hydroforming and tube hydroforming. Both processes have unique characteristics and are suited to different types of parts and applications.
3.1 Sheet Hydroforming
Sheet hydroforming is used to form flat steel sheets into complex 3D shapes by pressing the sheet into a die using hydraulic pressure. This process is ideal for producing large, thin-walled parts with intricate geometries.
- Diaphragm hydroforming: In this method, the steel sheet is pressed into the die by a flexible diaphragm filled with hydraulic fluid. This technique is commonly used for shallow, complex parts with smooth contours.
- Hydro-mechanical forming: In this process, a mechanical punch is used to press the sheet into the die, while hydraulic pressure applies back pressure to control material flow and prevent wrinkling.
Applications of sheet hydroforming include:
- Automotive body panels: Hoods, roofs, doors, and fenders are commonly made using sheet hydroforming.
- Aerospace panels: Lightweight steel parts such as fuselage skins and wing sections are formed using this method.
- Appliances: Parts like refrigerator panels and washing machine drums are often hydroformed to achieve precise shapes and smooth surfaces.
3.2 Tube Hydroforming
Tube hydroforming is used to shape hollow steel tubes by expanding them under internal hydraulic pressure. The tube is placed inside a die, and the hydraulic fluid is pumped into the tube, causing it to expand and conform to the die’s shape.
- High-pressure tube hydroforming: This method uses high internal hydraulic pressures to expand the tube against the die. It is commonly used for producing complex, high-strength hollow parts, such as automotive frames and chassis components.
- Low-pressure tube hydroforming: This method uses lower hydraulic pressures combined with mechanical forming to shape the tube. It is typically used for shallow, less complex parts.
Applications of tube hydroforming include:
- Automotive chassis components: Frame rails, subframes, and suspension parts are commonly made using tube hydroforming.
- Exhaust systems: Automotive exhaust manifolds and pipes are hydroformed to improve airflow and reduce emissions.
- Bicycle frames: Lightweight, durable steel tubes are hydroformed to create high-performance bicycle frames.
4. Types of Steel Used in Hydroforming
Different grades of steel are used in hydroforming depending on the mechanical properties and strength required for the final component. The choice of steel affects the formability, strength, and durability of the hydroformed part.
4.1 Low-Carbon Steel (Mild Steel)
Low-carbon steel, or mild steel, is highly formable and widely used in hydroforming. Its good ductility and malleability make it ideal for automotive body panels, appliances, and consumer goods.
4.2 High-Strength Low-Alloy (HSLA) Steel
HSLA steel combines high strength with good formability, making it ideal for structural components that require durability and weight savings. HSLA steel is commonly used in automotive frames and aerospace structures.
4.3 Stainless Steel
Stainless steel offers excellent corrosion resistance and is used in applications where environmental durability is important. Common applications include automotive exhaust systems, kitchen appliances, and structural components.
4.4 Advanced High-Strength Steel (AHSS)
AHSS provides superior strength-to-weight ratios, making it ideal for safety-critical components in automotive applications, such as side-impact beams and crash structures. AHSS allows manufacturers to create lightweight parts without compromising safety.
5. Advantages of Hydroforming for Steel
Hydroforming steel offers several significant advantages over traditional metal forming processes, making it a popular choice in industries that require lightweight, high-performance components.
5.1 Complex Geometries
Hydroforming allows for the creation of complex 3D shapes with tight tolerances, sharp angles, and intricate contours. This design flexibility is particularly beneficial for automotive and aerospace parts.
5.2 Lightweighting and Material Efficiency
Hydroforming allows manufacturers to produce lightweight steel components by controlling material thickness and reducing excess material waste. This contributes to improved fuel efficiency and performance, particularly in the automotive and aerospace industries.
5.3 High Structural Integrity
Hydroformed steel parts have uniform wall thickness and are typically seamless, resulting in high-strength components that are less prone to failure under stress.
5.4 Reduced Tooling Costs
Compared to traditional stamping, hydroforming typically requires only single-sided tooling, which reduces tooling costs and setup times, especially for complex parts.
5.5 Improved Surface Finish
The even application of hydraulic pressure during hydroforming results in parts with smooth surfaces and minimal defects, reducing the need for secondary finishing operations.
6. Applications of Hydroformed Steel Components
Hydroformed steel components are used in a wide range of industries, particularly in applications that require lightweight, high-strength parts.
6.1 Automotive Industry
The automotive industry is one of the largest users of hydroformed steel components. Applications include:
- Chassis frames: Lightweight steel frames and subframes offer better crash performance and fuel efficiency.
- Exhaust systems: Hydroformed steel exhaust components improve airflow and reduce emissions.
- Crash structures: Advanced high-strength steel parts like side-impact beams and bumper reinforcements improve vehicle safety.
6.2 Aerospace Industry
In aerospace, hydroformed steel components are used for:
- Fuselage panels: Lightweight yet strong steel panels ensure structural integrity while minimizing aircraft weight.
- Engine components: Hydroformed parts are used in aircraft engines and exhaust systems due to their strength and ability to withstand extreme temperatures.
6.3 Construction and Energy
In the construction and energy sectors, hydroformed steel parts are used for:
- Structural components: Hydroformed steel beams and supports provide strength and durability for buildings and infrastructure.
- Heat exchangers: Hydroformed steel tubes are used in heat exchangers for power plants, ensuring efficient heat transfer and reduced risk of leaks.
Conclusion
The hydroforming process for steel is a versatile and efficient method for producing high-strength, lightweight components with complex geometries. Whether shaping flat steel sheets or hollow steel tubes, hydroforming offers significant advantages over traditional forming methods, including improved structural integrity, material efficiency, and design flexibility. The process is widely used in industries such as automotive, aerospace, construction, and energy, where the need for lightweight, durable components is critical.
By leveraging the benefits of hydroforming, manufacturers can create innovative, high-performance steel parts that meet the growing demands of modern engineering and industrial applications. As technology continues to advance, hydroforming will remain a key process for producing the next generation of steel components.
Tube Steel Hydroforming: A Comprehensive Guide
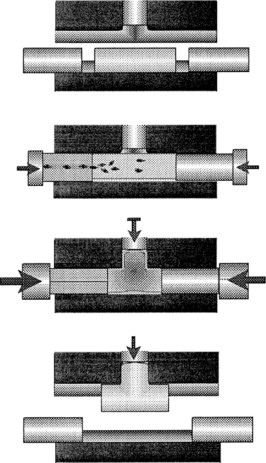
Tube steel hydroforming is a highly advanced and efficient metal forming process that uses internal hydraulic pressure to shape hollow steel tubes into complex, high-precision components. This process is widely employed across industries such as automotive, aerospace, construction, appliances, and energy due to its ability to produce lightweight, high-strength parts with seamless construction and superior mechanical properties.
Tube hydroforming offers several advantages over traditional manufacturing techniques, including the ability to form complex geometries, improved material distribution, and lower production costs. The process enables manufacturers to produce parts with precise tolerances, variable wall thickness, and integrated features such as ribs, flanges, and brackets—all in a single operation.
This article provides an in-depth overview of tube steel hydroforming, discussing the principles, processes, advantages, and applications of this technology. It also covers the types of steel used, the equipment involved, and the challenges faced in tube hydroforming.
1. Overview of Tube Steel Hydroforming
Tube hydroforming is a cold forming process in which a hollow steel tube is placed inside a die and subjected to internal hydraulic pressure. The pressure causes the tube to expand and conform to the shape of the die, creating complex, lightweight, and durable components. The process is ideal for parts that require high strength, low weight, and intricate geometries, making it a popular choice for automotive chassis parts, aerospace structures, and structural components in the construction industry.
Hydroforming provides several benefits over traditional metal forming processes like stamping, bending, or welding:
- Seamless construction: Hydroforming eliminates the need for welded seams, resulting in stronger and more durable parts.
- Complex geometries: The process can create parts with complex 3D shapes, including variable cross-sections, sharp angles, and smooth curves.
- Material efficiency: By precisely controlling material flow, hydroforming reduces waste and minimizes the need for secondary operations like trimming or welding.
2. The Tube Hydroforming Process
The tube hydroforming process involves several steps, from preparing the tube to applying hydraulic pressure and forming the final part. There are two main types of tube hydroforming: high-pressure and low-pressure hydroforming, each suited to different types of parts and applications.
2.1 High-Pressure Tube Hydroforming
High-pressure tube hydroforming is the most common form of the process, used to create parts with complex shapes and deep draws. In this method, the internal hydraulic pressure can reach up to 10,000–20,000 psi or more, depending on the material and part requirements.
Key steps in high-pressure tube hydroforming include:
- Tube Placement: A hollow steel tube is cut to length and placed inside the die. The ends of the tube are sealed, and it is clamped securely to prevent movement during the process.
- Pressurization: Hydraulic fluid is pumped into the tube, gradually increasing the internal pressure. This causes the tube to expand outward, conforming to the shape of the die.
- Axial Feeding: To prevent thinning of the tube walls, axial feeding (applying compressive force to the ends of the tube) may be used. This helps the tube material flow into the die more evenly, ensuring uniform wall thickness.
- Forming: As the hydraulic pressure continues to build, the tube fully conforms to the die, forming the final shape.
- Pressure Holding and Release: The pressure is held for a short period to allow the material to stabilize, then gradually released.
- Part Removal: The formed part is removed from the die, and any excess material at the ends is trimmed if necessary.
2.2 Low-Pressure Tube Hydroforming
In low-pressure tube hydroforming, lower hydraulic pressures (typically below 5,000 psi) are used to shape the tube. This process is often used for shallower draws and parts that require less material deformation. Low-pressure hydroforming is often combined with mechanical forming to assist in shaping the tube.
Key steps in low-pressure tube hydroforming include:
- Tube Placement: A steel tube is placed inside a die, and the ends are sealed.
- Gradual Pressurization: Hydraulic fluid is introduced at a lower pressure, gently expanding the tube to conform to the die.
- Mechanical Assistance: In some cases, a mechanical punch or press is used to help guide the tube into the die.
- Forming and Part Removal: The tube is shaped into the desired form, and the part is removed after the pressure is released.
3. Types of Steel Used in Tube Hydroforming
The choice of steel grade is critical in tube hydroforming, as it directly affects the formability, strength, and durability of the final part. Different types of steel are used depending on the application’s performance requirements and environmental conditions.
3.1 Low-Carbon Steel (Mild Steel)
Low-carbon steel, also known as mild steel, is highly formable and widely used in hydroforming. It offers good ductility, making it ideal for automotive body and chassis components. Mild steel is used in applications where strength is important, but extreme environmental conditions or corrosion resistance are not major concerns.
- Applications: Automotive frames, exhaust systems, and suspension components.
3.2 High-Strength Low-Alloy (HSLA) Steel
HSLA steel is a type of alloy steel that offers higher strength than mild steel while maintaining good formability. HSLA steel is commonly used in structural applications where weight reduction and high performance are key factors.
- Applications: Automotive structural components (e.g., crash structures, frame rails), aerospace parts, and construction beams.
3.3 Stainless Steel
Stainless steel is valued for its corrosion resistance, strength, and durability, making it ideal for applications where environmental exposure is a concern. Stainless steel tubes are commonly hydroformed into parts that require both high strength and protection against rust or corrosion.
- Applications: Automotive exhaust systems, kitchen appliances, and outdoor structural components.
3.4 Advanced High-Strength Steel (AHSS)
Advanced high-strength steel (AHSS) is used in safety-critical applications that require a high strength-to-weight ratio. AHSS combines the benefits of strength and lightweighting, making it a popular choice for automotive crash structures and other parts designed to absorb impact forces.
- Applications: Side-impact beams, bumper reinforcements, and structural reinforcements in the automotive and aerospace industries.
4. Advantages of Tube Hydroforming
Tube hydroforming offers several significant advantages over traditional metal forming methods, particularly for manufacturing hollow components that require complex shapes and high strength.
4.1 Complex Geometries and Design Flexibility
Tube hydroforming allows for the creation of intricate geometries, including curves, sharp angles, and variable cross-sections that are difficult or impossible to achieve using other forming methods. This design flexibility enables manufacturers to create components with integrated features, such as brackets, ribs, and flanges, in a single forming operation.
- Variable wall thickness: Hydroforming can produce components with variable wall thicknesses, optimizing the distribution of material to areas that require extra strength or stiffness.
4.2 Weight Reduction and Material Efficiency
By allowing for precise control over material flow, tube hydroforming enables the production of lightweight components with minimal material waste. This is particularly valuable in industries such as automotive and aerospace, where reducing weight without sacrificing strength is critical for improving fuel efficiency and performance.
- Seamless construction: The process eliminates the need for welding or joining multiple parts, which reduces weight and enhances the structural integrity of the final component.
4.3 Improved Strength and Durability
Hydroformed steel components typically have uniform wall thickness and seamless construction, resulting in parts with superior strength and durability. The absence of weld seams reduces weak points in the part, making it more resistant to fatigue, cracking, and failure.
- Crash safety: In the automotive industry, tube hydroforming is used to create high-strength components that improve vehicle safety by absorbing impact forces in the event of a crash.
4.4 Reduced Tooling and Production Costs
Compared to traditional stamping or deep drawing, tube hydroforming requires simpler tooling and fewer production steps. Because the process combines forming and shaping in a single operation, manufacturers can reduce tooling costs, eliminate secondary operations, and shorten production times.
- Fewer assembly operations: By forming complex parts in one step, hydroforming reduces the need for welding, fastening, or joining, which improves overall manufacturing efficiency.
5. Applications of Tube Hydroforming
Tube hydroforming is used across a wide range of industries, particularly where lightweight, high-strength steel components are required. Below are some of the key applications of hydroformed steel tubes.
5.1 Automotive Industry
The automotive industry is one of the largest users of tube hydroforming, as it allows for the production of lightweight, crash-resistant components that improve vehicle safety, performance, and fuel efficiency.
- Chassis and frames: Hydroformed steel tubes are used to create chassis components, such as frame rails, subframes, and engine cradles, that provide strength while reducing vehicle weight.
- Exhaust systems: Automotive exhaust manifolds and pipes are hydroformed to optimize airflow, reduce backpressure, and improve engine performance.
- Crash structures: Side-impact beams and bumper reinforcements are hydroformed from AHSS to absorb impact forces and enhance passenger safety.
5.2 Aerospace Industry
In the aerospace industry, tube hydroforming is used to create lightweight, high-strength components that can withstand the extreme loads and conditions experienced during flight.
- Fuselage and wing components: Hydroformed steel tubes are used in aircraft fuselages and wings to reduce weight while maintaining structural integrity.
- Engine components: Hydroformed steel parts are used in aircraft engines and exhaust systems, where high-temperature performance and strength are essential.
5.3 Construction and Architecture
In the construction industry, tube hydroforming is used to produce structural supports, columns, and beams for buildings, bridges, and infrastructure projects. Hydroformed steel tubes provide strength, durability, and aesthetic appeal, making them ideal for both structural and decorative applications.
- Structural supports: Hydroformed steel tubes are used as load-bearing supports in buildings and bridges, where they provide strength and stability.
- Architectural elements: Tube hydroforming allows for the creation of custom architectural features, such as curved or tapered steel columns, that enhance both the structural performance and visual appeal of buildings.
5.4 Energy and Power Generation
The energy sector uses hydroformed steel tubes in the production of pipelines, heat exchangers, and pressure vessels. Hydroforming enables the production of seamless, high-strength components that can withstand high pressures and temperatures, making it ideal for use in power plants, oil and gas facilities, and renewable energy systems.
- Heat exchangers: Hydroformed steel tubes are used in heat exchangers for efficient heat transfer in power generation systems, including nuclear, gas, and coal-fired plants.
- Pipelines: The seamless construction of hydroformed steel tubes makes them ideal for oil, gas, and water pipelines that must withstand high internal pressures.
6. Challenges in Tube Hydroforming
While tube hydroforming offers numerous advantages, there are several challenges associated with the process, particularly when working with steel.
6.1 Material Limitations
Not all steel grades are suitable for hydroforming. Materials with low ductility or poor formability, such as high-carbon steels, may crack or fail during the process, limiting the types of steel that can be hydroformed.
- Formability: To prevent failure, it is critical to select steel grades with good formability, such as mild steel, HSLA steel, or AHSS.
6.2 Equipment Costs
The initial investment in hydroforming equipment can be high, particularly for high-pressure hydroforming systems that require powerful hydraulic presses and specialized tooling. While the process is cost-effective for large-scale production, the upfront costs may be prohibitive for small-scale manufacturers.
6.3 Long Cycle Times
Compared to traditional metal forming methods like stamping, tube hydroforming can have longer cycle times, particularly for parts with complex geometries. The process may also require multiple pressure cycles to achieve the desired final shape, which can increase production time.
Conclusion
Tube steel hydroforming is a versatile and efficient manufacturing process that allows for the production of lightweight, high-strength components with complex geometries and seamless construction. By using hydraulic pressure to shape hollow steel tubes, hydroforming enables manufacturers to create parts with uniform wall thickness, superior strength, and precise tolerances—all in a single operation.
From automotive chassis frames and aerospace components to construction supports and energy infrastructure, hydroformed steel tubes are used in a wide range of industries where performance, durability, and cost-efficiency are critical. Despite some challenges, such as equipment costs and material limitations, the advantages of tube hydroforming, including design flexibility, material efficiency, and improved part quality, make it an indispensable technology for modern manufacturing.
As industries continue to seek out lightweight, high-performance components, tube steel hydroforming will remain a key process in shaping the future of automotive, aerospace, construction, and energy systems.
Advantages of Hydroforming Steel
Hydroforming is a versatile metal forming process that uses high-pressure hydraulic fluid to shape steel into complex, high-strength, and lightweight components. This process has gained widespread adoption across industries such as automotive, aerospace, construction, appliances, and energy due to its ability to form parts with superior mechanical properties, seamless construction, and precision. Hydroforming offers several key advantages over traditional metal forming methods, including improved material efficiency, structural integrity, weight reduction, and cost-effectiveness.
In steel hydroforming, both sheet hydroforming and tube hydroforming processes are used to create intricate components that require high precision and durability. Hydroforming allows manufacturers to create parts with variable wall thickness, complex geometries, and sharp angles, which are difficult to achieve using conventional stamping, pressing, or welding techniques. Moreover, the process reduces the need for secondary operations and results in a smoother surface finish, further enhancing the quality of the final product.
This article provides a comprehensive overview of the advantages of hydroforming steel, examining the benefits it offers over traditional manufacturing techniques. It also explores the applications of hydroformed steel components in various industries and the role this process plays in enhancing product performance, sustainability, and cost savings.
1. Weight Reduction and Material Efficiency
One of the primary advantages of hydroforming steel is its ability to produce lightweight components with minimal material waste. The process allows manufacturers to create parts that are stronger, lighter, and more efficient in terms of material usage, which is critical in industries such as automotive and aerospace, where reducing weight without compromising strength is a key priority.
1.1 Lightweight Components
Hydroforming steel enables the creation of lightweight parts with thin walls and complex shapes, making it an ideal solution for industries seeking to improve fuel efficiency, performance, and sustainability. By carefully controlling the material flow during the forming process, hydroforming allows for the production of parts that are both strong and lightweight, without the need for excessive material usage.
- Automotive Industry: Reducing vehicle weight is critical for improving fuel efficiency and reducing emissions. Hydroformed steel components, such as chassis frames, crash structures, and exhaust systems, offer superior strength-to-weight ratios, helping automakers meet regulatory standards for fuel economy and emissions reduction.
- Aerospace Industry: In aerospace, weight reduction is equally important for improving fuel efficiency and payload capacity. Hydroforming steel allows for the creation of lightweight fuselage panels, aircraft engine components, and structural parts, contributing to the overall performance of aircraft.
1.2 Material Efficiency and Waste Reduction
Hydroforming is known for its material efficiency, as it minimizes the need for excess trimming, cutting, or welding. Traditional metal forming processes like stamping or deep drawing often require multiple forming stages and result in significant material waste. In contrast, hydroforming shapes steel directly into the final part, reducing the need for secondary operations and minimizing material loss.
- Less Scrap: Hydroforming produces less scrap compared to traditional methods, which often require the removal of excess material to achieve the desired shape. This not only reduces material costs but also contributes to a more sustainable manufacturing process.
- Optimized Material Usage: The process allows manufacturers to optimize material usage by precisely controlling the thickness of the steel throughout the part, ensuring that material is distributed where it is needed most for strength and stiffness.
2. Complex Geometries and Design Flexibility
One of the most significant advantages of hydroforming steel is its ability to create complex geometries and intricate shapes that would be difficult or impossible to achieve using traditional metal forming methods. This design flexibility is particularly valuable in industries such as automotive and aerospace, where components often require sharp angles, smooth curves, and variable wall thicknesses.
2.1 Intricate Shapes and Features
Hydroforming allows manufacturers to produce parts with 3D geometries, including sharp radii, flanged edges, ribs, and recesses—all in a single forming operation. This capability enables the creation of parts that combine aesthetic appeal with structural integrity.
- Automotive Components: Hydroforming steel is commonly used to produce automotive body panels, chassis components, and crash structures with complex designs that offer both strength and lightweighting advantages. For example, side-impact beams and bumper reinforcements are hydroformed to provide optimal energy absorption during a crash.
- Aerospace Applications: In aerospace, hydroforming is used to create fuselage panels, wing skins, and engine components that require precise shapes and tight tolerances. The process allows for the integration of multiple features, such as flanges and brackets, into a single part, reducing the need for assembly and welding.
2.2 Variable Wall Thickness
Hydroforming allows for the creation of parts with variable wall thickness, which can be adjusted to meet specific design requirements. This capability enables manufacturers to add material where it is needed for strength and stiffness, while reducing thickness in areas where it is not required, resulting in lighter, stronger parts.
- Structural Components: In automotive and aerospace applications, parts like frame rails and crossmembers benefit from variable wall thicknesses, which help distribute loads more evenly and improve crash performance.
- Tubes with Complex Cross-Sections: Tube hydroforming, in particular, allows for the creation of tubes with variable cross-sections, such as oval or rectangular shapes, which are commonly used in exhaust systems and bicycle frames.
3. Improved Structural Integrity and Strength
Hydroformed steel components are known for their superior structural integrity and mechanical strength, thanks to the uniform material distribution and seamless construction achieved during the process. This makes hydroforming an ideal solution for parts that must withstand high loads, impact forces, and extreme conditions.
3.1 Uniform Wall Thickness and Material Distribution
Hydroforming applies uniform hydraulic pressure to the steel, ensuring even material flow and consistent wall thickness throughout the part. This eliminates the localized thinning or tearing that can occur with traditional forming methods, resulting in parts that are stronger and more durable.
- Crash-Resistant Components: In the automotive industry, components such as crash beams, roof rails, and side-impact structures benefit from the uniform wall thickness and enhanced strength provided by hydroforming. These parts are designed to absorb and dissipate energy during a collision, improving vehicle safety.
- Pressure Vessels: In industries such as oil and gas and energy, hydroformed steel is used to create pressure vessels and storage tanks that require high structural integrity to withstand high internal pressures and harsh environmental conditions.
3.2 Seamless Construction
One of the major advantages of hydroforming is the ability to produce seamless components that eliminate the need for welding or joining multiple parts. The absence of weld seams reduces the risk of weak points and improves the overall strength and durability of the component.
- Exhaust Systems: In automotive exhaust systems, hydroformed steel tubes offer superior strength and durability compared to welded tubes, which are more prone to corrosion and fatigue.
- Aerospace and Defense Applications: In the aerospace and defense sectors, hydroformed components are used in critical structural applications, such as aircraft fuselages and military vehicle armor, where seamless construction is essential for withstanding high loads and impact forces.
4. Cost Efficiency and Reduced Tooling
Hydroforming offers significant cost advantages over traditional metal forming methods, particularly when it comes to tooling and production efficiency. The process allows for the creation of complex parts using simpler, less expensive tooling, reducing overall manufacturing costs.
4.1 Lower Tooling Costs
Unlike traditional stamping or deep drawing, which requires matched dies (both upper and lower dies), hydroforming typically uses only a single-sided die, as hydraulic pressure from a flexible diaphragm or internal fluid replaces the need for an upper die. This reduces the complexity and cost of tooling, particularly for parts with intricate shapes.
- Simplified Tooling: Hydroforming eliminates the need for complex tooling systems, allowing manufacturers to produce high-precision parts with lower upfront investment in tooling.
- Flexibility: The same hydroforming press can be used to produce a variety of different parts by simply changing the die, offering greater flexibility and cost-effectiveness in high-mix, low-volume production.
4.2 Fewer Secondary Operations
Because hydroforming produces parts with precise geometries, smooth surfaces, and integrated features, the need for secondary operations such as trimming, welding, or machining is greatly reduced. This results in shorter production times, lower labor costs, and improved efficiency.
- One-Step Forming: Hydroforming combines multiple operations, such
Applications of Hydroformed Steel
Hydroformed steel components are widely used across various industries due to their lightweight, high-strength characteristics, and ability to form complex geometries. Hydroforming processes allow manufacturers to create seamless, structurally strong parts that are critical for sectors like automotive, aerospace, construction, appliances, and energy.
1. Automotive Industry
The automotive industry is one of the largest beneficiaries of hydroformed steel technology. Components produced via hydroforming, such as chassis frames, engine cradles, and exhaust systems, have become integral in car manufacturing. Hydroforming enables the production of lightweight but rigid structures, reducing vehicle weight, which directly contributes to improved fuel efficiency and reduced emissions.
- Chassis and Frame Rails: Hydroforming produces automotive frame rails and other load-bearing structures, offering increased rigidity and crash performance. The uniform material distribution ensures that high-impact areas are reinforced, enhancing safety during collisions.
- Exhaust Systems: The process is used to create seamless exhaust manifolds and pipes, reducing the need for welding. This improves durability, performance, and corrosion resistance, leading to better exhaust flow and lower emissions
- Crash Components: Hydroforming enables the creation of crash-resistant parts like side-impact beams and bumper reinforcements, which are crucial for absorbing energy in the event of an accident
2. Aerospace Industry
In the aerospace sector, the lightweight and strong characteristics of hydroformed steel make it ideal for use in aircraft manufacturing. This industry relies on components that can withstand extreme forces and temperatures while remaining as light as possible to improve fuel efficiency and reduce costs.
- Aircraft Fuselage Components: Hydroformed steel is used to manufacture parts like wing skins, ribs, and bulkheads. These components provide structural integrity while keeping the aircraft lightweight.
- Engine Components: Aerospace applications often require complex geometries, such as those found in jet engine housings and exhaust systems, where hydroforming is used to shape high-strength steel without compromising material properties.
- Landing Gear: Components like struts and landing gear supports are hydroformed to meet high safety standards, ensuring they can handle the stress of repeated takeoffs and landings
3. Energy and Power Generation
In the energy sector, hydroformed steel is widely used to create pressure vessels, pipelines, and heat exchangers. These components are critical in industries like oil and gas, nuclear, and renewable energy, where they must withstand high pressures and harsh environments.
- Heat Exchangers: Hydroformed steel tubes are ideal for heat exchangers used in power plants and industrial systems. Their seamless construction ensures efficient heat transfer and reduces the risk of leaks.
- Pipelines: The use of hydroformed steel in oil and gas pipelines allows for the creation of long, seamless sections that can handle high-pressure fluid transport, improving reliability and reducing maintenance costs
4. Construction and Architecture
Hydroformed steel is also used in construction and architectural applications, where strength, durability, and aesthetic appeal are important. Steel components formed through hydroforming offer increased structural stability, making them suitable for support beams, columns, and decorative architectural elements.
- Structural Beams: Hydroformed steel beams provide enhanced load-bearing capabilities, allowing for more efficient construction of high-rise buildings, bridges, and infrastructure projects.
- Architectural Designs: The ability to form complex shapes with hydroformed steel makes it ideal for custom architectural features, such as curved or tapered columns, which are commonly used in modern building designs
5. Medical and Healthcare
In the medical field, hydroformed steel components are used in the manufacture of surgical instruments, medical devices, and equipment housings. The process allows for the creation of precise, seamless parts that meet strict sanitary standards, ensuring the safety and reliability of medical products.
- Surgical Trays and Instruments: Hydroforming is used to produce lightweight, durable surgical trays, as well as covers for operating room lights and other medical devices that require smooth, clean surfaces.
- Equipment Housings: Medical equipment, including diagnostic machines, benefit from the precision and durability of hydroformed steel, ensuring that the devices remain reliable and easy to sterilize
6. Consumer Products
Hydroforming is also applied in the production of consumer products, particularly in industries that require high-quality surface finishes and lightweight, durable components.
- Appliances: Hydroformed steel is used to produce parts like refrigerator liners, oven panels, and washing machine drums. These components benefit from the high precision and smooth surface finishes achieved through hydroforming, reducing the need for additional finishing.
- Bicycles and Sports Equipment: Hydroformed steel tubes are used in bicycle frames and outdoor sports equipment due to their strength, lightweight properties, and ability to withstand high-impact forces
Limitations of Hydroforming Steel
Despite its many advantages, hydroforming steel also comes with certain limitations that can restrict its use in some applications. The challenges associated with hydroforming primarily relate to process costs, equipment complexity, material limitations, and cycle times.
1. High Initial Investment and Equipment Costs
One of the major limitations of hydroforming steel is the high initial investment required for hydroforming presses and specialized equipment. The hydraulic systems used in hydroforming need to generate extremely high pressures to form steel, which requires heavy-duty presses and expensive dies.
- Equipment Costs: The cost of hydroforming machines and tooling can be prohibitive, particularly for small-scale manufacturers or companies that produce low-volume parts. This limits the feasibility of hydroforming for smaller production runs or businesses with limited capital.
- Tooling: While hydroforming requires less tooling than traditional stamping or pressing, the specialized dies used in the process still represent a significant upfront cost
2. Long Cycle Times
Compared to traditional metal forming processes, hydroforming can have longer cycle times, especially for complex or large parts. The need to gradually apply hydraulic pressure and allow the steel to conform to the die means that each part may take longer to form.
- Slow Production Speeds: Hydroforming is not as fast as processes like stamping, making it less suitable for applications that require high-volume production. This can lead to higher labor costs and lower throughput, especially in industries where time-to-market is critical
3. Material Limitations
Not all steel grades are suitable for hydroforming. Materials with low ductility or poor formability may crack, wrinkle, or fail during the hydroforming process, limiting the types of steel that can be used effectively.
- Formability Issues: High-carbon steels, for example, may not perform well under the stresses of hydroforming, leading to cracking or material thinning in areas of high strain. Manufacturers must carefully select steel grades that offer sufficient ductility and formability to ensure the success of the hydroforming process
4. Thickness Limitations
While hydroforming excels at producing thin-walled components, it is less effective for forming parts with thicker walls. The process is typically used for steel parts with wall thicknesses of up to a certain limit, beyond which it becomes difficult to apply sufficient hydraulic pressure without causing material failure.
- Thick-Walled Parts: For parts that require thicker walls to meet strength or durability requirements, hydroforming may not be the most suitable process. In such cases, other metal forming techniques, such as forging or casting, may be preferred
5. Complexity of Process Control
Hydroforming requires precise control over pressure application, material flow, and axial force to achieve the desired part geometry without defects. This complexity increases with the size and intricacy of the part being formed, making the process more challenging for manufacturers to manage.
- Pressure Control: Insufficient pressure during the process can lead to wrinkling or incomplete part formation, while excessive pressure can cause thinning or fracturing of the material. This requires advanced monitoring and control systems to ensure optimal performance
Tubular Hydroforming is a specialized metal forming process that uses hydraulic pressure to shape metal tubes into complex, high-strength components. This technique, a subset of hydroforming, is particularly effective for manufacturing hollow, seamless parts that require intricate shapes or consistent wall thicknesses. Tubular hydroforming has applications across several industries, especially automotive, aerospace, bicycles, and furniture, where lightweight and structurally sound components are essential.
Key Aspects of Tubular Hydroforming
- Process Overview: Tubular hydroforming involves placing a pre-cut, hollow metal tube (commonly steel, aluminum, or stainless steel) into a specially designed die. Hydraulic fluid is then pumped into the tube at high pressures, forcing it to expand and take on the shape of the die. In some cases, axial compressive forces are applied from the tube’s ends to control material flow, which helps maintain consistent wall thickness throughout the component.
- Types of Tubular Hydroforming:
- High-Pressure Hydroforming: Uses high internal pressure to form complex shapes, such as automotive structural components like subframes and engine cradles.
- Low-Pressure Hydroforming: Utilizes lower hydraulic pressures combined with axial forces to shape the tube gently, often for parts that do not require deep draws or complex designs.
- Applications:
- Automotive Industry: Tubular hydroforming is heavily used for chassis components, engine cradles, frame rails, exhaust systems, and suspension parts. This process enables manufacturers to produce lightweight, strong, and safety-compliant components.
- Aerospace and Bicycle Manufacturing: In aerospace, hydroformed tubular components help reduce aircraft weight while ensuring high strength. For bicycles, hydroforming allows the creation of robust frames that can withstand impact while remaining lightweight.
- Construction and Furniture: Architectural and furniture applications include hydroformed tubes for durable structural elements and aesthetically appealing designs that also provide strength and support.
- Advantages:
- Enhanced Strength and Lightweighting: Tubular hydroforming creates high-strength parts with minimal weight, reducing fuel consumption in vehicles and contributing to energy efficiency.
- Design Flexibility: The process allows manufacturers to create complex, multi-dimensional shapes with integrated features like brackets and flanges.
- Cost Efficiency: By eliminating the need for welding and reducing waste, tubular hydroforming reduces production costs, especially in high-volume manufacturing.
- Challenges:
- Equipment and Tooling Costs: The process requires high-capacity hydraulic presses and durable dies, making the initial setup costly.
- Material Limitations: Not all materials are suitable; ductility is crucial, as brittle materials can crack under high hydraulic pressure.
- Cycle Time: Tubular hydroforming can take longer than stamping, which may be a limitation in high-speed production settings.
Summary
Tubular hydroforming is highly beneficial for producing complex, lightweight components with seamless construction and precise dimensional control. Its applications across multiple sectors underscore its versatility and importance in modern manufacturing. However, manufacturers must balance the benefits with the initial cost and material limitations to determine whether this process best meets their production needs.
Key Aspects of Tubular Hydroforming
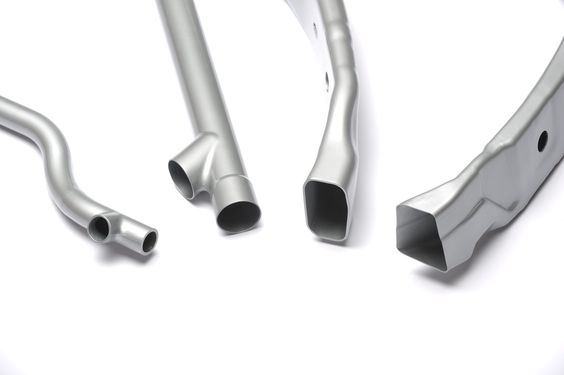
Tubular hydroforming is an advanced metal-forming process used to shape metal tubes into complex geometries by applying high-pressure hydraulic fluid. This technology is pivotal in industries where strength, durability, and weight reduction are critical, such as automotive, aerospace, and construction. By leveraging hydraulic pressure, tubular hydroforming creates components with uniform thickness, precise dimensions, and seamless construction, which are difficult to achieve using traditional methods like stamping or welding.
This article delves into the key aspects of tubular hydroforming, exploring the technology’s fundamentals, types of processes, benefits, applications, challenges, and the materials best suited for this innovative approach.
1. The Fundamentals of Tubular Hydroforming
Tubular hydroforming is based on applying hydraulic pressure to a metal tube, forcing it to expand and conform to the shape of a surrounding die. This process allows manufacturers to create complex, high-strength components with minimum material waste and seamless construction. The process requires careful control of pressure and material flow to achieve the desired shape without defects like wrinkling or tearing.
Process Overview
- Tube Preparation: A hollow metal tube (often steel, aluminum, or stainless steel) is cut to the required length and placed within the die.
- Clamping and Sealing: The tube’s ends are clamped and sealed, ensuring that hydraulic fluid can pressurize the tube without leaks.
- Hydraulic Pressurization: Hydraulic fluid is pumped into the tube, and pressure builds internally. This pressure, combined with axial forces applied at the ends, forces the tube to expand and take on the die’s shape.
- Shaping and Stabilization: The hydraulic pressure is maintained briefly to stabilize the material and ensure uniform wall thickness.
- Pressure Release and Part Removal: Once the desired shape is achieved, the hydraulic pressure is released, and the component is removed from the die.
2. Types of Tubular Hydroforming Processes
The choice of tubular hydroforming process depends on the component’s complexity, material type, and application requirements. High-pressure and low-pressure hydroforming are the two primary types, each suited for different manufacturing goals.
2.1 High-Pressure Hydroforming
High-pressure hydroforming is used to create parts with complex, tight-radius shapes that require significant material deformation. This method involves applying high hydraulic pressure—often exceeding 10,000 psi—inside the tube, forcing it to expand and conform closely to the die. The high-pressure approach allows for the creation of intricate, 3D shapes that require precision, such as automotive chassis parts and aerospace structural components.
- Applications: Automotive frame rails, crash-resistant components, structural aerospace parts.
- Advantages: Creates intricate shapes with high strength, uniform thickness, and minimal defects.
2.2 Low-Pressure Hydroforming
Low-pressure hydroforming uses significantly lower hydraulic pressure, combined with axial force, to shape the tube gently. This method is often used for shallower shapes and components that do not require deep draws. It is ideal for applications where precise control over wall thickness and material distribution is critical.
- Applications: Automotive exhaust systems, bicycle frames, and tubes with complex cross-sections.
- Advantages: Lower equipment cost, reduced material strain, ideal for parts requiring minimal deformation.
3. Benefits of Tubular Hydroforming
The advantages of tubular hydroforming make it an appealing choice for manufacturers aiming to produce lightweight, durable, and cost-effective components.
3.1 Lightweighting and Structural Efficiency
Hydroformed tubes are lightweight yet structurally sound, making them ideal for industries focused on weight reduction and fuel efficiency, such as automotive and aerospace. By optimizing wall thickness and using a seamless design, tubular hydroforming minimizes weight while maximizing strength.
- Automotive Sector: Lightweight hydroformed steel reduces vehicle weight, improving fuel efficiency and reducing emissions.
- Aerospace Sector: Hydroformed components help decrease aircraft weight, which enhances payload capacity and fuel economy.
3.2 Material and Cost Efficiency
Hydroforming allows manufacturers to use material more efficiently, as the process reduces waste by shaping metal tubes directly to the desired form. Additionally, the process typically requires fewer secondary operations like welding or trimming, which reduces production costs and speeds up the manufacturing process.
- Single-Part Formation: Complex shapes can be formed in one step, eliminating the need for multiple assembly stages.
- Reduced Scrap: Less material is wasted, contributing to cost savings and sustainability.
3.3 Complex Geometries and Seamless Construction
Hydroforming is highly effective at producing complex shapes with multi-dimensional geometries. Tubular components with integrated features—such as flanges, ribs, and brackets—can be created in a single forming operation, increasing design flexibility while reducing the need for additional manufacturing steps.
- Automotive Frames and Chassis: Hydroforming enables the creation of complex, load-bearing frames that offer safety and stability.
- Architectural Structures: The ability to form tubes with aesthetic curves and custom cross-sections makes hydroformed tubes appealing for architectural applications.
4. Applications of Tubular Hydroforming
Tubular hydroforming’s unique properties make it applicable across several industries. Its combination of strength, lightweighting, and design flexibility has made it an indispensable manufacturing technique for many sectors.
4.1 Automotive Industry
The automotive industry uses tubular hydroforming extensively to produce structural components that enhance vehicle safety, performance, and fuel efficiency. Hydroformed parts reduce vehicle weight while maintaining high strength, making them ideal for components like frame rails, engine cradles, and side-impact beams.
- Chassis Components: Hydroformed steel chassis frames and cross-members offer improved rigidity and crash performance.
- Exhaust Systems: Hydroformed tubes in exhaust manifolds improve airflow, reduce backpressure, and minimize emissions, contributing to cleaner exhaust systems.
4.2 Aerospace Industry
In aerospace, hydroforming is used to create lightweight, high-strength parts that withstand the high stresses of flight. Tubular hydroforming allows for the production of components with consistent wall thickness and smooth surfaces, ideal for applications that demand structural integrity and weight reduction.
- Fuselage and Wing Components: Hydroformed steel tubes serve as lightweight reinforcements in aircraft fuselages and wings.
- Engine Components: Complex engine parts, including turbine housings and exhaust ducts, benefit from hydroforming’s ability to create seamless structures.
4.3 Construction and Architecture
Hydroforming allows architects and builders to create structurally robust and visually appealing designs. Tubular hydroformed steel components are used in support columns, beams, and decorative structures, where strength, durability, and flexibility are paramount.
- Structural Beams: Hydroformed steel beams and supports offer increased load-bearing capacity.
- Architectural Features: The smooth curves and customized shapes possible with hydroforming are often employed in decorative architecture.
4.4 Bicycles and Sports Equipment
Bicycle manufacturers leverage hydroforming to create lightweight yet durable frames. By shaping the metal into streamlined forms with variable wall thickness, hydroformed bicycle frames withstand stress and impacts effectively, providing strength without added weight.
- Bicycle Frames: Hydroformed aluminum and steel frames offer cyclists greater durability, efficiency, and aesthetics.
- Outdoor Sports Equipment: Hydroformed tubular components are used in various sports gear, including structural supports in high-stress equipment.
5. Challenges of Tubular Hydroforming
Despite its advantages, tubular hydroforming presents several challenges that manufacturers must consider, especially when implementing the process on a large scale.
5.1 High Initial Equipment Costs
The initial setup for tubular hydroforming requires expensive hydraulic presses and specialized dies. The cost of this equipment can be prohibitive, especially for smaller manufacturers or low-volume production runs. The tooling costs for dies, particularly in high-pressure hydroforming, add further expense to the initial setup.
- Capital Investment: High-capacity hydraulic systems and tooling costs can limit hydroforming’s feasibility for small-scale operations.
- Maintenance Costs: The equipment used in hydroforming requires regular maintenance to ensure consistent performance, adding to ongoing operational costs.
5.2 Material Limitations
Not all metals are compatible with hydroforming. While the process is highly effective for materials with high ductility—such as aluminum, mild steel, and stainless steel—brittle metals or materials with low malleability can fracture or fail under high-pressure conditions.
- Material Constraints: High-carbon steels or materials with low stretchability may experience cracking during the hydroforming process, limiting the materials suitable for certain applications.
- Specialty Alloys: Using specialty alloys may help overcome some material limitations but can increase costs significantly.
5.3 Cycle Time and Production Speed
Compared to other manufacturing processes, hydroforming can have longer cycle times, particularly for complex parts. The need for precise pressure control and gradual shaping can slow down production, making it less suitable for applications that require high production speeds.
- Longer Forming Times: The hydraulic process takes longer than stamping or deep drawing, making it challenging for high-volume production.
- Automation Needs: While automated hydroforming equipment can help increase speed, this setup is costly and may not be feasible for smaller manufacturers.
Tubular hydroforming is a highly specialized metal-forming process that utilizes hydraulic pressure to shape metal tubes into complex geometries. This process is used extensively in industries like automotive, aerospace, and furniture manufacturing due to its ability to produce lightweight, high-strength components with seamless construction and consistent wall thickness. Depending on the application requirements, there are various types of tubular hydroforming, each designed to accommodate different material properties, component shapes, and production volumes.
1. High-Pressure Tubular Hydroforming
High-pressure tubular hydroforming is one of the most widely used techniques for shaping metal tubes, especially in applications where complex geometries and tight tolerances are required. This process involves applying hydraulic pressure, often exceeding 10,000 psi, inside the tube, causing it to expand and conform closely to the contours of the surrounding die.
1.1 Process Characteristics
- Hydraulic Pressurization: The metal tube is placed in a die, and hydraulic fluid is pumped into the tube, gradually increasing pressure until the tube fully expands to the shape of the die.
- Axial Feeding: To maintain even wall thickness and avoid thinning in specific areas, additional compressive force (known as axial feeding) may be applied to the tube’s ends. This ensures that the material flows smoothly and uniformly into the die cavity.
- Precision Control: High-pressure hydroforming requires precise control over pressure application and material flow to achieve complex shapes without defects like wrinkling or cracking.
1.2 Applications
High-pressure tubular hydroforming is ideal for creating structurally robust components with complex shapes, such as:
- Automotive chassis components: Frame rails, crash-resistant beams, and subframes, which require high strength and energy-absorbing properties for vehicle safety.
- Aerospace structural components: Tubular parts for aircraft fuselages, wings, and engine mounts that must withstand high stress and maintain low weight.
1.3 Advantages
- Greater Design Flexibility: Allows for intricate shapes with variable cross-sections, integrated flanges, and reinforcement features.
- High Structural Integrity: The process produces seamless, uniform parts with minimal stress points, enhancing durability and resistance to fatigue.
2. Low-Pressure Tubular Hydroforming
Low-pressure tubular hydroforming is a process variant that uses significantly lower hydraulic pressures (typically below 5,000 psi) compared to high-pressure hydroforming. This method is more suitable for less complex shapes and applications where the material does not need to expand as dramatically.
2.1 Process Characteristics
- Gentle Shaping: Low-pressure hydroforming shapes the tube by applying hydraulic pressure and axial compressive force to guide the material into the die without significant expansion.
- Mechanical Assistance: In some cases, mechanical presses or punches are used to help shape the tube in conjunction with hydraulic pressure, further reducing the need for high pressure.
2.2 Applications
Low-pressure tubular hydroforming is often used in applications that prioritize minimal deformation and surface quality over extreme material stretching. Typical applications include:
- Automotive exhaust systems: Where smooth curves and consistent wall thickness are important for airflow and exhaust gas management.
- Bicycle frames: Which require lightweight yet durable tubing without the need for complex shapes.
2.3 Advantages
- Reduced Equipment and Maintenance Costs: Lower pressures result in less wear and tear on equipment and dies, reducing maintenance requirements and prolonging tool life.
- Minimized Material Stress: Ideal for parts where surface finish and material consistency are essential, as low-pressure hydroforming reduces the risk of surface defects and internal stress.
3. Hybrid Hydroforming Processes
Hybrid hydroforming combines elements of both high-pressure and low-pressure techniques, as well as other metal-forming methods like mechanical pressing and stretch forming. This approach enables manufacturers to achieve complex shapes with high precision while maintaining control over material thickness and wall integrity.
3.1 Process Characteristics
- Combined Pressure and Force: Hybrid hydroforming uses a mix of hydraulic pressure and mechanical force to shape the tube, allowing for finer control over material flow.
- Custom Tooling: Hybrid processes may require specialized dies and tools, as they incorporate aspects of both traditional and hydroforming methods to meet specific part requirements.
3.2 Applications
Hybrid hydroforming is often used for components that require extreme precision and variable wall thickness. These applications include:
- High-performance bicycle frames: Where manufacturers can precisely control wall thickness for weight reduction and impact resistance.
- Medical devices and surgical instruments: Where durability and exact geometry are crucial, such as in specialized tubing for catheters or orthopedic supports.
3.3 Advantages
- Enhanced Precision: Hybrid methods provide manufacturers with greater flexibility to achieve precise wall thickness and complex shapes without sacrificing strength.
- Adaptability: The combination of different forming techniques allows for a broader range of part designs, catering to both complex and simpler shapes.
4. Warm Hydroforming
Warm hydroforming involves preheating the metal tube before or during the hydroforming process. The elevated temperature increases the ductility of certain metals, especially those with lower natural malleability, enabling them to be formed more effectively. Warm hydroforming is particularly useful for metals like high-strength steel and titanium.
4.1 Process Characteristics
- Controlled Heating: The tube is heated to a specific temperature to improve its plasticity, making it easier to shape under pressure without cracking.
- Temperature Management: Precise temperature control is essential, as overheating can weaken the material or affect its microstructure, while underheating may not sufficiently improve ductility.
4.2 Applications
Warm hydroforming is commonly used for high-strength materials that require greater flexibility during the forming process. Typical applications include:
- Automotive safety components: Such as high-strength crash beams and structural supports where traditional hydroforming would risk cracking.
- Aerospace and defense parts: For components that need to withstand high stress and impact forces, like engine mounts and structural frames.
4.3 Advantages
- Expanded Material Options: The ability to form high-strength materials like advanced high-strength steel (AHSS) and titanium opens up more applications for hydroforming.
- Increased Ductility: Warm hydroforming allows for more extensive material shaping without compromising component strength.
5. Hydro-Mechanical Forming
Hydro-mechanical forming is a process in which both hydraulic pressure and a mechanical punch are used to shape the tube. This hybrid technique leverages the precision of hydraulic forming and the force of mechanical pressing to achieve deep draws and complex forms.
5.1 Process Characteristics
- Mechanical and Hydraulic Integration: The mechanical punch initiates deformation, followed by hydraulic pressure to complete the shaping, allowing for deep, complex shapes.
- High Forming Pressure: While hydraulic pressure helps shape the material, the mechanical punch enhances material flow and allows for greater wall thickness control.
5.2 Applications
Hydro-mechanical forming is commonly used in automotive and industrial machinery where components require strength and detailed geometry. Applications include:
- Deep-drawn parts: Such as chassis components with deep cross-sections.
- Industrial tooling: Parts that require high rigidity and specific shapes for heavy machinery.
5.3 Advantages
- Better Control of Material Flow: Combining hydraulic pressure with mechanical force allows for greater control over wall thickness, essential for parts with varying cross-sections.
- High-Quality Surface Finishes: The process reduces defects like wrinkling and thinning, creating parts with improved aesthetics and mechanical properties.
6. Explosive Hydroforming
Explosive hydroforming is a highly specialized hydroforming process that uses an explosive charge to generate the necessary hydraulic pressure. While not widely used, this technique is effective for forming very large components or high-strength materials that are difficult to shape using traditional hydroforming methods.
6.1 Process Characteristics
- Explosive Force: A controlled explosion in a water-filled chamber generates a shockwave that forces the tube against the die.
- High-Pressure Output: Explosive hydroforming produces pressures much higher than conventional hydraulic systems, making it suitable for challenging materials or large parts.
6.2 Applications
Explosive hydroforming is used primarily in aerospace, defense, and energy sectors where high-strength, large-scale components are required. Applications include:
- Aircraft components: Large fuselage sections, engine housings, and wing structures.
- Nuclear and energy sectors: Pressure vessels and containment systems designed to withstand extreme forces.
6.3 Advantages
- Unmatched Forming Power: The explosive force allows for the shaping of ultra-high-strength materials and very large parts.
- Suitable for Large Components: Explosive hydroforming is one of the few methods capable of forming very large and thick-walled parts without excessive equipment costs.
7. Applications of Different Types of Tubular Hydroforming
Each type of tubular hydroforming serves distinct needs across multiple industries:
- Automotive: High-pressure and low-pressure hydroforming are used extensively for frame rails, exhaust systems, and crash components, whileTubular hydroforming encompasses a variety of methods that shape metal tubes by applying hydraulic pressure, each method tailored to specific application needs. Here’s a deeper look at each type, showcasing their unique applications, strengths, and suitability for different industries.
1. High-Pressure Hydroforming
High-pressure tubular hydroforming involves intense hydraulic pressures (often above 10,000 psi), ideal for creating complex, load-bearing structures with high precision and consistency in wall thickness.
- Process: Hydraulic pressure inside the tube forces it to expand fully within a surrounding die. Additional axial compression (pushing at the ends) helps the material flow smoothly, maintaining thickness in areas with deeper contours.
- Applications: Often used for automotive parts like frame rails and cross-members that must be durable, crash-resistant, and lightweight. In aerospace, high-pressure hydroforming shapes lightweight structural components like engine mounts and fuselage supports, which need to withstand high stress without adding weight.
Advantages:
- Highly precise shapes: Achieves complex forms with minimal defects, ideal for safety-critical parts.
- Weight savings: Enables thinner, high-strength components for fuel-efficient designs.
2. Low-Pressure Hydroforming
Low-pressure hydroforming applies lower hydraulic pressures combined with axial force to shape tubes. This method is suitable for less complex shapes and parts requiring minimal material deformation.
- Process: Hydraulic pressure gently expands the tube, often with minimal deformation, allowing for smoother curves without sharp angles. Axial force may still be used, but at a lower level.
- Applications: Frequently used in exhaust systems where airflow needs smooth curves and controlled wall thickness. Bicycle frames also benefit from low-pressure hydroforming, providing strength with light weight without significant material stress.
Advantages:
- Lower equipment costs: Less pressure means reduced wear on machinery, which translates into savings.
- Preservation of material properties: Reduced pressure prevents stress-related defects, preserving the tube’s original properties.
3. Hybrid Hydroforming
Hybrid hydroforming combines hydraulic and mechanical forces to form complex geometries and control wall thickness with even more precision.
- Process: Mechanical presses or punches apply force alongside hydraulic pressure, optimizing material flow and enabling control over depth and thickness.
- Applications: Ideal for high-performance applications like bicycle frames with variable wall thickness for specific weight distribution and medical device tubing that requires tight dimensional tolerances and durability.
Advantages:
- Superior precision and flexibility: Combines benefits of both hydraulic and mechanical methods, allowing for complex shapes with variable wall thickness.
- Adaptability: Suited for both straightforward and intricate designs.
4. Warm Hydroforming
Warm hydroforming involves preheating the tube to increase material ductility, making it easier to form high-strength metals like titanium and advanced high-strength steels (AHSS).
- Process: Metal tubes are heated just enough to increase pliability without affecting strength. This allows more challenging materials to be shaped effectively without cracking.
- Applications: High-strength automotive safety parts (e.g., crash beams) and aerospace components that must remain lightweight yet resist extreme forces.
Advantages:
- Enhanced material versatility: Enables use of tougher materials like AHSS or titanium.
- Increased ductility: Makes it possible to shape metals that would normally crack or thin under hydroforming pressures.
5. Hydro-Mechanical Forming
In hydro-mechanical forming, hydraulic pressure and a mechanical punch work in tandem, allowing for deep draws and intricate forms that are difficult to achieve with standard hydroforming.
- Process: A mechanical punch initiates tube deformation, followed by hydraulic pressure that completes the shape formation, ideal for parts with deep cavities.
- Applications: Used for chassis components in the automotive industry, where deep contours are necessary for structural integrity.
Advantages:
- Deeper shapes: Achieves more intricate geometries than pure hydroforming alone.
- Controlled material flow: Ensures uniform wall thickness across deep shapes.
6. Explosive Hydroforming
Explosive hydroforming generates hydraulic pressure through a controlled explosion in a water-filled chamber, allowing large and ultra-high-strength parts to be shaped quickly.
- Process: The explosive shockwave deforms the tube to match the die, producing shapes that require extreme forces not achievable with standard hydraulic systems.
- Applications: Common in defense and aerospace for large, heavy-duty components like aircraft fuselage parts and pressure vessels in the nuclear industry.
Advantages:
- Ability to form high-strength materials: Produces parts that withstand immense stress.
- Effective for large components: Suitable for parts that require large-scale forming without standard equipment limitations.
Applications and Industry Use
Automotive Industry: Each type of tubular hydroforming is valuable in automotive manufacturing. High-pressure hydroforming creates lightweight yet rigid chassis frames and crash structures. Low-pressure methods are used in exhaust systems to optimize flow and reduce emissions, while warm hydroforming allows for the creation of durable safety components from high-strength materials.
Aerospace: Warm and high-pressure hydroforming are crucial in aerospace for producing lightweight, high-strength components. These parts need to withstand high stress without adding unnecessary weight, such as engine mounts, fuselage supports, and wing reinforcements.
Sports Equipment and Bicycles: Low-pressure and hybrid hydroforming are ideal for bicycle frames and sports equipment. They allow for variable wall thicknesses and shapes that provide strength and flexibility while keeping weight low. In applications like hiking poles and outdoor gear, hydroformed tubes offer durability and resistance to impact.
Construction and Architecture: Low-pressure and hybrid hydroforming are often used in construction for producing structural beams and support columns. Explosive hydroforming is used to create large architectural components with unique geometries, which are often desired in modern construction for both function and aesthetic appeal.
Conclusion
Each type of tubular hydroforming offers unique capabilities suited to specific applications. High-pressure hydroforming allows for complex, high-strength automotive and aerospace parts. Low-pressure hydroforming is ideal for smoother, gentler shaping needed in exhaust systems and structural elements, while hybrid and warm hydroforming open up possibilities for materials like titanium and advanced high-strength steels.
Understanding these different methods enables manufacturers to select the most appropriate type of hydroforming for their applications, optimizing for strength, weight, cost, and complexity. As industries increasingly demand lightweight, high-performance components, tubular hydroforming will continue to evolve and expand, driven by innovations in material science and equipment technology.
Applications of Tubular Hydroforming
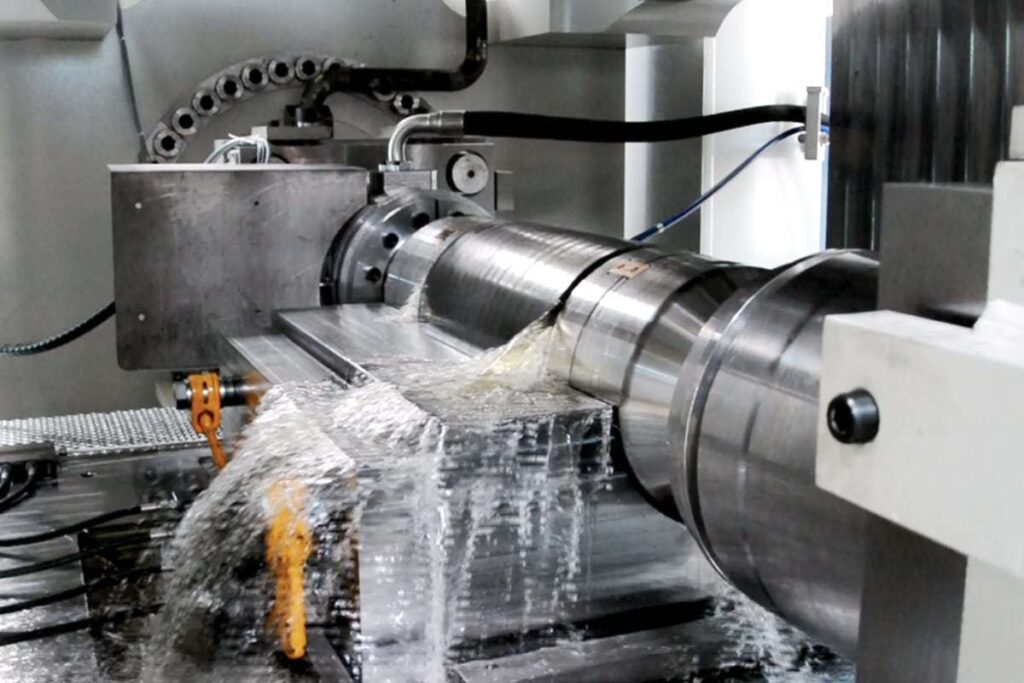
Tubular hydroforming is a revolutionary manufacturing process used to create lightweight, high-strength components with complex geometries. The process, which uses high-pressure hydraulic fluid to expand and shape metal tubes within a die, has broad applications across various industries, including automotive, aerospace, construction, sports equipment, and energy. The versatility, material efficiency, and ability to create complex parts in a single operation have made tubular hydroforming invaluable in many high-performance applications.
This article explores the applications of tubular hydroforming in different sectors, highlighting how the process contributes to safety, efficiency, and innovative design across multiple fields.
1. Automotive Industry
The automotive industry is one of the largest and most established users of tubular hydroforming technology. Manufacturers rely on this process to produce components that are lightweight, structurally robust, and cost-effective, meeting the automotive sector’s demands for fuel efficiency, performance, and crash safety.
1.1 Chassis and Frame Components
One of the primary applications of tubular hydroforming in automotive manufacturing is in creating chassis and frame components. The process allows for the formation of frame rails, engine cradles, and subframes with intricate shapes and uniform thickness, which are critical for vehicle structural integrity and crash safety.
- Frame Rails: Hydroformed frame rails provide a strong yet lightweight foundation for vehicle bodies, allowing cars to absorb impact forces more effectively in a collision. This leads to safer vehicles with enhanced crash performance.
- Engine Cradles and Crossmembers: These components benefit from tubular hydroforming due to their need for precise geometry and high strength. Hydroforming allows for complex cross-sectional shapes that increase rigidity without adding weight.
1.2 Crash Structures and Impact Absorption Components
Hydroformed components play a key role in automotive crash structures, where their high-strength, seamless construction helps absorb impact energy, reducing the force transferred to occupants during a crash.
- Side-Impact Beams and Bumper Reinforcements: Advanced high-strength steels (AHSS) are commonly hydroformed into side-impact beams and bumpers, providing excellent resistance to bending and crushing forces during a collision. This improves overall safety ratings by enabling components to absorb and dissipate crash forces effectively【203†source】.
1.3 Exhaust Systems
Hydroformed tubular components are widely used in exhaust systems, including exhaust manifolds and exhaust pipes. The seamless, smooth surfaces created by hydroforming improve airflow, reducing backpressure and optimizing exhaust gas flow for better engine performance.
- Exhaust Manifolds: Hydroformed exhaust manifolds are designed to be lightweight and corrosion-resistant, helping reduce vehicle weight while maintaining durability. These components are shaped for optimal airflow, contributing to fuel efficiency and emissions reduction【202†source】.
2. Aerospace Industry
In the aerospace industry, tubular hydroforming is valued for its ability to create lightweight yet strong components that can withstand extreme environmental conditions and stress. This industry prioritizes weight reduction to improve fuel efficiency and increase payload capacity, making hydroforming an ideal solution for critical components.
2.1 Fuselage and Structural Components
Aerospace components such as fuselage supports, wing spars, and engine mounts benefit from hydroforming’s capability to produce seamless, lightweight, and structurally sound parts.
- Wing and Fuselage Supports: Hydroformed tubular components are used within aircraft wings and fuselage frames, where they provide structural stability while minimizing weight. The complex shapes achievable through hydroforming allow for optimized load distribution, which is essential for aerodynamic efficiency and safety【200†source】.
2.2 Hydraulic and Pneumatic Tubing
The process is also used to create tubular systems for hydraulic and pneumatic applications within aircraft, such as fuel lines, ventilation ducts, and other fluid systems. These tubes require precise internal and external geometries for efficient fluid flow, making hydroforming an ideal manufacturing method.
- Fuel and Hydraulic Lines: Hydroformed tubes in aircraft fuel and hydraulic systems ensure smooth and efficient fluid transfer, reducing turbulence and pressure losses in the system.
2.3 Engine Components
Hydroforming also plays a role in aerospace engine manufacturing. Components like exhaust ducts and turbine housings require high strength and precision, as they must endure extreme temperatures and pressures.
- Exhaust Ducts and Turbine Components: The durability and heat resistance of hydroformed steel and titanium tubes make them suitable for critical engine parts that demand reliability under intense operating conditions
3. Energy and Power Generation
In the energy and power sectors, tubular hydroforming is used for components that need to withstand high pressures, corrosion, and temperature fluctuations. These applications include heat exchangers, pipelines, and pressure vessels commonly used in oil and gas, nuclear, and renewable energy systems.
3.1 Heat Exchangers
Hydroformed steel tubes are a preferred choice for heat exchangers in power plants and industrial systems, where they facilitate efficient heat transfer between fluids. The seamless construction achieved through hydroforming enhances thermal conductivity while reducing the risk of leaks.
- High-Performance Heat Exchangers: Hydroforming allows for the production of complex tubular shapes required in heat exchanger assemblies. The seamless design minimizes the chances of leakage and increases heat transfer efficiency, particularly in nuclear and fossil-fuel power plants.
3.2 Pipelines and Fluid Transfer
The oil and gas industry utilizes hydroformed tubular components for pipelines and fluid transfer systems due to their durability and ability to withstand high-pressure conditions. These pipelines are designed to transport fluids and gases over long distances and under extreme conditions.
- Oil and Gas Pipelines: Hydroformed pipelines are less prone to cracking and corrosion, ensuring safe and efficient fluid transport. The smooth, uniform surfaces achieved through hydroforming reduce drag and minimize pressure losses within the pipeline, enhancing overall efficiency【199†source】.
3.3 Renewable Energy Equipment
Tubular hydroforming is increasingly being applied in the renewable energy sector for creating structural components in wind turbines and solar panel mounts. These components benefit from hydroforming’s strength-to-weight ratio and ability to withstand harsh outdoor conditions.
- Wind Turbine Supports: Hydroformed steel tubes provide the necessary strength and stability for wind turbine structures, supporting the blades and tower against high wind loads and vibrations【203†source】.
4. Construction and Architecture
Tubular hydroforming has applications in the construction and architectural sectors, where it is used to create structural supports and decorative elements. Architects and engineers leverage the process to design structures that are both functional and visually appealing.
4.1 Structural Beams and Supports
Hydroformed steel beams and tubular columns offer increased load-bearing capacity, making them ideal for building structures, bridges, and other load-bearing applications. The ability to form strong, lightweight structures reduces material usage and simplifies construction.
- Building Columns and Frameworks: The ability to create custom shapes with hydroforming allows architects to design structural columns with unique profiles that meet both aesthetic and functional requirements. These columns are often seen in modern architectural designs where both strength and aesthetics are paramount【200†source】.
4.2 Custom Architectural Elements
Tubular hydroforming allows for complex geometric designs, making it popular in decorative architecture. Architects use hydroformed steel tubes to create unique shapes for building facades, railings, and interior design elements.
- Aesthetic Structures: In applications like interior stair railings or modern building facades, hydroformed tubes provide a sleek, continuous design with smooth curves, creating a refined appearance that enhances architectural appeal.
5. Medical Devices and Healthcare Equipment
The medical field uses hydroformed tubular components in equipment and devices that require precision, durability, and sanitation. The process is used to produce surgical instruments, equipment housings, and medical tubing with specific dimensional accuracy and surface finish requirements.
5.1 Surgical Instruments and Trays
Hydroformed stainless steel components are commonly used in the production of surgical instruments and trays, where durability and ease of sterilization are crucial.
- Surgical Trays and Covers: Hydroformed stainless steel trays are lightweight, corrosion-resistant, and can withstand repeated sterilization cycles, making them ideal for use in sterile environments such as operating rooms【202†source】.
5.2 Equipment Housings and Frames
In addition to surgical instruments, hydroformed components are used in diagnostic equipment housings and structural supports for devices like imaging machines, where precision and strength are required.
- Diagnostic Equipment Casings: Hydroformed casings protect delicate imaging equipment from damage, ensuring consistent performance and extending equipment lifespan.
5.3 Medical Tubing
Hydroformed tubing is often used in medical devices that require precise tubing dimensions, such as in catheters, ventilators, and other respiratory devices.
- Catheter and Ventilator Tubes: Hydroforming creates smooth, consistent tubing walls, reducing the risk of turbulence and improving the flow of fluids or gases through the device.
6. Bicycles and Sports Equipment
In the sports equipment industry, particularly in bicycle manufacturing, hydroformed tubular components are valued for their lightweight, durable, and aerodynamic characteristics. Hydroforming is used to create bicycle frames, structural supports in sports gear, and equipment frames for recreational activities.
6.1 Bicycle Frames
Hydroforming provides bicycle manufacturers with the flexibility to create lightweight and durable frames that are able to withstand high stress and impact. Aluminum and steel frames are commonly hydroformed to form smooth, seamless designs that improve the aerodynamics and aesthetics of bicycles.
- Aerodynamic Designs: Tubular hydroforming allows the creation of frames with tapered or oval shapes, which reduce drag and enhance rider performance.
- Strength and Flexibility: The resulting lightweight frames balance flexibility and resilience, suitable for both high-impact mountain biking and streamlined racing.
6.2 Sports Equipment and Outdoor Gear
Beyond bicycles, tubular hydroforming is used to manufacture frames and supports for outdoor sports equipment like hiking poles, ski poles, and climbing gear. These applications benefit from the process’s ability to produce high-strength, corrosion-resistant tubes that withstand environmental stress.
- Hiking Poles: Lightweight aluminum and steel poles benefit from hydroforming for both durability and user comfort.
- Climbing Frames and Racks: Tubular hydroformed components provide a strong, resilient structure for carrying loads and handling wear in extreme conditions.
Low-Pressure Tubular Hydroforming
Low-pressure tubular hydroforming is a specialized variation of the hydroforming process that uses lower hydraulic pressures to shape metal tubes. Unlike high-pressure hydroforming, which typically requires pressures exceeding 10,000 psi, low-pressure hydroforming operates at pressures below 5,000 psi. This method is preferred for simple shapes and applications where minimal material deformation is required. It offers a more cost-effective and gentle approach to shaping, making it ideal for parts that need consistent wall thickness and smooth surfaces without extensive stretching.
This article delves into the fundamentals of low-pressure tubular hydroforming, including its process steps, applications, benefits, limitations, and comparison with high-pressure hydroforming.
1. Fundamentals of Low-Pressure Tubular Hydroforming
In low-pressure tubular hydroforming, internal hydraulic pressure is applied inside a metal tube, causing it to expand and conform to the shape of a surrounding die. This process is suitable for materials that require gentler forming, such as certain aluminum and stainless steel alloys, which may become brittle or crack under high pressure. Low-pressure hydroforming is typically used for tubes with simpler geometries and moderate shapes that do not require deep draws or extreme deformation.
Process Steps
- Tube Preparation: A pre-cut hollow metal tube is inserted into the hydroforming press and placed within a specially designed die.
- Clamping and Sealing: The tube is clamped securely at both ends to ensure hydraulic fluid is contained inside without leakage.
- Gradual Hydraulic Pressurization: Hydraulic fluid is pumped into the tube, gradually increasing the internal pressure. In low-pressure hydroforming, this pressure is relatively low compared to high-pressure methods.
- Forming the Tube: The hydraulic pressure causes the tube to gently expand against the die, taking on the desired shape without excessive stretching.
- Pressure Release and Part Removal: Once the tube has conformed to the die, the pressure is released, and the formed part is removed.
2. Characteristics and Mechanisms of Low-Pressure Hydroforming
Low-pressure hydroforming is characterized by a gentle shaping mechanism that minimizes material strain and reduces the risk of defects. This process is particularly useful when working with thin-walled tubes or materials with limited ductility, as it helps avoid issues like wrinkling or tearing that can arise with high-pressure hydroforming.
- Controlled Material Flow: Low-pressure hydroforming applies force evenly along the tube, allowing for controlled material distribution. This helps maintain consistent wall thickness, which is especially important for parts that require precise dimensions.
- Reduced Surface Defects: The gentler hydraulic pressures used in this process minimize the likelihood of surface flaws, resulting in a smooth, high-quality surface finish.
Material Compatibility
Low-pressure hydroforming is compatible with mild steel, aluminum, stainless steel, and certain high-strength low-alloy (HSLA) steels. It is especially well-suited to materials that have low ductility and cannot withstand the intense pressures of high-pressure hydroforming.
3. Advantages of Low-Pressure Tubular Hydroforming
The low-pressure variant of tubular hydroforming has unique advantages, particularly in terms of cost-efficiency, reduced material strain, and versatility.
3.1 Cost Efficiency
Low-pressure hydroforming requires less robust equipment and has lower maintenance costs due to the reduced stress on machinery. This is advantageous for manufacturers who produce low-volume parts or for applications where the investment in high-pressure equipment would be unnecessary.
- Reduced Equipment Wear: Lower pressures reduce wear and tear on both hydraulic systems and dies, prolonging the lifespan of these components and reducing maintenance costs.
- Lower Tooling Costs: The dies and clamping mechanisms used in low-pressure hydroforming are less costly to produce, making the process more affordable for manufacturers working with simpler part designs.
3.2 Minimal Material Deformation
The gentle nature of low-pressure hydroforming minimizes material deformation, making it ideal for thin-walled parts or materials prone to cracking. By reducing the amount of strain on the material, the process maintains consistent wall thickness and avoids issues like wrinkling or buckling, which are common challenges in more aggressive forming techniques.
- Enhanced Surface Finish: The reduced pressure used in this process helps maintain the natural finish of the material, minimizing surface defects and improving the overall quality of the part.
- Controlled Thickness: Low-pressure hydroforming allows for precise control over material distribution, particularly beneficial for parts where uniform thickness is critical for performance.
3.3 Versatility in Design
Low-pressure hydroforming is highly versatile and can be applied to create a wide range of part geometries. Although it does not support extreme deformation, it is suitable for moderate shapes and parts with curves or gradual contours.
- Automotive Exhaust Components: Low-pressure hydroforming is commonly used to create exhaust systems with smooth bends, improving exhaust flow and reducing backpressure in automotive applications.
- Furniture and Decorative Components: The process’s gentle shaping and ability to produce clean lines make it suitable for furniture and architectural elements, where aesthetics and structural integrity are important.
4. Applications of Low-Pressure Tubular Hydroforming
Low-pressure hydroforming is applied in various industries, especially where smooth, seamless tubular structures are required. The following sections outline some of the main applications.
4.1 Automotive Industry
In the automotive sector, low-pressure hydroforming is used to create parts that require smooth contours, consistent wall thickness, and improved fluid dynamics.
- Exhaust Manifolds and Pipes: Low-pressure hydroforming is ideal for shaping exhaust components, as the process allows for smooth bends that reduce turbulence, improve gas flow, and increase engine efficiency.
- Heat Shields and Structural Braces: Some structural components, such as heat shields and braces, benefit from low-pressure hydroforming due to its ability to maintain consistent material thickness, enhancing thermal resistance and durability.
4.2 Furniture and Decorative Elements
For furniture and decorative applications, low-pressure hydroforming is an excellent choice due to its precision, aesthetic appeal, and ability to produce thin-walled components that remain strong and visually pleasing.
- Furniture Frames: Tubular components in furniture, such as table and chair legs or frames, are commonly produced using low-pressure hydroforming. The process allows for the creation of intricate designs that are both lightweight and strong.
- Architectural and Decorative Tubes: The smooth finish and controlled wall thickness achieved through low-pressure hydroforming make it suitable for decorative architectural elements like handrails and metal frames used in building facades.
4.3 Bicycle Manufacturing
In bicycle manufacturing, hydroformed aluminum tubes are highly desirable for their strength-to-weight ratio, durability, and ability to form ergonomic shapes. Low-pressure hydroforming provides the necessary control over tube dimensions without over-stretching the material.
- Bicycle Frames: Low-pressure hydroforming allows for the creation of streamlined and lightweight bicycle frames, optimizing material distribution for performance without adding unnecessary weight. This is particularly advantageous for high-performance road and mountain bikes, where strength, durability, and weight are critical.
4.4 Industrial and Medical Tubing
Low-pressure hydroforming is also applied in industrial tubing and medical devices, where precision and smooth surfaces are essential.
- Hydraulic and Pneumatic Tubes: For applications in machinery or fluid systems, low-pressure hydroforming creates seamless, durable tubes with consistent wall thickness that withstand high pressures and minimize the risk of leaks.
- Medical Device Tubing: Low-pressure hydroforming can produce tubing for medical devices such as catheters and respiratory systems, where precise dimensions and smooth surfaces improve patient safety and comfort.
5. Comparison with High-Pressure Hydroforming
While both high-pressure and low-pressure hydroforming use hydraulic pressure to shape tubes, they serve different purposes and are suited to different types of parts.
5.1 High-Pressure Hydroforming
High-pressure hydroforming is suited for complex shapes and deep-drawn parts that require significant material deformation. It operates at pressures exceeding 10,000 psi and is typically used in automotive chassis components and aerospace structural parts.
- Advantages: Ideal for parts requiring intricate shapes and high strength; capable of achieving deep draws and sharp angles.
- Limitations: Higher equipment and tooling costs, increased risk of material defects due to high strain.
5.2 Low-Pressure Hydroforming
Low-pressure hydroforming, by contrast, is more appropriate for simple shapes that do not require extreme deformation. It offers advantages in terms of cost-efficiency, material consistency, and surface quality.
- Advantages: Lower costs, reduced risk of material failure, and minimal equipment wear. Suitable for parts requiring moderate shaping and controlled wall thickness.
- Limitations: Limited ability to create deep-drawn or highly complex shapes.
6. Limitations of Low-Pressure Hydroforming
Despite its advantages, low-pressure hydroforming does have some limitations that restrict its applicability.
6.1 Limited Shape Complexity
The lower pressures used in this process limit the range of geometries that can be achieved, making it less suitable for parts with sharp angles, tight curves, or deep draws.
- Design Restrictions: Parts that require complex shapes may not be feasible with low-pressure hydroforming, as the process cannot create extreme deformations without compromising material integrity.
Hybrid Hydroforming Processes combine elements of both traditional hydroforming (low and high pressure) and mechanical metal-forming methods to create components with complex geometries, variable wall thickness, and high strength-to-weight ratios. By blending techniques such as mechanical pressing, stamping, or stretch forming with hydroforming, hybrid hydroforming processes offer a unique solution for creating deep-drawn or multi-dimensional parts that would be difficult to achieve through standard hydroforming alone. These processes are especially valuable in industries like automotive, aerospace, and construction, where the demand for lightweight yet durable components is constantly increasing.
This article provides an in-depth overview of hybrid hydroforming processes, exploring their characteristics, advantages, applications, and the types of hybrid methods currently in use.
Overview of Hybrid Hydroforming
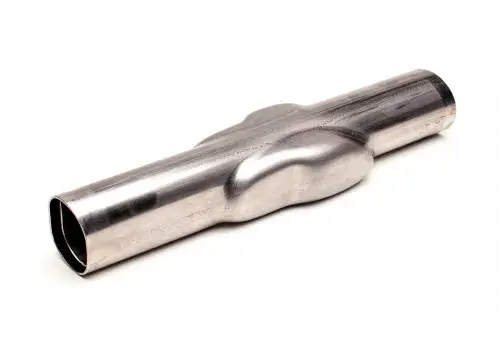
In traditional hydroforming, hydraulic fluid is used to press a metal blank or tube into a die, allowing it to take on the desired shape. In hybrid hydroforming, additional mechanical forces—such as axial compression, punches, or mechanical stamping—are applied in conjunction with hydraulic pressure to enhance material flow control and achieve greater part complexity. This combination enables manufacturers to achieve precise control over material thickness, dimensional accuracy, and surface finish.
How Hybrid Hydroforming Works
- Mechanical Assistance: Hybrid hydroforming involves the use of mechanical presses or punches that work alongside hydraulic pressure to initiate and guide the shaping process. This additional force assists in stretching or forming the material into specific features, like deep recesses or flanges.
- Reduced Pressure Requirements: Since mechanical forces are used in conjunction with hydraulic pressure, hybrid hydroforming can often operate at lower pressures than traditional high-pressure hydroforming, reducing equipment wear and increasing the lifespan of tooling.
Hybrid hydroforming processes are adaptable to both sheet and tubular hydroforming, enabling manufacturers to work with a wider range of metals, including high-strength alloys that may not be suitable for pure hydroforming.
2. Types of Hybrid Hydroforming Processes
Several types of hybrid hydroforming processes have been developed to cater to different material characteristics, geometries, and industrial applications. Each type has unique attributes, which allow manufacturers to fine-tune the process for specific part designs.
2.1 Hydro-Mechanical Forming
Hydro-mechanical forming is a widely used hybrid process that combines mechanical pressing with hydraulic pressure. A mechanical punch initiates the deformation of the metal blank or tube, followed by hydraulic pressure to complete the forming process. This hybrid technique provides greater control over material flow, reducing the likelihood of thinning or tearing, which can be a concern in deep-drawn parts.
- Applications: Hydro-mechanical forming is ideal for automotive chassis components, deep-drawn engine cradles, and fuselage frames in aerospace that require deep shapes and tight tolerances.
- Benefits: This process allows for deeper draws and sharper radii than traditional hydroforming, enabling the production of parts with complex cross-sections and multiple integrated features, such as flanges and reinforcing ribs.
2.2 Hydroforming with Pre-Forming
In this process, pre-forming techniques—such as stamping or stretch forming—are used to create an initial shape before applying hydraulic pressure to complete the part. Pre-forming establishes the part’s general geometry and wall thickness, while hydroforming finalizes the shape with improved dimensional accuracy and surface quality.
- Applications: Hydroforming with pre-forming is particularly useful for automotive body panels and structural supports that need a combination of deep contours and smooth, complex curves.
- Benefits: The pre-forming stage reduces the strain on the material, allowing for lower pressures during hydroforming and decreasing the risk of material failure. This approach is highly effective for parts requiring consistent wall thickness and sharp angles without excessive thinning.
2.3 Hydroforming with Axial Feed
In hydroforming with axial feed, axial forces are applied at the ends of the tube, pushing material into the die during the forming process. This technique is often combined with hydraulic pressure to create long, complex parts with uniform thickness. Axial feed helps control material flow, allowing for better distribution and reducing the likelihood of tearing or thinning in critical areas.
- Applications: Automotive frame rails, crash-resistant beams, and subframes are commonly made with hydroforming and axial feed due to the need for extended, strong shapes that can withstand impact.
- Benefits: The addition of axial feeding prevents thinning and allows for better control over the wall thickness along the length of the component, ensuring that the finished product meets strict safety and durability standards.
2.4 Warm Hybrid Hydroforming
Warm hybrid hydroforming uses controlled heating of the metal tube or blank before or during the forming process to increase the material’s ductility. Combining elevated temperature with hydraulic pressure and mechanical assistance allows for more significant deformation of materials like high-strength steel and titanium, which are prone to cracking under standard conditions.
- Applications: Warm hybrid hydroforming is used in automotive crash structures, aerospace engine mounts, and aircraft wing spars, where components need to withstand high impact without losing structural integrity.
- Benefits: The addition of heat increases the formability of high-strength materials, reducing the risk of cracks and allowing manufacturers to use tougher alloys that would typically require more costly forming techniques.
3. Advantages of Hybrid Hydroforming Processes
Hybrid hydroforming offers numerous advantages over traditional hydroforming and standalone mechanical methods, making it highly suitable for manufacturing complex, high-strength components.
3.1 Enhanced Formability and Material Control
Combining mechanical and hydraulic forces gives manufacturers greater control over material flow, thickness distribution, and shape accuracy. This is particularly useful for components that require complex features, such as deep draws, variable cross-sections, or integrated flanges.
- Improved Material Flow: Mechanical assistance during the forming process ensures that material flows more evenly into the die, reducing thinning and preventing defects like tearing or wrinkling.
- Reduced Material Stress: By distributing force between hydraulic and mechanical sources, hybrid hydroforming reduces strain on the material, enabling the use of high-strength metals like advanced high-strength steels (AHSS).
3.2 Increased Design Flexibility
Hybrid hydroforming opens up possibilities for creating components with multi-dimensional geometries and integrated features in a single forming operation. Manufacturers can produce parts with complex cross-sections, varying thicknesses, and intricate curves that would be difficult to achieve with conventional methods.
- Integrated Features: By combining mechanical and hydraulic shaping, manufacturers can integrate features such as flanges, stiffeners, and brackets directly into the part, eliminating the need for secondary operations.
- Variable Wall Thickness: Hybrid processes enable the creation of parts with customized wall thicknesses, optimizing strength-to-weight ratios and enhancing performance for specific applications.
3.3 Cost and Production Efficiency
Hybrid hydroforming processes allow for the production of complex parts with fewer forming steps and simplified tooling, reducing manufacturing costs and cycle times. The combination of techniques also reduces the likelihood of defects, minimizing scrap and rework requirements.
- Lower Tooling Costs: Hybrid hydroforming often requires simpler dies than conventional methods, especially when pre-forming techniques are used, reducing initial setup costs.
- Faster Production: By integrating multiple forming steps into a single operation, hybrid hydroforming streamlines the production process, making it ideal for high-volume applications where speed is critical.
4. Applications of Hybrid Hydroforming Across Industries
Hybrid hydroforming processes are employed across a wide range of industries, particularly in applications where lightweighting, strength, and complex shapes are essential.
4.1 Automotive Industry
In the automotive sector, hybrid hydroforming is used extensively to produce lightweight, high-strength structural components that enhance vehicle safety and fuel efficiency. Key applications include:
- Crash Structures and Reinforcements: Hybrid hydroforming produces impact-resistant components, such as side-impact beams and bumper reinforcements, which help absorb energy during collisions.
- Chassis Frames and Engine Cradles: Parts that require intricate cross-sections, high strength, and dimensional accuracy benefit from hybrid hydroforming, as it allows for deep shapes and integrated flanges that improve overall structural integrity.
4.2 Aerospace Industry
Aerospace manufacturers rely on hybrid hydroforming to create lightweight yet robust components for aircraft that can withstand extreme loads and environmental conditions. Key applications include:
- Fuselage and Wing Supports: Hybrid hydroforming allows for the creation of complex, strong supports within aircraft fuselage frames and wings, enhancing structural stability while reducing weight.
- Engine Components: Warm hybrid hydroforming enables the use of high-strength materials like titanium for aerospace engine components, where the combination of heat and mechanical force helps prevent cracking.
4.3 Medical and Healthcare Equipment
In the medical field, hybrid hydroforming produces precision tubing and structural components for medical devices. This is particularly useful in applications that require thin-walled tubing with exact dimensions and smooth finishes, such as:
- Catheter and Respiratory Tubes: Hybrid hydroforming ensures precise tubing dimensions and consistent wall thickness, essential for fluid and air transfer in medical devices.
- Surgical Instruments and Diagnostic Equipment Frames: Complex yet lightweight frames benefit from the strength and dimensional accuracy provided by hybrid hydroforming.
4.4 Energy and Power Generation
Hybrid hydroforming is also used in the energy industry to create high-strength tubing and structural supports for power plants, pipelines, and renewable energy applications in Hybrid Hydroforming Processes within energy and power generation sectors include high-strength tubing and support structures for power plants, pipelines, and renewable energy installations. These industries demand durable and efficient materials capable of withstanding extreme pressures and environmental conditions.
5. Challenges and Limitations of Hybrid Hydroforming
While hybrid hydroforming offers several advantages, it is not without its challenges. These limitations can affect its feasibility for certain applications and industries.
5.1 High Initial Investment
Hybrid hydroforming requires specialized equipment capable of applying both mechanical and hydraulic forces simultaneously, which often results in higher upfront costs compared to standard hydroforming or traditional mechanical forming processes.
- Specialized Tooling: Creating tools that can withstand the combined forces of hydraulic and mechanical pressure can be costly, especially for applications that require custom tooling.
- Maintenance Costs: The added complexity of hybrid systems can also lead to higher maintenance costs and longer downtime, as more sophisticated equipment is generally required.
5.2 Material Limitations
While hybrid hydroforming enhances the formability of certain materials, not all metals are suitable for this process. High-strength materials, especially those prone to cracking or brittleness, may still require careful consideration regarding process pressures and temperatures.
- Risk of Material Failure: Materials like high-carbon steels, while strong, may struggle to retain their structural integrity under combined pressure forces, limiting the materials that can be effectively used in hybrid hydroforming applications.
5.3 Complexity of Process Control
Hybrid hydroforming requires precise coordination between hydraulic and mechanical forces, which can complicate the control system and increase the risk of process errors.
- Process Optimization: Maintaining consistent pressure levels, timing the application of mechanical forces, and managing temperature in warm hybrid hydroforming can be challenging. Achieving optimal results often requires careful monitoring and fine-tuning, which can add time and cost to the production process.
Conclusion
Hybrid hydroforming processes offer an innovative solution for creating complex, high-strength components that meet the evolving demands of industries such as automotive, aerospace, healthcare, and energy. By combining hydraulic pressure with mechanical force and, in some cases, heat, hybrid hydroforming overcomes many of the limitations associated with traditional forming methods, allowing manufacturers to produce parts with intricate shapes, controlled wall thickness, and enhanced material properties.
Despite its advantages, hybrid hydroforming faces certain limitations, including high initial investment costs, material constraints, and complex process control. Nevertheless, as technology advances and industries continue to seek lightweight, strong, and cost-effective materials, hybrid hydroforming is poised to play an increasingly important role in modern manufacturing.
Şunu dedin:
hydroforming pressure (2000 words)
ChatGPT:
Hydroforming Pressure refers to the hydraulic pressure applied to a metal blank or tube during hydroforming processes, a technique used to shape complex, high-strength components. The pressure level in hydroforming is critical, as it directly impacts the material flow, dimensional accuracy, and mechanical properties of the final part. Hydroforming pressure varies depending on the type of hydroforming process (such as low-pressure or high-pressure hydroforming), the material properties, and the geometry of the desired part.
1. The Role of Pressure in Hydroforming
In hydroforming, hydraulic pressure acts as the driving force that deforms the metal blank or tube into a die, allowing the material to take on complex shapes that are difficult to achieve with traditional forming methods like stamping or pressing. The applied pressure causes the metal to expand, fill, and conform to the contours of the die, resulting in components with uniform thickness, high precision, and smooth surface finishes.
Key Functions of Hydroforming Pressure
- Material Flow Control: The hydraulic pressure must be sufficient to ensure that the material flows evenly within the die, preventing defects such as wrinkling, thinning, or tearing.
- Maintaining Wall Thickness: Proper pressure levels help control the material distribution, ensuring consistent wall thickness across the part. This is especially important in applications like automotive frame components or bicycle frames.
- Achieving Dimensional Accuracy: Sufficient pressure helps the material fully conform to the die shape, ensuring that the final part meets precise design specifications.
2. Factors Affecting Hydroforming Pressure
The pressure level in hydroforming processes varies based on several factors, each of which affects the material behavior and quality of the final component. Determining the optimal pressure level is essential for achieving the desired shape and structural properties without compromising the material’s integrity.
2.1 Material Properties
The choice of material has a significant impact on the required hydroforming pressure. Materials with higher ductility, like aluminum and mild steel, typically require lower pressures than high-strength steel or titanium, which are more resistant to deformation.
- Ductility and Formability: Ductile metals can expand and stretch more easily, requiring lower pressure levels to form complex shapes.
- Strength and Hardness: High-strength metals require greater pressure to achieve the same deformation as softer metals, as they resist deformation more effectively.
2.2 Part Geometry and Complexity
The geometry of the part plays a crucial role in determining the hydroforming pressure. Components with deep draws, sharp angles, or complex contours require higher pressures to ensure that the material fills all areas of the die.
- Complex Shapes: Parts with deep draws or sharp radii require higher pressures to prevent wrinkling and ensure the material fills the die completely.
- Thin-Walled Components: Thin-walled parts can be more prone to tearing, requiring lower pressure levels to avoid material failure.
2.3 Type of Hydroforming Process
The specific type of hydroforming process—low-pressure, high-pressure, or warm hydroforming—determines the typical pressure range applied. Each process has unique pressure requirements to achieve optimal part quality.
- Low-Pressure Hydroforming: Typically uses pressures below 5,000 psi, ideal for creating components with minimal deformation and shallow geometries, such as automotive exhaust systems and furniture frames.
- High-Pressure Hydroforming: Involves pressures exceeding 10,000 psi and is used for complex automotive and aerospace parts that require deep draws and intricate shapes.
- Warm Hydroforming: Applies moderate pressure combined with heat, enabling the forming of high-strength materials like AHSS and titanium with reduced risk of cracking.
3. Types of Hydroforming Processes and Associated Pressures
The pressure applied in hydroforming is specific to each type of process, which uses different techniques and pressures based on the component’s design requirements and material characteristics.
3.1 Low-Pressure Hydroforming
Low-pressure hydroforming operates at pressures below 5,000 psi and is commonly used for applications where minimal deformation is required. In this process, hydraulic pressure is applied gradually, allowing the material to conform to the die without significant stretching. Low-pressure hydroforming is ideal for creating exhaust components, furniture frames, and decorative architectural elements where complex shapes or extreme deformation are not necessary.
- Applications: Automotive exhaust pipes, furniture frames, and handrails.
- Advantages: Reduced equipment wear, cost-efficiency, and minimal risk of material tearing.
3.2 High-Pressure Hydroforming
High-pressure hydroforming requires pressure levels ranging from 10,000 to 20,000 psi or higher, depending on the material and part complexity. This high-pressure approach is essential for creating automotive chassis components, aerospace structural parts, and other applications where deep draws and complex geometries are necessary.
- Applications: Automotive frame rails, crash-resistant beams, and aerospace components.
- Advantages: Ability to achieve complex shapes, high structural integrity, and consistent wall thickness in components that require high strength and precision.
3.3 Warm Hydroforming
In warm hydroforming, moderate pressures are combined with controlled heating to improve the ductility of certain metals, such as high-strength steel and titanium. The temperature increases the material’s flexibility, allowing it to expand more easily under lower pressure than would be required at room temperature. Warm hydroforming is particularly beneficial for automotive crash structures and aircraft engine mounts, where high-strength materials are needed.
- Applications: High-strength steel crash components, aerospace engine mounts.
- Advantages: Enables the use of high-strength alloys, reduces the risk of cracking, and enhances material formability.
4. Optimizing Hydroforming Pressure for Material and Process Requirements
Determining the correct pressure is essential for the success of hydroforming processes, as it directly affects the quality and reliability of the final component. Optimizing pressure involves a combination of material testing, process modeling, and real-time adjustments.
4.1 Simulation and Process Modeling
Computer simulation and finite element modeling (FEM) are widely used to predict how different pressures affect material flow and wall thickness in hydroforming processes. Modeling allows engineers to analyze potential defects and make adjustments before production, saving time and reducing material waste.
- Finite Element Analysis (FEA): Simulation models use FEA to predict material behavior under different pressures, ensuring the optimal pressure is applied for each part’s unique geometry and material.
4.2 Real-Time Monitoring and Pressure Adjustment
Modern hydroforming presses are equipped with sensors and monitoring systems that allow for real-time pressure adjustments. These systems can detect material anomalies, such as thinning or wrinkling, and automatically adjust pressure levels to ensure consistent quality.
- Adaptive Pressure Control: By monitoring pressure throughout the process, manufacturers can make adjustments based on part requirements and material behavior, preventing defects and improving accuracy.
5. Challenges Associated with Hydroforming Pressure
While hydroforming offers numerous benefits, maintaining optimal pressure levels can be challenging, particularly in applications involving complex geometries or high-strength materials.
5.1 Risk of Material Failure
Applying too much pressure can lead to cracking, tearing, or thinning in the material, particularly with metals that have lower ductility. This is a common challenge in high-pressure hydroforming, where materials like titanium or advanced high-strength steel are used.
- Material Limits: High-strength materials often have limited ductility, increasing the likelihood of failure under extreme pressure. Warm hydroforming can help mitigate this, but it requires additional equipment and process controls.
5.2 Equipment Wear and Maintenance Costs
High-pressure hydroforming requires robust equipment capable of withstanding extreme forces, which can increase wear and tear on presses, dies, and hydraulic systems. Regular maintenance is required to ensure consistent performance and avoid costly downtime.
- Maintenance Requirements: High-pressure systems, in particular, require regular inspections and part replacements to prevent equipment failure, especially in high-volume production environments.
6. Future Trends in Hydroforming Pressure Technology
Advancements in hydroforming technology are continually improving the precision and efficiency of pressure application, enabling manufacturers to produce more complex, high-performance components. Some emerging trends include:
6.1 Enhanced Simulation and Predictive Modeling
New developments in simulation and modeling software are allowing engineers to more accurately predict pressure requirements and material behavior, leading to more efficient production setups.
- Advanced FEA and AI Integration: The integration of artificial intelligence with FEA enables more complex predictive modeling, allowing manufacturers to simulate material flow and predict pressure-related defects with greater accuracy.
6.2 Hybrid Hydroforming Techniques
Hybrid hydroforming combines hydraulic pressure with mechanical force to reduce the overall pressure required, improving material formability and reducing equipment wear.
- Combined Hydraulic and Mechanical Pressing: By integrating mechanical forming methods with hydraulic pressure, manufacturers can achieve complex shapes at lower pressures, reducing the risk of material failure and increasing process efficiency.
Conclusion
Hydroforming pressure plays a pivotal role in shaping high-quality metal components, impacting everything from material flow and wall thickness to dimensional accuracy. Whether using low-pressure hydroforming for minimal deformation or high-pressure hydroforming for intricate parts, the correct pressure application is critical to achieving optimal results. Factors such as material properties, part geometry, and process type mustto achieve optimal results requires precise calibration, factoring in material properties, part geometry, and process type. Innovations in simulation technologies, adaptive control systems, and hybrid processes are continuously refining pressure application, enabling hydroforming to tackle increasingly challenging part designs. Future developments in predictive modeling and AI integration promise to make pressure regulation more efficient, reducing material waste, costs, and equipment wear while improving part quality.
Understanding the importance and complexities of hydroforming pressure helps manufacturers select the best approach, allowing for the creation of lighter, stronger, and more complex components across industries from automotive and aerospace to medical and construction. By leveraging the optimal pressure settings tailored to specific materials and design requirements, hydroforming will continue to advance as a critical manufacturing technique in modern engineering.
Hydroforming is a metal forming technique that uses high-pressure hydraulic fluid to shape metal sheets or tubes into complex, high-strength parts. The hydraulic pressure applied in this process is critical to achieving the desired part geometry, material consistency, and structural integrity of the finished component. In hydroforming, pressure is not only the force that shapes the material but also a key factor in determining the quality and properties of the final part. This makes understanding the role of pressure essential for optimizing hydroforming processes across industries, including automotive, aerospace, construction, and medical devices.
This article explores the various ways in which pressure influences hydroforming, from material behavior and process efficiency to the advantages and limitations associated with different pressure levels. Additionally, it covers how engineers determine optimal pressures for different materials and parts and recent innovations in pressure control technology.
1. Importance of Pressure in Hydroforming
Hydroforming relies on hydraulic pressure to deform metal into desired shapes. The applied pressure enables manufacturers to achieve seamless components with complex geometries and uniform wall thicknesses. Proper pressure application can significantly reduce or eliminate defects like wrinkling, tearing, and uneven thickness, improving the quality and reliability of the part.
Functions of Pressure in Hydroforming
- Shape Formation: Pressure is the primary force that causes the material to expand and take the shape of the die, making it possible to achieve complex geometries that traditional forming methods cannot accomplish.
- Material Flow Control: Correct pressure distribution ensures even material flow, which is crucial for preventing issues like localized thinning or excessive thickness in certain areas.
- Dimensional Accuracy: The application of consistent pressure helps the material fully conform to the die, improving the dimensional accuracy of the final part.
2. Pressure Requirements for Different Hydroforming Processes
Different types of hydroforming processes—such as sheet hydroforming, tube hydroforming, and explosive hydroforming—require varied pressure levels depending on the material type, part geometry, and the forming goals. Each type of process requires specific pressure levels to achieve optimal part quality without risking damage to the material or equipment.
2.1 Sheet Hydroforming
In sheet hydroforming, metal sheets are deformed into dies using hydraulic pressure, usually with a flexible diaphragm that presses the sheet into the mold. This process is typically low-pressure and operates at pressures of around 3,000 to 10,000 psi. It is ideal for thin metal sheets and parts with less extreme geometries.
- Applications: Automotive body panels, lightweight aerospace components, and appliance casings.
- Pressure Role: Sufficient pressure allows the sheet to conform to the die without tearing, wrinkling, or thinning, ensuring a consistent wall thickness and smooth surface finish.
2.2 Tube Hydroforming
In tube hydroforming, hollow tubes are expanded inside a die under hydraulic pressure, allowing for the creation of hollow, seamless structures. This process is used to make complex, multi-dimensional parts such as automotive chassis components, bicycle frames, and industrial piping.
- Pressure Range: High-pressure hydroforming for tubes typically requires pressures above 10,000 psi, with some applications exceeding 20,000 psi, especially for materials that need substantial deformation.
- Pressure Role: Pressure in tube hydroforming controls both material expansion and wall thickness. High pressures are required to form intricate, deep geometries and ensure that the material conforms fully to the die.
2.3 Explosive Hydroforming
Explosive hydroforming uses a controlled explosion in a water-filled chamber to generate extremely high-pressure shockwaves. This technique is used for very large parts or parts with extreme geometries that cannot be produced by conventional methods.
- Applications: Large aircraft fuselage sections, pressure vessels, and energy industry components.
- Pressure Role: The shockwave pressure enables the formation of ultra-high-strength materials and large parts with complex shapes. This type of hydroforming is particularly useful for high-strength materials like titanium and advanced steel alloys that are difficult to form with conventional pressures.
3. Determining Optimal Pressure Levels
The optimal pressure for hydroforming is determined by various factors, including material properties, part geometry, thickness, and required tolerances. Engineers use finite element modeling (FEM) and computer simulations to predict how different pressures affect the material and to identify the best pressure for achieving the desired results without risking material damage or equipment strain.
3.1 Material Properties
Different materials respond differently to pressure in hydroforming, making material properties one of the most important factors in determining the optimal pressure.
- Ductility and Formability: Materials with high ductility, such as aluminum and mild steel, require lower pressures, while harder materials like high-strength steel need higher pressures to achieve similar deformation.
- Strength and Hardness: High-strength materials resist deformation more effectively and, as a result, require higher pressures for hydroforming to shape them accurately without causing defects like wrinkling or tearing.
3.2 Part Geometry
The shape and complexity of the part significantly influence the required pressure. Parts with deep draws or sharp contours need higher pressures to fill out the die and avoid defects.
- Deep Draws and Complex Shapes: Higher pressures are often needed for parts with deep sections or intricate curves, as these require more force to push the material into all areas of the die.
- Uniform Wall Thickness: Maintaining consistent wall thickness across the entire part requires precise control over pressure levels, as excessive pressure can lead to thinning, while insufficient pressure can result in incomplete fills.
4. Pressure-Related Challenges in Hydroforming
While pressure is essential for achieving accurate part shapes, improper application or excessive pressure levels can introduce defects and compromise the quality of the hydroformed part.
4.1 Material Tearing and Thinning
Applying too much pressure during hydroforming can cause material tearing or thinning, particularly in areas where the metal is stretched significantly. Thinning can reduce the durability and structural integrity of the part, making it less suitable for load-bearing applications.
- Risk Factors: Thin-walled materials, high-strength steels, and parts with extreme geometries are at higher risk of tearing or thinning under excessive pressure.
4.2 Wrinkling and Incomplete Fills
Inadequate pressure can prevent the material from filling the die completely, leading to wrinkling or incomplete fills. Wrinkling can be particularly problematic in applications where aesthetic appearance is important, as it affects surface quality and may lead to functional defects.
- Mitigation: Using incremental pressure increase or adjusting die design can help reduce the likelihood of wrinkling and improve material flow.
4.3 Equipment Wear and Maintenance Costs
High pressures place significant strain on hydroforming equipment, including the hydraulic systems, dies, and clamping mechanisms. This increases maintenance requirements and can lead to costly downtime, especially in high-volume production settings.
- Solutions: Employing a combination of hydraulic and mechanical forces, as seen in hybrid hydroforming, can reduce the pressure demands on equipment, thereby reducing maintenance costs and extending equipment lifespan.
5. Technological Advances in Pressure Control
New developments in pressure control technology are helping manufacturers improve hydroforming precision, efficiency, and reliability. These advancements include adaptive pressure systems, predictive modeling, and pressure sensors, all of which enable greater control over pressure levels during the forming process.
5.1 Adaptive Pressure Control Systems
Modern hydroforming presses are equipped with adaptive control systems that adjust pressure levels in real-time, based on material behavior and process conditions. This technology enables manufacturers to optimize pressure application dynamically, reducing the risk of defects and ensuring high-quality parts.
- Real-Time Monitoring: Pressure sensors and controllers monitor material flow, wall thickness, and strain, allowing for real-time adjustments to pressure levels and improving part consistency.
5.2 Predictive Modeling and Simulation
Computer simulations, including finite element analysis (FEA) and predictive modeling, are essential for determining the ideal pressure for each part. These models allow engineers to test different pressure levels and identify potential defects before production.
- Finite Element Modeling: FEA is used to simulate how materials respond to different pressures, helping manufacturers predict thinning, wrinkling, and other pressure-related defects, thereby improving process efficiency and reducing material waste.
Conclusion
Pressure plays a central role in the success of hydroforming processes, determining everything from shape accuracy and surface quality to structural integrity. The precise application of hydraulic pressure allows for the creation of complex geometries, uniform wall thickness, and aesthetically pleasing finishes, making hydroforming an invaluable technique for industries that demand high-performance metal components.
Understanding how different materials and geometries respond to pressure is essential for selecting the best approach to hydroforming, as incorrect pressure levels can lead to defects such as wrinkling, tearing, or incomplete fills. By leveraging advanced pressure control technologies, including real-time monitoring and computer simulations, manufacturers can optimize the hydroforming process, ensuring consistent quality and reducing production costs. As technology continues to advance, the role of pressure in hydroforming will become even more precise, supporting the creation of increasingly intricate and high-performance components across multiple industries.
Hydroforming is a specialized metal-forming technique that uses hydraulic pressure to shape metals into complex geometries, typically for creating lightweight, high-strength parts. The pressure applied during hydroforming is a critical aspect, as it determines the final part’s shape accuracy, surface quality, and structural integrity. Determining the optimal pressure for a given hydroforming process involves understanding various influencing factors, such as material properties, part geometry, thickness, and hydroforming method.
This article explores the key factors that affect hydroforming pressure, discussing how each variable contributes to the outcome of the hydroforming process and the challenges that arise when balancing these elements.
1. Material Properties
The properties of the material being hydroformed are among the most significant factors affecting the necessary pressure. Different materials have unique strength, hardness, ductility, and formability levels, which influence how they respond to pressure in the hydroforming process.
1.1 Ductility and Formability
Materials with high ductility, such as aluminum and mild steel, are easier to shape under lower pressures than high-strength materials like titanium or advanced high-strength steels (AHSS). Metals with high formability can undergo significant deformation without cracking, reducing the pressure required to achieve the desired shape.
- Ductile Metals: Ductile metals like aluminum can be formed at lower pressures due to their ability to stretch and fill the die without tearing.
- High-Strength Materials: High-strength steels and titanium require higher pressures because they resist deformation, which also increases the risk of cracking or tearing if not handled carefully.
1.2 Strength and Hardness
High-strength materials require higher pressures to achieve desired deformations. Metals with higher tensile strength and hardness are more resistant to pressure, meaning they need more force to be shaped effectively without compromising their integrity.
- Steel Alloys: Alloys like high-strength low-alloy (HSLA) steel, commonly used in the automotive industry, require careful pressure control due to their limited ductility, often necessitating advanced process techniques like warm hydroforming to reduce cracking risks.
2. Part Geometry and Complexity
The shape and geometry of the part being hydroformed significantly affect the required pressure. Components with complex shapes, sharp angles, or deep draws need more pressure to ensure the metal fills all contours of the die without defects.
2.1 Deep Draws and Sharp Angles
Parts with deep draws or sharp angles are more challenging to form, as the metal needs to be pushed into deeper sections of the die. Higher pressures are required to ensure complete fill of the die, avoiding issues like wrinkling or incomplete fills.
- Automotive Frame Rails and Subframes: These components, which often have intricate, multi-dimensional shapes, require high pressures to form accurately, especially in areas with deep contours.
2.2 Thin-Walled Parts
Thin-walled parts are prone to tearing and wrinkling if too much pressure is applied too quickly. These parts require a balance of pressure to ensure the material flows evenly without excessive stretching or thinning.
- Exhaust Systems and Heat Shields: In automotive applications, hydroformed exhaust systems and heat shields need controlled pressures to maintain consistent wall thickness and structural integrity.
2.3 Variable Wall Thickness
Hydroforming allows for variable wall thicknesses in a single part, which optimizes the weight and strength of the component. However, creating parts with varying thicknesses requires precise pressure adjustments to ensure the material flows correctly in each section of the die.
- Bicycle Frames: Parts like bicycle frames, which benefit from variable wall thicknesses, require sophisticated pressure control to ensure that thin areas do not crack while thicker areas are properly formed.
3. Hydroforming Process Type
Different hydroforming methods—such as low-pressure, high-pressure, warm hydroforming, and explosive hydroforming—have unique pressure requirements based on their specific applications and the results they aim to achieve.
3.1 Low-Pressure Hydroforming
Low-pressure hydroforming generally operates at pressures below 5,000 psi and is ideal for creating parts that do not require extreme deformation. This method uses less pressure to gently form parts with minimal deformation and consistent wall thickness.
- Applications: Automotive exhaust systems, furniture frames, and decorative architectural elements.
- Pressure Requirement: Low pressures reduce the risk of tearing or cracking in thin-walled parts and allow for the creation of smooth, rounded contours.
3.2 High-Pressure Hydroforming
High-pressure hydroforming requires pressures above 10,000 psi and is used for parts with complex shapes and tight tolerances. High-pressure hydroforming can produce components with deep draws and sharp radii, making it ideal for parts that need to withstand high structural loads.
- Applications: Automotive chassis components, aerospace structures, and crash-resistant beams.
- Pressure Requirement: High pressures ensure complete die fill for intricate shapes, though they require careful control to avoid material failure in thin or complex areas.
3.3 Warm Hydroforming
In warm hydroforming, moderate pressures are combined with heat, making it easier to shape materials like high-strength steel and titanium. The increased temperature makes these metals more ductile, allowing for more controlled material flow at lower pressures than would otherwise be required.
- Applications: Crash-resistant structures in automotive and aerospace industries.
- Pressure Requirement: The combination of heat and moderate pressure improves formability and reduces the risk of cracks, especially in high-strength materials.
3.4 Explosive Hydroforming
Explosive hydroforming generates extremely high-pressure shockwaves, suitable for large parts or high-strength materials. This method, often used for large aerospace components and energy sector vessels, provides the power needed to shape ultra-high-strength materials into complex, large-scale structures.
- Applications: Aircraft fuselage sections, pressure vessels, and nuclear energy containment systems.
- Pressure Requirement: Explosive force exceeds conventional hydraulic systems, making it ideal for parts with high structural requirements.
4. Die Design and Tooling
The design of the die and tooling used in hydroforming also influence the required pressure. The die must be robust enough to withstand the applied pressure without deforming, while the tooling must accommodate complex geometries and allow for optimal material flow.
4.1 Die Material and Strength
The die material must be chosen based on the pressures required in hydroforming. High-pressure hydroforming requires high-strength die materials to avoid deformation under extreme forces, especially when working with high-strength metals like AHSS or titanium.
- Die Longevity: The die’s strength and durability are critical for maintaining dimensional accuracy in high-pressure hydroforming processes, especially in high-volume applications.
4.2 Die Design for Complex Geometries
For parts with intricate shapes, the die must be designed to facilitate material flow without causing localized thinning or tearing. Complex die designs with deep draws require higher pressures and more sophisticated control systems.
- Material Flow Optimization: Dies designed to support uniform material flow are essential in preventing defects, as poor flow can result in inconsistent thickness or incomplete fills.
5. Wall Thickness and Material Distribution
The thickness of the material being formed directly impacts the pressure required for successful hydroforming. Thicker materials require higher pressures to deform effectively, while thin-walled parts are more susceptible to defects under excessive pressure.
5.1 Controlling Wall Thickness
Maintaining consistent wall thickness is critical in hydroforming, particularly for load-bearing applications in automotive and aerospace sectors. Too much pressure can lead to uneven thinning or material stress, reducing the structural integrity of the part.
- Multi-Stage Forming: For parts requiring controlled thickness variation, multi-stage hydroforming processes with incremental pressure increases are used to achieve optimal results.
5.2 Material Flow in Thin-Walled Parts
Thin-walled parts require careful pressure control to avoid tearing or wrinkling, especially in sections that undergo significant deformation. Reducing the risk of thinning in these areas involves using adaptive pressure systems that can adjust pressure based on material thickness at different stages of the forming process.
6. Technological Advances in Pressure Control
Recent advancements in pressure control technology have improved hydroforming’s accuracy, efficiency, and quality. These innovations include real-time pressure monitoring, adaptive control systems, and predictive modeling, which optimize pressure application and reduce the risk of defects.
6.1 Real-Time Pressure Monitoring
Real-time monitoring systems equipped with pressure sensors enable manufacturers to make adjustments during the hydroforming process, improving consistency and quality.
- Adaptive Control Systems: These systems monitor material behavior and adjust pressure levels dynamically, preventing issues like tearing or wrinkling in thin-walled sections.
6.2 Predictive Modeling and Finite Element Analysis (FEA)
Finite element analysis (FEA) and predictive modeling help engineers determine the best pressure settings for different materials and geometries by simulating the forming process before production.
- Defect Prevention: Simulation tools allow for precise predictions of material flow, thinning, and risk areas, enabling manufacturers to optimize the process setup and reduce material waste.
Conclusion
Hydroforming pressure plays a central role in shaping high-quality metal components, impacting everything from material flow and wall thickness to part accuracy and durability. Various factors—including material properties, part geometry, process type, and tooling design—influence the required pressure, with each factor contributing to the unique demands of a given application.
Advancements in pressure control, predictive modelingtechnologies, such as real-time pressure monitoring, adaptive control systems, and predictive modeling, have made hydroforming more precise, reliable, and efficient. These innovations enable manufacturers to apply the optimal pressure for each part’s unique requirements, thereby improving quality and reducing production costs.
Understanding the diverse factors that influence hydroforming pressure—from material characteristics and part complexity to process type and die design—helps manufacturers fine-tune the process for a range of applications across industries like automotive, aerospace, medical devices, and construction. With the continued evolution of hydroforming technology, these pressure-dependent forming processes are likely to expand their applicability, allowing for even more complex, high-performance components to be manufactured with efficiency and precision.
EMS Metalworking Machinery
We design, manufacture and assembly metalworking machinery such as:
- Hydraulic transfer press
- Glass mosaic press
- Hydraulic deep drawing press
- Casting press
- Hydraulic cold forming press
- Hydroforming press
- Composite press
- Silicone rubber moulding press
- Brake pad press
- Melamine press
- SMC & BMC Press
- Labrotaroy press
- Edge cutting trimming machine
- Edge curling machine
- Trimming beading machine
- Trimming joggling machine
- Cookware production line
- Pipe bending machine
- Profile bending machine
- Bandsaw for metal
- Cylindrical welding machine
- Horizontal pres and cookware
- Kitchenware, hotelware
- Bakeware and cuttlery production machinery
as a complete line as well as an individual machine such as:
- Edge cutting trimming beading machines
- Polishing and grinding machines for pot and pans
- Hydraulic drawing presses
- Circle blanking machines
- Riveting machine
- Hole punching machines
- Press feeding machine
You can check our machinery at work at: EMS Metalworking Machinery – YouTube
Applications:
Flange-punching
Beading and ribbing
Flanging
Trimming
Curling
Lock-seaming
Ribbing